Blood products are administered as therapeutic agents in perioperative management. Despite guidelines, most physicians either have not read the guidelines or have difficulty following them. Clinicians often resort to empiric therapy because laboratory tests are difficult to obtain, take too long, do not determine platelet defects, or because they have concerns about later getting blood products from the blood bank in a timely fashion for high-risk patients. Of note is that transfusions are important for massive bleeding following trauma and transfusion under these conditions will be considered in a separate chapter. In a critically ill patient, the importance of a multimodality approach is important rather than focusing on the individual transfused components.
The American Society of Anesthesiologists established the Task Force on Blood Component Therapy to develop evidence-based guidelines for transfusing RBCs, platelets, FFP, and cryoprecipitate in perioperative settings. Specific guidelines were developed according to a defined methodology.5 The recommendations of the task force were reported and can be found at http://www.asahq.org/publicationsAndServices/transfusion.pdf (Table 28-2). Although these guidelines are reported as recommendations, there are also other important considerations regarding the use of specific blood units in surgical patients that need to be considered. The rationale for transfusion of individual blood components will also be considered.

Transfusion Therapy for Bleeding
Volume replacement in the perioperative setting when there is critical bleeding requires the use of replacement therapy. Although crystalloid, colloid, and RBCs are used initially, none provide coagulation factors or platelets and thus their use can exacerbate coagulopathy. Severe bleeding requires use of FFP, platelets, cryoprecipitate, and factor concentrates (e.g., fibrinogen and prothrombin complex concentrates) to restore circulating levels of hemostatic factors. Following massive transfusion therapy, hypothermia and acidosis frequently occur, further complicating bleeding. Thus, temperature and pH must be monitored and corrected during any ongoing transfusion effort.
Red Blood Cells
There is no single minimum acceptable hemoglobin level that can be applied to all patients when deciding when to transfuse RBCs. Chronic anemia is better tolerated than acute anemia. However, with acute anemia, compensatory mechanisms that increase cardiac output and improve oxygen transport depend on the patient’s cardiovascular reserve. In surgical patients with heart failure and/or flow-restricting lesions, compensation during acute anemia may be limited. Multiple factors should be considered including intravascular volume, whether the patient is actively bleeding, and the need for improvement in oxygen transport. For instance, the patient receiving multiple inotropes and requiring an intraaortic balloon pump who is anemic following surgery may need RBC transfusion at a higher hemoglobin level than an otherwise healthy and hemodynamically stable patient. The decision to transfuse must weigh the risks of transfusion against the need for improved oxygen-carrying capacity in recovery from trauma, surgery, or illness.5 The American Society of Anesthesiologists Task Force on Perioperative Blood Transfusion noted in its recommendations that transfusion of RBCs should usually be administered when the hemoglobin concentration is low (e.g., <6 g/dL in a young, healthy patient), especially when the anemia is acute. RBCs are usually unnecessary when the hemoglobin concentration is more than 10 g/dL. These conclusions may be altered in the presence of anticipated blood loss or active critical (i.e., myocardium, central nervous system or renal) or target organ ischemia. Determining whether intermediate hemoglobin concentrations (i.e., 6 to 10 g/dL) justify or require RBC transfusion should be based on any ongoing indication of organ ischemia, potential or actual ongoing bleeding (rate and magnitude), the patient’s intravascular volume status, and the patient’s risk factors for complications of inadequate oxygenation. These risk factors include a low cardiopulmonary reserve and high oxygen consumption.”5 Hemoglobin triggers for transfusion are not to be taken as absolute; patients with significant cardiac disease should be transfused if signs or symptoms of inadequate myocardial oxygenation appear.
One important aspect of adverse events associated with RBC transfusion relates to the age of RBCs transfused and changes that occur in stored RBCs as they age (the so-called storage lesion).6–8 Although RBCs are stored for up to 42 days after donation, biochemical changes occur in RBCs as they age. Studies suggest that RBC units stored for long periods (often described as >14 to 21 days) may lead to adverse effects. The mechanisms for these adverse events include senescent RBC fragments, impaired nitric oxide production, and increased nitric oxide scavenging by stored RBCs, together with reduced nitric oxide synthesis by dysfunctional endothelial cells. However, many of the studies demonstrating adverse outcomes are from large retrospective studies with all of the inherent problems associated with retrospective analyses and not all studies are in agreement.
Red Blood Cell Storage Lesions
RBCs develop a complex series of biochemical and metabolic changes during storage in the blood bank that include depletion of adenosine triphosphate (ATP) and of 2,3-diphosphoglycerate (2,3-DPG); membrane phospholipid vesiculation and shedding, protein oxidation, and lipid peroxidation of the cell membrane. As the blood ages, RBCs undergo shape changes with increased fragility that may impair microcirculatory flow. Because of increased red cell–endothelial cell interaction, bioreactive lipids and other substances are released that may initiate inflammatory responses leading to TRALI, as described in more detail in the following text. These complex changes can also decrease oxygen delivery and increase hemolysis. When free hemoglobin is released from the RBC, it binds to nitric oxide and this nitric oxide scavenging causes endothelial dysfunction and additional proinflammatory events including generation of oxygen free radicals.
Red Blood Cell Storage and Tissue Oxygenation Parameters
Transfusion of RBCs is used therapeutically to increase the oxygen-carrying capacity of blood and thereby improve oxygen delivery to tissues. Multiple retrospective studies have attempted to evaluate the clinical outcomes of critically ill patients receiving fresh compared to older blood. There are multiple analysis in the literature that examine the clinical effect of blood storage in multiple patient groups including trauma patients, ICU patients, and patients undergoing cardiac surgery or those with acute heart disease with variable effects. Most of the studies are observational based and there is clinical equipoise regarding results. Currently, a randomized study is underway to address this issue as will be described later. As they also note, previous systematic reviews and a meta-analysis conducted in critically ill patients have been inconclusive.
Current studies underway include the Red Cell Storage Duration Study (RECESS: NCT00991341), a prospective, randomized clinical trial (RCT). The National Heart, Lung and Blood Institute (NHLBI)–funded RECESS is a multicenter RCT. Complex cardiac surgical procedures are being randomized to receive RBC units stored for 10 days or fewer versus RBCs stored for at least 21 days and will include approximately 1,434 patients. Outcomes being evaluated include multiple organ dysfunction score, all-cause mortality, and other measures of organ dysfunction will be evaluated. Other clinical studies include The Canadian Age of Blood Evaluation (ABLE) study (trial of the resuscitation of critically ill patients: ISRCTN44878718) is currently being conducted in ICU patients. It will enroll a total of 2,510 patients in Canada, France, and the United Kingdom and will compare 90-day mortality between patients transfused with fresh RBC (storage <8 days) compared to current practices. The TRANSFUSE trial (ACTRN12612000453886) started in 2012 in Australia and New Zealand is a large (5,000 patients) pivotal, multicenter, randomized, controlled trial in critically ill patients to determine whether, compared with standard care, transfusion of the freshest available RBC decreases patient mortality. Completion is expected in 2015.
The true impact of the age of stored RBC on patient outcomes remains in question but an answer should emerge from these ongoing clinical trials. Although RBC transfusions are used extensively in the perioperative setting, all transfusions can be associated with adverse events. Multiple factors are responsible for the storage lesion that is specific to RBCs, and current studies will further resolve the questions.
Plasma/Fresh Frozen Plasma
Plasma is transfused for multiple indications, especially in surgical and trauma patients. Plasma use has increased with the increasing understanding of its role in managing the coagulopathy associated with massive transfusion (massive transfusion is covered in detail in a separate chapter). Currently, plasma and FFP are used to replace volume and coagulation factors during massive transfusion, to treat or prevent future bleeding during surgery and invasive procedures, to reverse warfarin therapy in patients, and for treatment of coagulation factor abnormalities where specific concentrates are not available. FFP is plasma frozen within 8 hours of collection. However, many plasma units transfused in the United States are actually frozen within 24 hours after phlebotomy (FP24). The difference is that cryoprecipitate can be obtained from FFP but not FP24; nonetheless, experts agree that FFP and FP24 can be transfused interchangeably and most clinicians refer to both products as FFP, despite this subtle distinction. Thawed plasma (either FFP of FP24) stored for up to 5 days before administration is also commonly used for transfusion. Plasma is used throughout the text to refer to FFP, FP24, or thawed plasma, as most scientific evidence supporting and distinctions among different plasma transfusion practices is limited.
Following collection of a unit of blood, FFP is the plasma that remains after RBC and platelet removal and contains blood coagulation factors, fibrinogen, and other plasma proteins in a volume of 170 to 250 mL that is then frozen and can be stored for up to 1 year. Most plasma administered in perioperative settings is actually FP24. Before administration, the plasma must be thawed in a water bath at 37°C, which takes about 30 minutes. After thawing, the units of FFP are stored at 1°C to 6°C and are transfused within 24 hours. FFP should be administered through a component administration set with a 170-micron filter. If not used within 24 hours, it can be relabeled as “thawed plasma” and stored at 1°C to 6°C for an additional 4 days. Thawed plasma maintains normal levels of all factors except factor V which falls to 80% of normal and factor VIII which falls to 60% of normal during storage.9 Because these levels are above the in vivo threshold for normal hemostatic function for these factors and factor VIII is an acute phase reactant, thawed plasma can be used as a substitute for FFP.9
FFP is used for treating bleeding because of coagulopathies that are associated with a prolongation of either the activated partial thromboplastin time (aPTT) or prothrombin time (PT)/international normalized ratio (INR) greater than 1.5 times normal, or a specific coagulation factor assay of less than 25%.5 FFP is often used to reverse the effect of warfarin before surgery or during active bleeding episodes (see prothrombin complex concentrates later in this chapter and chapter 29). When FFP is indicated, it should be administered in a dose calculated to achieve a minimum of 30% of plasma factor concentration. Ten to 15 mL/kg of FFP will generally result in a rise of most coagulation proteins by 25% to 30% (or increases in 0.25 to 0.3 U/mL), although a dose of 5 to 8 mL/kg may be adequate to urgently reverse warfarin anticoagulation but varies based on the initial levels of the vitamin K–dependent coagulation factors.5 FFP is also an important part of a transfusion algorithm for posttraumatic bleeding that is covered in more detail in the chapter on massive transfusion.
Guidelines exist in many countries for the use of plasma and include active bleeding preoperatively, invasive procedures in patients with acquired coagulation abnormalities, immediate correction of vitamin K antagonists (e.g., warfarin), thrombotic thrombocytopenic purpura, and patients with a congenital coagulation factor deficiency where specific factor concentrates are not available. Published plasma transfusion indications are listed in Table 28-3.

Plasma is overused in surgery, most often because of the empirical nature of transfusion therapy. The most common cause of bleeding after surgery is platelet dysfunction. Further, the PT and partial thromboplastin times (PTT), which are widely used to evaluate bleeding, have never been demonstrated to accurately reflect the cause of bleeding in surgical patients. Indeed, the PT and PTT can be abnormal in patients who are not bleeding. Despite the widespread use of plasma, there is little evidence for its effectiveness outside of trauma patients requiring massive transfusion.10,11 Analyses of randomized controlled trials have been unable to demonstrate consistent evidence of benefit for plasma in most clinical scenarios.10–12 The use of plasma in many situations to treat elevated INRs, especially when the INR is less than 1.7 is problematic, as these patients may not be at risk of bleeding and the lowest INR obtainable with plasma is approximately 1.5 because that is the INR of plasma/FFP.13 A recent survey evaluating approximately 5,000 plasma transfusions reported that 43% were administered in the absence of bleeding in efforts to correct abnormal coagulation tests preoperatively or before invasive procedures, and in 31% of cases where plasma was given the INR was 1.5 or less.14
Plasma transfusions, like all blood products, have the potential for adverse effects. A recent study reported a 6% incidence of transfusion-associated circulatory overload (TACO) in ICU patients, which can occur when patients with heart failure/ventricular dysfunction are given as little as 2 to 4 units of plasma.15 TRALI is a major cause of mortality and morbidity from blood transfusion, although its incidence has declined with the use of plasma from male donors or female donors who have no history of pregnancy.16,17 TRALI and TACO will be considered in more detail later.
Solvent/Detergent–Treated Plasma
Human pooled plasma that has been solvent/detergent (S/D) treated is now available commercially in a sterile, frozen solution of pooled human plasma from donors that has been treated with an S/D process. This method of preparation kills certain viruses and minimizes the risk of serious virus transmission but also removes other agents, including cellular debris and lipid contaminants. This process is thought to reduce the risk of TRALI. The plasma used to manufacture this product is collected from specific pools of U.S. donors who have been screened and tested for diseases transmitted by blood, and determined to be suitable donors. Collected donor pools include approximately 500 to 1,600 donors. This product is used extensively in Europe and other countries and approximately 13 million have been administered outside of the United States. In the United States, this product is indicated for replacement of multiple coagulation factors in patients with acquired deficiencies due to liver disease, those undergoing cardiac surgery and liver transplantation, and for plasma exchange in patients with thrombotic thrombocytopenic purpura. Administration is based on ABO blood group compatibility.
The collection and testing process for this pooled and treated plasma is extensive; unlike other blood products, it is extensively purified and tested. The product is manufactured from U.S. plasma donations that are extensively tested for viral markers with each pool limited to 630 to 1,520 individual donors. Frozen plasma units are thawed, pooled, filtered through a 1-µm pore membrane, then treated with S/D reagents (1% tri[n-butyl] phosphate [TNBP] and 1% octoxynol for 1 to 1.5 hours at +30°C [86°F]) to inactivate enveloped viruses. The S/D reagents are removed by sequential oil and solid phase extraction procedures that also remove prions. After sterile filtration, the product is filled into blood bags, labeled, deep-frozen, and stored at 4°F. The S/D treatment step has been shown to effectively inactivate relevant pathogenic and enveloped viruses.
Leukocyte antibodies are not detected in S/D plasma because the process dilutes white blood cell antibodies and soluble human leukocyte antigens (HLAs) are present in the product, neutralizing the antibodies. This pooled and treated plasma product has not been associated with TRALI, with more than 13 million units transfused to date.
Cryoprecipitate
In the early 1960s, attempts to create an improved factor VIII concentrate led to the development of cryoprecipitate. Cryoprecipitate forms when frozen plasma is allowed to thaw slowly at 1°C to 10°C; cryoprecipitate is rich in fibrinogen, factor VIII, and factor XIII but also contains other factors. This product was introduced as a therapy for patients with hemophilia A18,19; however, its major use today is to replete fibrinogen levels during coagulopathies. Cryoprecipitate contains fibrinogen and high concentrations of factor VIII, von Willebrand factor, and factor XIII.
Cryoprecipitate is composed of the insoluble proteins that precipitate when FFP is thawed and is named for that process. The residual volume of cryoprecipitate (~15 mL) is refrozen and stored. Cryoprecipitate contains therapeutic amounts of factor VIII:C, factor XIII, von Willebrand factor, and fibrinogen. Each bag of cryoprecipitate contains 80 to 100 units of factor VIII:C, 150 to 200 mg of fibrinogen, significant amounts of factor XIII, and von Willebrand factor, including the high-molecular-weight multimers. Cryoprecipitate is used not only to increase fibrinogen levels depleted because of massive hemorrhage or coagulopathy but also for the treatment of congenital or acquired factor XIII deficiency. For fibrinogen replacement therapy, in Europe, specific fibrinogen concentrates are available (see the following text); however, 1 unit of cryoprecipitate per 10 kg body weight increases plasma fibrinogen by roughly 50 to 70 mg/dL in the absence of continuing consumption or massive bleeding.20 The minimum hemostatic level of fibrinogen is traditionally suggested to be around 100 mg/dL but normal fibrinogen levels are 200 mg/dL and higher, and higher levels of fibrinogen may be important for clot formation (see “Fibrinogen Concentrates” section). Because cryoprecipitate does not contain factor V, it should not be the sole replacement therapy for disseminated intravascular coagulopathy (DIC), which is almost always associated with a variety of factor deficiencies and thrombocytopenia. Because fibrinogen is an important determinant of hemostatic function and clot strength, fibrinogen levels should be routinely evaluated in bleeding patients especially following multiple transfusions. Hypofibrinogenemia itself can cause a prolonged PT and PTT, and FFP transfusion alone may not provide sufficient repletion. Cryoprecipitate is likely underused in cardiac surgical patients who are bleeding and “refractory” to standard FFP and platelets.
Cryoprecipitate has been withdrawn from many European countries due to safety concerns, primarily the transmission of pathogens. Instead, commercial fibrinogen preparations are available for fibrinogen replacement therapy. The fibrinogen concentrates used for repleting fibrinogen levels are free of known pathogens, stored as a lyophilized product, and can be readily administered when required. Nevertheless, cryoprecipitate remains available for hemostatic therapy in several countries, including the United States, Canada, and the United Kingdom. An adult dose of cryoprecipitate is ~10 units obtained from 10 different donors, and is equivalent to ~2 g fibrinogen.
Platelet Concentrates
Platelets that are used clinically are either pooled random-donor platelet concentrates or single-donor apheresis and can be stored for up to 5 days. In medical patients, a platelet count of 10,000/µL is a typical threshold for prophylactic platelet transfusion (normal platelet count ranges from 150,000 to 400,000 platelets per µL), but the optimal platelet count or dose is still being evaluated. Consensus descriptions suggest the platelet count for therapeutic transfusions to control or prevent bleeding with trauma or surgical procedures requires a higher transfusion trigger of 100,000/µL for neurosurgical procedures and between 50,000/µL and 100,000/µL for other invasive procedures or trauma. Many transfused products, including platelets, undergo leukoreduction to reduce alloimmunization rates, cytomegalovirus (CMV) transmission, and febrile transfusion reactions. Whether leukoreduction reduces immunomodulatory effects of transfusion (i.e., decreases infection rates and cancer recurrence) is still controversial, as is the use of universal leukoreduction as the procedure, is associated with significant cost.
Platelet concentrates can be prepared either from whole blood or by apheresis. For many years, the use of platelet concentrates was the standard for platelet administration, and this required exposure to multiple donors, as 10 units of platelets required 10 different donors. An important advantage of platelets collected by apheresis is that a sufficient enough number can be collected from a single donor while an equivalent number of platelets require pooling of at least 4 to 6 whole blood–derived platelets concentrates. Reducing donor exposures by using apheresis platelets also has the potential advantages of reducing transfusion-transmitted infections and platelet alloimmunization where antibodies form because platelets have many antigens besides ABO.21,22 Testing has reduced the infectious risk to low levels. The quality of apheresis platelets is similar to pooled random-donor platelets concentrates, these two products can be used interchangeably based on availability and cost considerations.21,22
There remains significant risk of bacterial infection with platelet administration, because they are stored at 22°C rather than at the 4°C storage used for red cell storage; storage at 22°C is permissive for bacterial growth. Some studies have suggested a reduction in bacterial transmission by transfusion with the use of single-donor platelets.21,22 However, both the American College of Pathologists and the American Association of Blood Banks (AABB) have mandated testing of all platelet products for bacteria.21,22
Alloimmunization
Allogeneic blood transfusions are in many ways similar to organ transplantation. Transfusing cells from one patient (the donor) to the recipient introduces multiple foreign cells, antigens, and other potential contaminants. An immunocompetent recipient often develops variable immune responses to the transfused agents that include graft versus host disease (to be considered later in this chapter). Multiple other antigens that are not routinely crossmatched for platelets and responsible for alloimmunization include HLAs, class I shared by platelets and leukocytes and class II present on some leukocytes; granulocyte-specific antigens; platelet-specific antigens (human platelet antigen); and RBC-specific antigens. Platelets are cross matched only to RBC specific antigens. The spectrum of additional antigenic components in platelets is why leukoreduction is part of an important management strategy.
Leukoreduction
Leukoreduced platelet and RBC products have many potential benefits. By reducing additional leukocyte exposure, sensitization and antibody formation to different white blood cell antigens (alloimmunization) is reduced.21,22 CMV transmission is also reduced by reducing leukocyte burden, and as a result, there is also a reduction in febrile transfusion reactions.21,22 Other potential benefits of leukoreduction include decreased exposure to white cells that potentially contribute to immunomodulatory effects of transfusion (transfusion-related immunomodulation or TRIM) that may present as increased risk for postoperative infections and tumor metastasis formation in cancer surgery. There is extensive controversy about the immunomodulatory effects of transfusions as patients often have multiple other risk factors that contribute to outcomes.21,22 Several countries including Canada and many medical centers have instituted universal leukoreduction of the blood supply.
Graft versus Host Disease
In cancer patients and certain pediatric populations, platelets are irradiated to prevent transfusion-related graft versus host disease, a potentially fatal complication of transfusion. Graft versus host disease occurs more commonly after a bone marrow or stem cell transplant, or following platelet transfusions where viable white cells from the donor regard the recipient’s body as foreign and create acute inflammatory responses and tissue and organ injury by attacking the recipient’s body. For platelet transfusions, γ-irradiation is performed for patients receiving allogeneic stem cell transplants, for patients receiving blood products from related donors, and for patients who are severely immunocompromised, usually because of their disease or its treatment (e.g., patients with Hodgkin’s disease or other lymphomas).21,22
Indications for Platelet Transfusions and Transfusion Triggers
In medical patients, a platelet transfusion trigger of approximately 10,000 platelets/µL in efforts to prevent bleeding is often described. However, data and prospective studies to evaluate the effects of platelet dose on hemostasis and rates of platelet use overall for perioperative management are often based on consensus guidelines rather than clinical studies. There are three important areas of controversy regarding the use of platelet transfusions without active bleeding23: first, the optimal prophylactic platelet dose to prevent thrombocytopenic bleeding even in medical patients is not well known. Second, the exact platelet count threshold that requires transfusion of platelets is not known. Finally, whether prophylactic platelet transfusions are superior to therapeutic platelet transfusions in surgical patients is not known.
A review of clinical trials24 suggests that in hematologic malignancies, a target platelet count of more than 10,000 platelets/µL is acceptable in preventing spontaneous bleeding caused by thrombocytopenia alone,25 although platelet dosing was not found to influence bleeding when administered prophylactically.26 Guidelines for platelet transfusions exist in many countries.27–32 Hematology patients receive about two-thirds of all platelet concentrates, depending on the medical center. Additional studies are needed to determine the optimal transfusion practice; however, the clinical use of platelet transfusions to obtain hemostasis is complicated because direct platelet function testing is rarely possible in the bleeding or coagulopathic patient. For instance, after cardiopulmonary bypass, platelet counts may be normal, but platelets are functioning poorly (qualitative platelet defect). Most recommendations are to maintain platelet counts of greater than 50,000/µL in surgical patients; however, this is also dependent on whether the circulating platelets are functional. While definitive data for the most effective platelet dosing strategy for maintaining perioperative hemostasis is not available, following platelet numbers is our only practical guide. However, clinicians must bear in mind that patients with abnormal platelet counts and/or hemostasis may not bleed at the same time that patients with normal platelets counts may bleed based on the platelet dysfunction that appears in many surgical settings.
In most surgical patients, there is little data to support prophylactic platelet transfusions; the exceptions are massive transfusion coagulopathy and certain closed procedures where bleeding may be highly problematic such as intracranial hemorrhage. Dilutional thrombocytopenia often occurs as an early manifestation of massive transfusion. However, studies also suggest thrombocytopenia may not always correlate with abnormal bleeding. In cardiac surgical patients, defective platelet function is part of the clinical problem, and the inability to have suitable platelet function testing for postoperative use complicates our ability to decide when to transfuse platelets.21,22
Studies in chronic thrombocytopenic patients suggested that significant spontaneous bleeding with an intact vascular system does not occur until the platelet count is 5,000 platelets/µL or less.21,22 Previously, a platelet count of less than or equal to 20,000/µL was considered to be an indication for a prophylactic platelet transfusion. However, four randomized prospective transfusion trials comparing prophylactic platelet transfusion triggers of 10,000 platelets/µL versus 20,000 platelets/µL showed no differences in hemorrhagic risks.33 Current recommendations are that a threshold of 10,000 platelets/µL for prophylactic platelet transfusion be used in hematology patients who are chronically thrombocytopenic.
Platelet Counts for Surgery and Invasive Procedures
For surgery or following trauma, expert recommendations suggest that a platelet count of greater than or equal to 50,000/µL be maintained. However, there is little data to support these recommendations. In neurosurgical patients or patients with intracerebral bleeding and for neurosurgical procedures, expert recommendations suggest that platelet counts should be maintained at greater than 100,000/µL. With platelet counts between 50,000 and 100,000/µL, clinical decisions to transfuse platelets should be based on the type of surgery, trauma, rates of bleeding, risk of bleeding, use of platelet inhibitors, and other potential coagulation abnormalities. An assessment of whether platelet function is normal should also weigh in to the decision about when to transfuse platelets.
Abnormal platelet function can arise from numerous causes, including multiple medications, sepsis, malignancy, tissue injury following trauma, obstetric issues including eclampsia, cardiopulmonary bypass, or hepatic or renal failure with azotemia/uremia. In the bleeding patient, laboratory testing can determine platelet counts but not platelet function, so bleeding due to tissue injury may occur at higher platelet counts. If platelet dysfunction is present in the face of trauma or surgery, platelet transfusions may be necessary, even in the presence of a normal platelet count. Unfortunately, there is little data to help clinicians manage these complex but common occurrences, and as a result, platelet transfusions must be guided by a logical approach that weighs each of these factors.
ABO Compatibility
Red cell antigens are expressed on platelets, and ABO-incompatible platelets have reduced posttransfusion platelet count recoveries but normal platelet survival.21,22 ABO-compatible means the donor has no A or B antigens incompatible with the recipient’s A or B antibodies.21,22 Patients who receive ABO-incompatible platelets become refractory to additional platelet transfusions at a higher rate than the ABO-compatible recipients because sensitization produces anti-HLA and platelet-specific alloantibodies.21,22 ABO-compatible platelets are important both to maximize posttransfusion platelet counts and to reduce the incidence of sensitization, which decreases platelet counts, shortens the half-life, and increases the potential for platelet dysfunction.21,22
Purified Protein Concentrates
Fibrinogen Concentrates
Fibrinogen is a critical clotting protein, with increasing data further reporting its importance for perioperative hemostasis.34 Cryoprecipitate is routinely administered as the source of fibrinogen in many countries, while fibrinogen concentrates are also used in some countries. Although any biologic agent can potentially produce an adverse event, current reviews of published clinical data and pharmacovigilance reporting have not demonstrated significant thrombogenic concerns with fibrinogen concentrate to date.35,36 However, fibrinogen concentrate administration in patients with hypofibrinogenemia and disseminated intravascular coagulation should be avoided and the focus placed on treatment of the underlying disease (i.e., sepsis).37
The advantage of factor concentrates is that the risk of viral infection is significantly reduced due to viral inactivation and removal that minimize the risk of transmitting viruses. Although fibrinogen concentrate is manufactured using human plasma from a large pool of donors, the production processes involved remove antibodies and antigens, largely mitigating the risk of immunologic and allergic reactions resulting from its administration, and provide a pure product without other cellular and protein contaminants.
Prothrombin Complex Concentrates
While warfarin reversal in the United States occurs with FFP,38 most other countries use prothrombin complex concentrates (PCCs).39 PCCs are recommended in guidelines as primary treatment for reversal in patients with life-threatening bleeding and an increased INR when urgent reversal is required. For reversal of warfarin and vitamin K antagonists, several therapies are used and include oral/intravenous vitamin K, human FFP, and PCCs. Although recombinant active factor VII (rFVIIa) has been used, better alternatives are currently available. Although vitamin K can be considered for nonemergency reversal, if rapid normalization of the INR is required, then PCCs should be used. Vitamin K is an active cofactor necessary to convert coagulation factor proteins to their active forms after they are synthesized in the liver. Because of the need for immediate reversal in the perioperative setting, FFP or PCCs should be considered.
Plasma/FFP is the agent most likely to be used in the United States, but for emergent reversal, clinicians should consider PCCs. Agents approved specifically for warfarin reversal include the 4 component PCC available in the US KCENTRA/Beriplex P/N (CSL Behring, King of Prussia, PA), Octaplex (Octapharma, Lachen,Switzerland) approved elsewhere, and there are also other 4 component PCCs available worldwide. Extensive clinical experience with the new purified PCCs available suggests the incidence of thrombotic complications is relatively low; however, they should be avoided in patients requiring surgery who were previously anticoagulated for a prothrombotic reason.
U.S. guidelines recommend PCCs as primary treatment for anticoagulation reversal in patients with life-threatening bleeding and elevated INR and are labeled as such. Clinical protocols for administering PCCS should be accompanied by discontinuing warfarin and its derivatives, and administering vitamin K. Many clinicians continue to use plasma/FFP to reverse an elevated INR even for urgent and emergency procedures, and use of plasma is one of the major causes of TRALI in the United States. The rapid onset of PCCs is important for emergency reversal of vitamin K antagonists including warfarin. Compared with FFP, PCCs provide quicker INR correction, have a lower infusion volume, and are more readily available without cross matching.39,40 Although there are historical concerns about potential thrombotic risk with PCCs, present-day PCCs are much improved.41
Von Willebrand Factor
Human antihemophilic factor/von Willebrand factor complex is commercially available in the United States and is indicated for treatment and prevention of bleeding in adult patients with hemophilia A (classical hemophilia). This agent is also indicated in adult and pediatric patients with von Willebrand’s disease (VWD) for (a) treatment of spontaneous and trauma-induced bleeding episodes and (b) prevention of excessive bleeding during and after surgery. This applies to patients with severe VWD and patients with mild and moderate VWD for whom use of desmopressin is known or suspected to be inadequate. This agent is not indicated for the prophylaxis of spontaneous bleeding episodes.
This product is the first plasma-derived von Willebrand factor/factor VIII (FVIII)–containing concentrate that was pasteurized to reduce the risk of virus infection and approved for use in Germany in 1981 and it is now available in many countries worldwide for on-demand treatment and long-term prophylaxis in patients with VWD or hemophilia A (factor VIII deficiency). This agent is used off label for bleeding with acquired VWD due to ventricular assist devices or aortic stenosis.
Hemophilia Management: Factors VIII and IX
Before the development of factor concentrates, FFP was the treatment for hemophilia A and hemophilia B. However, FFP contains minimal amounts of factor VIII and factor IX, thus large volumes are required to stop bleeding episodes, and this mandated hospitalization for treatment of joint bleeding. In the mid-1960s, the development of cryoprecipitate with concentrated factor VIII preparations allowed a concentrated form of factor VIII to be administered intravenously. By the late 1960s, methods for isolating both factor VIII and factor IX from pooled plasma allowed the development of lyophilized factor VIII or factor IX concentrates, which provided for more accurate dosing using true concentrates that could rapidly restore factor levels in hemophilia, and this also led to the ability to effectively treat patients at home. However, isolating factor concentrates requires combining plasma from multiple donors, and the associated risks of transmission of hepatitis viruses and HIV. Multiple steps were subsequently used to reduce viral contamination using heat, S/D treatment, and pasteurization, but not before many patients were infected. Better screening methods for blood donors improved the safety of donated plasma and included hepatitis B and HIV, and later hepatitis C virus. Recombinant human factor VIII (R factor VIII) was developed following cloning of the gene, and by 1992, two licensed factor VIII products for use in hemophilia A were available, and a factor IX product became available for people with hemophilia B in 1997. Other important developments for hemophilia patients include treatment for patients with inhibitors to factor VIII or factor IX and prophylaxis. Inhibitor antibodies develop in approximately 30% to 35% of people with hemophilia A and 1% to 3% with hemophilia B. A novel therapies for “bypassing” the inhibitor, recombinant activated factor VII (R factor VIIa), was first licensed for use in hemophilia in 1997.
Hereditary Angioedema and C1 Esterase Inhibitor Concentrates
Hereditary angioedema (HAE) is a life-threatening disease resulting from the absence or genetic mutation of a complement component inhibitor called C1 esterase inhibitor (C1 INH). Although there are several variants of HAE, they share a final common pathway following tissue injury, intubation, or other inciting events, leading to unopposed activation of multiple inflammatory pathways and mediators including kallikrein and bradykinin that increase vascular permeability.42 Angioedema produces increased permeability of submucosal or subcutaneous capillaries and postcapillary venules leading to plasma extravasation and subsequent swelling of critical airway structures and other systemic effects. C1 INH concentrates have been used since 1974 in Europe and now are available in the United States for both preventing and terminating attacks. Two of these have now been licensed in the United States for use in HAE patients, one for prophylaxis (Cinryze [ViroPharma, Lexington, MA]) and the other for treating acute abdominal and facial HAE attacks (Berinert-P [CSL Behring, King of Prussia, PA]). The use of C1 INH concentrates is critical in the perioperative management of HAE patients.42
Adverse Effects of Transfusions
The risks of allogeneic transfusion extend beyond viral transmission and include allergy, alloimmunization, anaphylaxis, bacterial sepsis, graft versus host disease, TRALI, TACO, renal failure, volume overload, and immunosuppression.43–45 Platelet transfusions also carry the added risk of bacterial contamination and they contain a high concentration of donor white blood cells. Transfusion of donor white blood cells has the potential to produce multiple adverse effects. Cytokines, such as interleukins 6 (IL-6), 8 (IL-8), tissue necrosis factor alpha (TNF-α), and other inflammatory mediators are especially concentrated in platelet products and could contribute to adverse outcomes.
Transfusion as an Inflammatory Response
Transfusions of allogeneic blood is reported to have multiple immunomodulatory effects including immunosuppression; they contain bioactive substances that cause febrile reactions, and they release inflammatory mediators.46 Inflammatory responses to cardiac surgery are affected by giving packed RBCs during surgery as displayed by neutrophil activation.47 Neutrophils in allogeneic cellular blood components are associated with adverse effects in the recipient.48 In cardiac surgical patients who are already immunosuppressed by surgical trauma, added inhibition of immunomodulation may have harmful effects.
Because of increasing awareness and identification of TRALI and decreases in the incidence of infectious and hemolytic complications of transfusions, TRALI is now a primary cause of transfusion-associated mortality reported to the FDA and has become a frequent cause of transfusion-related morbidity and mortality.49 TRALI can be confused with other transfusion and non–transfusion-related events such as anaphylaxis, hemolysis, circulatory overload and cardiac failure and present with acute shock, florid pulmonary edema and pulmonary hypertension.48,50 Because TRALI is a major cause of mortality resulting from transfusion, strategies have evolved to reduce its incidence and will be considered in more detail later.
Transfusion-Associated Circulatory Overload
TACO is simply a volume overload state, where the rate of volume infusion of blood products is in excess of what the patient’s cardiovascular status can handle. An example is the transfusion of 4 units of FFP to reverse warfarin in a patient with heart failure; in such a patient who can barely manage their own intravascular volume, they may well go on to develop orthopnea and paroxysmal nocturnal dyspnea following transfusion. TACO is characterized by the acute onset of dyspnea and is typically associated with hypertension, tachypnea, and tachycardia—an exacerbation of heart failure as shown in Table 28-4. Sometimes it can be difficult clinically to differentiate TRALI from TACO, but use of echocardiography, transthoracic or transesophageal, will reveal hypervolemia, ventricular dysfunction, and potentially reveal exacerbation of valvular dysfunction. In a TRALI patient, volume overload is not the cause of pulmonary edema, rather left ventricular size should be normal or low, and often the right ventricle is dilated. Alternately, attesting for brain-type natriuretic peptide (BNP) is another approach and this should be greatly elevated, usually several fold above a baseline of 100 to 200 in patients with TACO. TACO incidence ranges from approximately 1% to 8% of transfusions. TACO is increasingly report as a common cause of morbidity and mortality associated with transfusion, especially with the use of FFP.


Full access? Get Clinical Tree
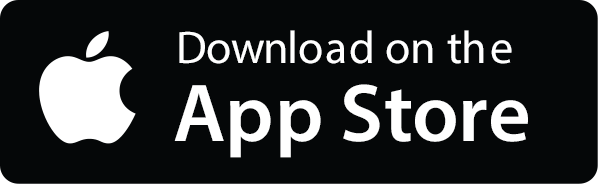
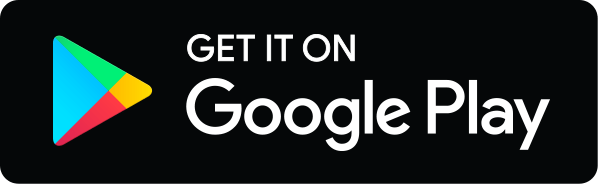