Blood Conservation and Transfusion
KEY POINTS
1 In hemodynamically stable, nonbleeding patients, reasonable target levels for hemoglobin are 7 to 9 gm/dL and greater than 20,000/mm3 for platelets. Higher levels may be appropriate in patients actively bleeding or those at particularly high risk from anemia or hemorrhage.
2 Many transfusions can be avoided if careful thought is given to blood conservation measures, including reevaluating transfusion triggers, limiting laboratory determinations to only those necessary, minimizing the volume of blood for each test, and thoughtfully using erythropoeisis stimulating agents.
3 Use of blood components effectively targets specific patient deficiencies and is a much more efficient use of the limited blood supply than use of whole blood.
4 Transfusion of leukocyte blood that has been stored less than 2 weeks is associated with better clinical outcomes including fewer organ failures; shorter time in the ICU and on the ventilator; and lower rates of death.
5 Massive transfusion of the actively bleeding patient should be guided by frequent measurements of hemoglobin, coagulation parameters, and platelet counts. Supplemental fresh frozen plasma and platelets are usually required after 5 to 10 units of packed red blood cells have been transfused.
▪ INDICATIONS FOR BLOOD PRODUCTS
No blood is as good as your own. Setting aside concerns of transfusion-related infection and immune reactions, transfused blood is substantially less robust than native blood in its primary function of carrying O2 to tissues and leaving it there. In addition, receiving another’s blood is associated with numerous risks outlined below, some of which are only partially understood. Therefore, unless absolutely necessary, it is not a good idea to receive allogeneic transfusion or perhaps even one’s own blood if it has been stored for more than several weeks. Despite the risks and limitations of transfusion, there are three situations in which blood product can be useful and even lifesaving: (i) to increase O2-carrying capacity, (ii) to reverse deficiencies of clotting factors, and (iii) to correct thrombocytopenia.
Tissue oxygen delivery (DO2) is the product of cardiac output, hemoglobin (Hgb) concentration and its saturation, plus the normally trivial contribution of O2 dissolved in plasma. DO2 remains adequate even in the face of profound anemia (Hgb of 3 to 4 gm/dL) if arterial saturation is normal and reductions in Hgb are offset by proportional increases in cardiac output or O2 extraction. Unfortunately, these compensatory mechanisms are often impaired in critically ill patients and saturation is rarely normal without supplemental O2. For these reasons, Hgb concentration has traditionally been maintained greater than 10 gm/dL, a value that corresponds to a hematocrit (Hct) ≥30%. By contrast, experimental evidence suggests that for most hemodynamically stable patients, substantially lower Hgb concentrations (approx. 7 gm/dL) are not only tolerated, but are also associated with better outcomes. Similarly, the lower acceptable limit for Hgb can be relaxed in patients with long-standing anemia and in those whose tissues and cardiac performance accommodate to chronically reduced O2 delivery (e.g., chronic renal failure). Conversely, traditional Hgb goals may be more important in patients with coronary or carotid ischemia, refractory hypoxemia, or limited cardiac reserve. Increasing Hgb however, does not always increase DO2; as the Hct rises above 40%, increases in viscosity eventually reduce overall DO2 (Fig. 14-1). In addition, depletion of 2,3-DPG (diphosphoglyceric acid) in stored blood results in a leftward shift in the oxyhemoglobin dissociation curve resulting in less tissue O2 delivery
than that of an identical concentration of native Hgb. Numerous other changes in stored red blood cells (RBCs), outlined below, further limit DO2.
than that of an identical concentration of native Hgb. Numerous other changes in stored red blood cells (RBCs), outlined below, further limit DO2.
Administration of blood products solely to increase circulating volume is probably only rational in the setting of acute bleeding where there is concomitant anemia and coagulopathy (e.g., massive hemorrhage in trauma or bleeding in the patient with hepatic failure or disseminated intravascular coagulation [DIC]). However, even in hemorrhagic shock it makes sense to replace deficits with a combination of packed red blood cells (PRBCs) and crystalloid, unless massive losses necessitate supplemental plasma and platelet transfusion. In most settings, intravascular volume depletion can be corrected as quickly, with less risk and cost, using crystalloid solutions or non-bloodcolloid.
There are clear indications for treatment with clotting factors, where no other option will suffice. These situations include (i) bleeding from congenital or acquired isolated factor deficiencies; (ii) hemorrhage due to multiple factor deficiencies from warfarin toxicity, hepatic failure, or consumptive or dilutional coagulopathy; and (iii) correction of severe thrombocytopenia resulting in hemorrhage.
▪ ANEMIA
Approximately one third of patients are anemic at the time of intensive care unit (ICU) admission, and nearly half develop anemia by the third day in ICU. Greater than 85% of all patients with an ICU stay exceeding a week receive a blood component; and of the transfused group almost half receive more than 5 units of blood products. The incidence of anemia is understandable because patients enter the ICU with chronic diseases and while their RBC production is blunted by functional iron deficiency and by decreased erythropoietin production and sensitivity. Critically ill patients are also subjected to intense phlebotomy typically losing 40 to 70 mL of blood daily, a value that outstrips production capacity.
▪ BLOOD PRODUCT CONSERVATION
Because of stricter rules for donation, blood supplies are decreasing while demands are increasing. The good news is that donor selection and enhanced blood testing have made blood supply safer now than ever with regard to traditional transfusion risks (e.g., viral hepatitis, human immunodeficiency virus [HIV]). Unfortunately, emerging data suggest transfusions may be associated with heretofore under or unappreciated hazards (e.g., immune modulation). Furthermore, blood products are very expensive in many locations: a unit of PRBCs now costs approximately $1,000. Because blood is increasingly scarce, expensive, and has potential dangers, it makes sense to limit the need for its use.
Perhaps half of all RBC transfusions could be averted if the volume and frequency of blood drawing were minimized, thresholds for transfusion were sensibly reduced, and erythropoiesis stimulating agents (ESAs) thoughtfully administered. Simple
first steps to RBC conservation are the prohibition of “standing orders” for blood tests, reduced use of indwelling catheters, and whenever possible, use of alternative monitoring methods to minimize phlebotomy. Routine morning evaluations of chemistry and hematology panels and arterial blood gasses are unnecessary and wasteful for most patients. Blood test orders should be driven by clinical indication, not habit, or the solar cycle. Because they make obtaining samples easy, central venous and arterial catheters encourage sampling more frequently than is clinically necessary. Techniques such as bedside testing of blood glucose and the use of pulse oximetry and capnography to determine arterial O2 and CO2 tensions, respectively, can decrease bloodletting. ICU-based, small-volume, multichannel chemistry-blood gas analyzers can further reduce the quantity of blood used for monitoring as long as clinicians do not increase the frequency of testing because results are easy to obtain. Use of “cell savers” in trauma victims and in patients undergoing bloody surgical procedures is also prudent. Highly automated systems now collect shed blood from operative sites, mix it with an anticoagulant, wash the collected mixture, concentrate the RBCs, and then reinfuse them through a filter. For patients undergoing some elective operations, autologous transfusion is another technique to avoid donated cells.
first steps to RBC conservation are the prohibition of “standing orders” for blood tests, reduced use of indwelling catheters, and whenever possible, use of alternative monitoring methods to minimize phlebotomy. Routine morning evaluations of chemistry and hematology panels and arterial blood gasses are unnecessary and wasteful for most patients. Blood test orders should be driven by clinical indication, not habit, or the solar cycle. Because they make obtaining samples easy, central venous and arterial catheters encourage sampling more frequently than is clinically necessary. Techniques such as bedside testing of blood glucose and the use of pulse oximetry and capnography to determine arterial O2 and CO2 tensions, respectively, can decrease bloodletting. ICU-based, small-volume, multichannel chemistry-blood gas analyzers can further reduce the quantity of blood used for monitoring as long as clinicians do not increase the frequency of testing because results are easy to obtain. Use of “cell savers” in trauma victims and in patients undergoing bloody surgical procedures is also prudent. Highly automated systems now collect shed blood from operative sites, mix it with an anticoagulant, wash the collected mixture, concentrate the RBCs, and then reinfuse them through a filter. For patients undergoing some elective operations, autologous transfusion is another technique to avoid donated cells.
ESAs like erythropoietin and darbepoetin comprise another element of an RBC conservation strategy. When administered just once a week, 40,000 U of recombinant human erythropoietin (rhEPO) has been shown to reduce the volume of RBCs transfused. Perhaps more important, about 10% of patients have avoided RBC transfusion altogether. Use of rhEPO requires the clinician to plan ahead; on average, a dose given today stimulates manufacture of the equivalent of roughly 1 unit of RBCs by next week. The lag time to produce results and the cost of rhEPO (about $300 weekly) have been barriers to use. One rational strategy for ESA use is (i) begin weekly ESA injections at the time of admission for all anemic, critically ill patients who are thought to be salvageable and likely to have at least a 7-day hospital stay (e.g., severe sepsis, ARDS); (ii) at the time of admission, withhold ESA treatment from moribund patients, nonanemic patients, and those anticipated to have short ICU stays (e.g., asthma or COPD exacerbation, drug overdose, diabetic ketoacidosis); and (iii) reassess the therapeutic plan at 4- to 7-day intervals. For example, it is reasonable to begin therapy for an anemic COPD patient initially thought likely to have a short stay but who develops nosocomial pneumonia and progressive anemia, or a patient initially believed to be moribund who rallies. Conversely, it is sensible to discontinue ESAs from a patient dying with inexorable multiple organ failure. When using ESAs, the target should not be a normal or supranormal Hgb, doing so is associated with a higher risk of complications including thromboses.
The use of fresh frozen plasma (FFP) and platelets can also be sharply curtailed by knowing a few simple facts and making several safe, easy changes in practice. The first is recognizing that bleeding, either spontaneous or following procedures or surgery, is uncommon with platelet counts greater than 20,000/mm3 and exceedingly rare with platelet counts greater than 50,000/mm3. Hence, unless the patient is bleeding or platelet function is known to be abnormal, withholding platelet transfusions until counts are less than 20,000/mm3 (certainly <50,000/mm3) can dramatically reduce the number of platelets transfused without compromise of safety. (There is some data to suggest that patients with consumptive coagulopathy may have a higher bleeding risk than patients with equivalent platelet counts without consumption.) Although not evidence based, some experts recommend maintaining periprocedural platelet counts greater than 50,000/mm3 for patients undergoing surgery or invasive procedures, especially if bleeding would pose a high risk. The administration of platelets to thrombocytopenic patients with thrombotic thrombocytopenic purpura (TTP) or heparin-induced thrombocytopenia (HIT) is not only wasteful, but it may also be harmful by producing thrombotic complications.
FFP is often administered to nonbleeding patients in an attempt to normalize a prolonged prothrombin time (PT) or activated partial thromboplastin time (aPTT), based upon the assumption that correcting the laboratory value is possible and doing so will decrease the risk of bleeding. This practice is perhaps most common in patients presenting with excessive warfarin effect or hepatic failure, but is also done for patients with DIC, vitamin K deficiency, or dilutional coagulopathy. Several observations suggest this practice should be limited if not abandoned; only a minority of patients given FFP have in vitro clotting studies corrected and the correction typically lasts only 2 to 4 h. Perhaps more fundamental is the fact that there is a poor correlation between modest PT and aPTT prolongations and risk of bleeding. Therefore, it makes
sense to withhold FFP from nonhemorrhaging patients with PT and aPTT prolongations less than three times normal. When vitamin K deficiency, warfarin effect, or hepatic dysfunction are etiologic, administration of vitamin K can often correct in vitro clotting abnormalities in less than a day, at much lower cost and risk. (An exception to this guideline occurs in patients with INRs > 10 to 20 from warfarin, in whom the risk of spontaneous bleeding is quite high and prompt correction with FFP may warrant the additional risks and costs of transfusion.) A nonevidence based and especially wasteful practice is the formulaic administration of platelets or FFP to patients, after a fixed number of red cells have been transfused.
sense to withhold FFP from nonhemorrhaging patients with PT and aPTT prolongations less than three times normal. When vitamin K deficiency, warfarin effect, or hepatic dysfunction are etiologic, administration of vitamin K can often correct in vitro clotting abnormalities in less than a day, at much lower cost and risk. (An exception to this guideline occurs in patients with INRs > 10 to 20 from warfarin, in whom the risk of spontaneous bleeding is quite high and prompt correction with FFP may warrant the additional risks and costs of transfusion.) A nonevidence based and especially wasteful practice is the formulaic administration of platelets or FFP to patients, after a fixed number of red cells have been transfused.
Emerging data suggest that reducing or better yet avoiding transfusion altogether can shorten the length of stay, and reduce organ failure rates, and mortality. Attention to blood conservation during a protracted ICU stay reduces cost, risk of transfusion complications, and workload for nurses and laboratory personnel.
▪ SOURCES OF BLOOD LOSS
Because Hgb and clotting function measurements are sensitive to error and artifact, each time a physician is confronted with an abnormal value, the validity of the result should be questioned. Failure to do so will result in unnecessary diagnostic tests and transfusions. One should be particularly skeptical when abnormalities develop suddenly and are large in magnitude, especially if they do not match the patient’s clinical appearance. In stable patients with no “visible” blood loss, an acute decline in Hct of nine points or more (the equivalent of 3 units of RBCs) should make the laboratory result suspect. Similarly, sudden apparent “pancytopenia” with proportional decreases in white cells, red cells, and platelets is likely to indicate that the sample was obtained from a catheter and was diluted by infusate.
When convinced that abnormal laboratory data are accurate, it is important to carefully consider the cause of the abnormality. Simply stated, where did the blood clotting factors or platelets go? With few exceptions, it is difficult to rapidly lose substantial red cell mass without external evidence of blood loss. In the ICU, the most common sources of visible blood loss are the gastrointestinal tract or surgical and traumatic wounds. Because blood is an emetic and cathartic, rarely will large quantities of blood be “concealed” in the gut for long. When a true acute drop in Hct is not accompanied by obvious bleeding, retroperitoneal, rectus muscle, or thigh (soft tissue) bleeding, alveolar hemorrhage, and hemolysis should be suspected. Retroperitoneal bleeding may be spontaneous in patients with thrombocytopenia or soluble factor deficiencies or warfarin excess, but more commonly therapeutic anticoagulation and trauma (including femoral artery or vein cannulation or vena caval filters placement) are the causes. Soft tissues of the thigh can clandestinely harbor large amounts of blood following femoral vessel puncture. Alveolar hemorrhage is most common in immunocompromised thrombocytopenic patients, occurring less often with Goodpasture disease, Wegener’s granulomatosis, or systemic lupus. Hemolysis may occur spontaneously, but in the ICU is more commonly drug or transfusion induced.
When production of platelets is diminished but consumption is not excessive, platelet counts usually decline slowly over 3 to 5 days. Therefore, sudden (<1 day) decreases in platelet counts are usually indicative of a consumptive process, often, for example, DIC or drug- (e.g., heparin) associated thrombocytopenia.
Similar to the situation with platelets, when production of soluble clotting factors is curtailed but consumption is not excessive, changes in the PT and aPTT times occur over several days. Rapid development of a coagulopathy is most likely related to accelerated factor consumption of sepsis-induced DIC or resulting from dilution of clotting factors by massive transfusion or resuscitation. The problems of thrombocytopenia and abnormal clotting studies are discussed at length in Chapter 30.
▪ THE COMPONENT SYSTEM
Most patients receiving transfusions do not require all the components available in whole blood (Table 14-1). Component therapy “stretches” the blood supply by allowing prolonged storage of stable constituents and by permitting several patients to receive the specific components they need from a single donation. For example, RBCs are routinely stored 5 to 6 weeks and after freezing, can be stored up to 10 years. FFP can be stored for months and purified clotting factors can be stored for years. The time limited component of blood is the platelet fraction, which lasts only days. By limiting administered volume, component therapy also reduces the risk of fluid overload,
the amount of transfused anticoagulant, and the risk of infection.
the amount of transfused anticoagulant, and the risk of infection.
TABLE 14-1 WHOLE BLOOD COMPONENTS | |||||||||||
---|---|---|---|---|---|---|---|---|---|---|---|
|
Consequences of Blood Storage
In the 1970s and 1980s, some physicians insisted their patients receive fresh whole blood because they believed better outcomes were achieved compared to use of component therapy. Advocates of the practice were often ridiculed because it seemed incredulous that giving whole blood could be any different than “remixing” just the needed components. Despite their advocacy, whole blood transfusion largely ended as the product became scarce; and with the rise of the AIDS epidemic, more dangerous. Two decades later, it is apparent that fresh whole blood may well have produced better outcomes, but we now know that important part of the equation was that the blood was fresh, not that it was whole. So what are the important differences between fresh and stored blood? Numerous abnormalities, collectively known as the “storage lesion,” have been identified in RBCs stored for two or more weeks. RBC rigidity, aggregation, and vessel wall adhesiveness are increased due to nitric oxide depletion and altered membrane lipid content. RBCs that lyse during storage release free Hgb which neutralizes endothelial bound nitric oxide causing vasoconstriction. Together, these changes impair capillary transit of RBCs. In addition, reductions in adenosine triphosphate (ATP) and 2,3-DPG inhibit tissue O2 release by shifting the oxyhemoglobin dissociation curve leftward. The 2,3-DPG depletion persists for 1 to 2 days after transfusion. Because of metabolism of glucose in the storage media and spontaneous lysis of RBCs, the plasma portion of stored blood also contains higher levels of lactate, ammonia, potassium, and iron than fresh blood. Finally, inflammatory cytokines and microvesicles of shed RBC cell membranes increase in stored blood.
Red Blood Cell Components
Whole Blood
Whole blood can be used for emergent restoration of circulating volume and O2-carrying capacity, but unless fresh, it is a poor source of clotting factors and platelets. (Platelets are nonfunctional within 24 h; within 48 h, essentially all factor VIII is depleted; and within a week, factors V and VII levels are negligible.) Furthermore, transfusion of whole blood may produce circulatory overload in the euvolemic patient who requires only RBCs (e.g., sickle cell disease, chemotherapy-induced anemia, myelodysplasia). A 500 mL “unit” of whole blood contains approximately 60 mEq of Na+ and has an average Hct of 35% to 40%. Whole blood is also more likely to contain microaggregates of leukocytes and platelets which may be detrimental. The only remaining indication for whole blood is in support of massively bleeding patients. Even then, whole blood must usually be supplemented by transfusions of platelets and/or plasma.
Packed Red Blood Cells
Removing the plasma from whole blood leaves a 200- to 300-mL unit of PRBCs having a Hct of 65% to 75%. PRBCs are used to restore O2-carrying capacity. A unit of transfused PRBCs should raise the Hct of an adult patient by approximately 3%. (Continued bleeding or excessive volume expansion blunts the expected increase.) PRBCs contain few platelets, clotting factors, or leukocytes and are not particularly effective as volume expanders when used alone. PRBCs can be infused as rapidly as whole blood when viscosity is reduced by adding approximately 75 mL of normal saline per unit.
PRBCs offer several advantages over whole blood: (i) less volume expansion for a given increase in O2-carrying capacity (each unit of PRBCs contains only 8 to 20 mEq of Na+, a particularly helpful feature in patients with heart or kidney failure); (ii) PRBCs contain less anticoagulant, reducing the potential risk of citrate toxicity; and (iii) lower plasma volume, reducing the risk of allergy, anaphylaxis, viral hepatitis, and immunologic reactions from transfused antibodies. There are several disadvantages of using stored PRBCs: (i) there is a significant decrement in posttransfusion viability
of the cells; (ii) stored RBCs release K+ into the surrounding plasma as they age with concentrations often reaching 90 mEq/L; and (iii) plasma ammonia concentrations rise.
of the cells; (ii) stored RBCs release K+ into the surrounding plasma as they age with concentrations often reaching 90 mEq/L; and (iii) plasma ammonia concentrations rise.
Specialized RBC Components
Five processing methods are employed to improve the safety or longevity of RBCs (Table 14-2). Leukocyte-poor RBC transfusions are used in patients who have experienced white cell or leukoagglutinin reactions; and for seronegative patients at-risk for cytomegalovirus (CMV) (CMV lives in white blood cells [WBCs]). Leukoreduction can be achieved by filtering, repeated washing, or freezing collected blood. Each method destroys some platelets while reducing the WBCs and plasma by approximately 75% to 90%. Because of the loss of RBCs in processing, a unit of leukocyte-poor RBCs contains less Hgb than a unit of PRBCs. Even leukocyte-poor RBCs contain small numbers of viable WBCs; therefore, blood products transfused into immunosuppressed patients must be irradiated to prevent graft versus host disease (GVHD). Even though it is probably a good idea to leukoreduce all RBCs, it is not done universally because the costs of doing so are prohibitive. Freezing or washing RBCs has additional advantages over filtration: the former two processes also minimize reactions caused by allergy to transfused proteins and those resulting from presence of anti-IgA or IgE antibodies.

Full access? Get Clinical Tree
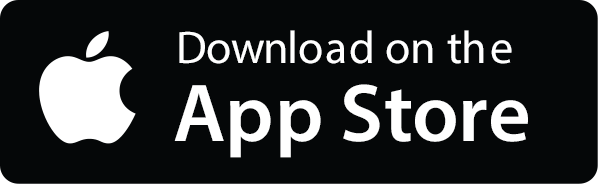
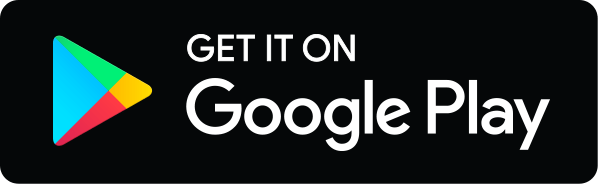
