© Springer Science+Business Media New York 2015
Rifat Latifi, Peter Rhee and Rainer W.G. Gruessner (eds.)Technological Advances in Surgery, Trauma and Critical Care10.1007/978-1-4939-2671-8_1717. Biology of Nutrition Support and Gut Access in Critically Ill Patients
(1)
Department of Primary Care and Emergency Medicine, Kyoto University Hospital, Kyoto City, Japan
(2)
Department of Surgery, University of Arizona Medical Center, Tucson, AZ, USA
Keywords
Nutrition supportBiology of nutrientsn-3 fatty acidGlutamineArginineLeucineBCAAWhey proteinLactoferrinBackground
Nutrition support for critically ill and injured patients has undergone significant advances in recent decades as the direct result of scientific progress and our increased knowledge of the biology and biochemistry of key nutrient changes induced by injury, sepsis, and other critical illnesses, both in adults and in children. The science of nutrition support (or, more accurately, of nutrition therapy) has become more disease-based. Also called “specialized” or “artificial” nutrition support, nutrition therapy refers to the provision of either enteral nutrition (EN) via tube feeding or total parenteral nutrition (TPN). In contrast, “standard therapy” refers to a patient’s own volitional intake, without the provision of nutrition therapy [1].
Of all the advances in nutritional support, the development of Total Parenteral Nutrition by Dr. Stanley J. Dudrick and his team is the most significant event to have clearly changed the way we think about nutrition, the way we feed the critically ill and the way we support these patients who cannot, should not, or will not be fed orally [2]. Furthermore, in the past three to four decades, the understanding of the molecular and biological effects of nutrients in maintaining homeostasis in the critically ill population has made exponential advances. Traditionally, nutrition support in the critically ill population was regarded as adjunctive care designed to provide exogenous fuels to support the patient during the stress response. This support had three main objectives: to preserve lean body mass, to maintain immune function, and to avert metabolic complications. Recently, these goals have become more focused on nutrition therapy; specifically attempting to attenuate the metabolic response to stress, prevent oxidative cellular injury, and favorably modulate the immune response.
Depending on the individual patient’s metabolic needs, nutrition therapy helps ensure that key nutrient substrates are replenished, or added in larger amounts, to supplement specific deficiencies or to simply prevent further deterioration and clinical consequences. The benefit of early institution of either EN or TPN in the overall care of critically ill and injured patients has now been well-established. After a critical illness or injury, the patient’s energy and overall metabolic requirements greatly increase in order to sustain increased metabolism and the process of wound repair. Given current evidence derived from randomized controlled trials (RCTs), early provision of nutrition for critically ill and injured patients is now a level I recommendation [3].
Critical illness and tissue injury initiate a complex series of rapidly responding homeostatic events in an attempt to prevent ongoing tissue damage and to activate the repair process. Classically, inflammation has been recognized as the hallmark of the homeostatic response. But more recently, attention has been focused on defining the complexities of response at the cellular, metabolic, and molecular levels. There is mounting evidence regarding the multiple specific metabolic changes in critically ill and injured patients and their need for fundamental nutrients and special substrates.
The benefit of the early institution of adequate enteral or parenteral nutrition in the overall management of critically ill patients has been well-established. Early nutritional support has the potential to reduce disease severity, diminish complications, and decrease the intensive care unit (ICU) length of stay and favorably impact patient outcome. Recognizing the importance of the appropriate nutritional support of trauma and critically ill patients with severe and/or multiple injuries, we have implemented a standard nutritional assessment and support program that aligns with guidelines set by the SCCM, ASPEN, ESPEN, and EAST. Despite all of this, it is very difficult to extrapolate nutritional support alone as the major factor impacting the patient’s outcome. Sophisticated delivery tubing systems for enteral feeding, complex pharmacological mixtures of various nutrients in the form of parenteral nutrition, and recognition of various nutrients as pharmaco-nutrients have exploded in recent years. Gut access for provision of nutrition support has undergone major changes as well. Advanced laparoscopic approaches, percutaneous endoscopic access, and fine technology of these tubing systems have been refined continuously for the last few decades. In the following pages we describe some of the key nutrients and their effect on the biology of the disease.
n-3 Fatty Acids
Pharmacological Effects of n-3 Fatty Acids
Fatty acids are categorized as either saturated or unsaturated fatty acids. Monounsaturated fatty acids have one double bond while polyunsaturated fatty acids have two or more double bonds. Depending on the number of carbon atoms, fatty acids are also referred to as “short chain” (<8 carbons), “medium chain” (8–14 carbons), or “long chain” (≥15 carbons) fatty acids. Unsaturated fatty acids are also distinguished by where the first double bond occurs, counting from the carbon atom at the very end. These are typically designated ω3 (n-3), ω6 (n-6), and ω9 (n-9). The role of fatty acids include important aspects such as (1) sources of energy, (2) components of cell membranes, and (3) conversion into lipid metabolites that take part in physiological activities (e.g., prostagladins). Fatty acid administration leads to their incorporation into cell membranes. Arachidonic acid, which is an n-6 series fatty acid, plays a role in the formation of cell membranes, along with the n-3 series fatty acids eicosapentaenoic acid (EPA) and docosahexanoic acid (DHA). These fatty acids are discharged from cell membranes if cell injury or phospholipase activation occurs in association with a cellular insult. Although n-3 and n-6 fatty acids are metabolized by the same enzymes (cyclooxygenase and 5-lipoxygenase), the final metabolic products are different. When inflammatory mediators such as prostaglandin (PG) E2, thromboxane (TX) A2, or leukotriene (LT) B4 are produced from n-6 fatty acids in excessive amounts, neutrophil activation is triggered which leads to systemic inflammatory response syndrome (SIRS). PGE3, TXA3, LTB5, and other similar factors are produced from n-3 fatty acids; they are known to compete with metabolites of n-6 fatty acids and bring about anti-inflammatory activity. Reports indicate that n-3 fatty acids inhibit the production of the inflammatory cytokines interleukin (IL)-1 and tumor necrosis factor (TNF) by neutrophils and reduce the production of inducible nitric oxide synthase (iNOS) by macrophages. Furthermore, it has been demonstrated that n-3 fatty acids metabolize into resolvins and protectins, which promote convergence of inflammation, and have gained particular attention for their anti-inflammatory effects. The anti-inflammatory effect of n-3 fatty acids has been corroborated by many fundamental studies.
Clinical Studies Involving n-3 Fatty Acids
n-3 fatty acids is commonly found in a number of IEN or IMN, but one formula that has received particular attention is known that Oxepa® (Abbott Labs, Abbott Park, IL), which contains EPA, DHA, γ-linoleric acid (GLA), and antioxidants, is recommended for Acute lung injury (ALI) and Adult respiratory distress syndrome (ARDS) by guidelines in Europe, the USA, Canada, and other countries [3–5].
Pontes-Aruda et al. [6] studied 165 patients with severe septicemia involving acute lung injury (ALI) and acute respiratory distress syndrome who received Oxepa® and Pulmocare® (Abbott Labs, Abbott Park, IL). They reported that Oxepa® significantly improved the survival rate (67.3 % vs. 47.9 %) and decreased the number of days under artificial respiration control (14.6 days vs. 22.2 days) and the number of days patients remained in the intensive care unit (ICU) (17.2 days vs. 23.4 days). Furthermore, significant improvements were seen in oxygenating function and there was a significant reduction in the occurrence of new organ dysfunction. The fact that the control group in this study received a nutritional supplement that contained 55 % n-6 polysaturated fatty acid became an issue. In a study of the effectiveness of Oxepa® that included 106 patients with early-stage septicemia, the control group was given an ordinary nutritional supplement that contained 29 % fatty ingredients [7]. In that study, Oxepa® significantly decreased the incidence of septicemia (26.2 % vs. 51.0 %), the incidence of new organ dysfunction such as cardiovascular failure (21.0 % vs. 36.2 %) or respiratory failure (24.6 % vs. 39.6 %), the number of days patients remained in the ICU (7 days vs. 13 days), and the number of days patients remained in the hospital (9 days vs. 19 days). However, there was no significant change in mortality rates. Grau-Carmona et al. [8] compared Oxepa® to an ordinary nutritional supplement that contained 30 % fatty acids in 133 patients with septicemia. In that study, Oxepa® did not lead to any significant differences in mortality, oxygenation function, infection outbreak rate, or organ dysfunction incidence rate. However, Oxepa® was associated with significantly fewer days in the ICU (16 days vs. 18 days). Investigators also conducted a randomized controlled trial to examine the effectiveness of enteral bolus administration of EPA and DHA to patients with ALI. Stapleton et al. performed bolus administration of EPA and DHA to 90 patients with ALI and compared them to a group that did not receive this treatment. The group treated with bolus administration experienced a significant rise in the concentrations of EPA and DHA in the blood, but no significant differences were found in proinflammatory cytokine levels in the bronchoalveolar lavage fluid. Furthermore, there were no significant differences in mortality, number of days in the hospital, number of days in the ICU, or organ dysfunction incidence. Rice et al. [9] performed bolus administration of EPA, DHA, GLA, and antioxidants in 272 patients with ALI and compared them to a group that did not receive this treatment. The group that received the bolus administration had a significantly increased concentration of EPA in the blood but no significant differences were found in proinflammatory cytokines in the blood or in LTB4 concentration. Furthermore, a significant rise in the rate of death was observed in the group that received the bolus administration. It is necessary to consider the rise in death rate observed after bolus administration of EPA, DHA, and GLA separately from the anti-inflammatory effects of Oxepa® that support the guidelines.
Fat emulsions containing fish oil (EPA and DHA) have been widely administered intravenously, primarily in Europe, and clinical studies of their effectiveness have been conducted [10]. A recent meta-analysis of critically ill patients conducted by Manzanares et al. [11] confirmed a tendency toward decreased mortality and shortened period of mechanical ventilation; however, no effect was found on the infection rate or number of days in the ICU. Pradelli et al. [12] conducted a meta-analysis of patients who underwent elective surgery and critically ill patients, and they found significant decreases in infection rates, the number of days in the ICU, and the number of days in the hospital. When Palmer et al. [13] conducted a meta-analysis of critically ill patients in a similar manner, the conclusions were somewhat different; they reported no improvements in mortality, infection rate, or number of days in the ICU in patients who used fish oil. Currently, clinical studies have not yielded consistent conclusions. Although the issue of the sale of fish oil in the USA and Japan has not yet been available, there are physicians who are awaiting the permission of usage fat emulsions containing fish oil.
Glutamine
Pharmacological Effects of Glutamine
Glutamine is synthesized from other amino acids. Therefore, it is not necessary to ingest it as a nutrient when cells are not subject to insult. However, when the body is experiencing an insult such as trauma and sepsis the demand for glutamine increases, and the amount that can be synthesized by the body cannot keep up with demand. For this reason, it is considered a conditionally essential amino acid. Supplementation with glutamine to make up for relative shortages is considered beneficial for the body. Glutamine plays a diverse range of roles and can be converted into metabolites such as ammonia, nucleic acids, glutathione, arginine, and glucosamine. When the body is experiencing an insult, glutamine acts as an energy substrate in the intestinal mucosa, lymphocytes, macrophages, and neutrophils, and administration of glutamine to the intestinal tract is considered effective for sustaining the intestinal tract barrier function and preventing bacterial translocation. Many reports have demonstrated that glutamine increases levels of heat shock proteins (HSPs) as an anti-inflammatory mechanism. Intravenous administration of glutamine after the onset of septicemia activates the transcription factor heat shock factor-1, causing expression of HSP-70 and HSP-25 and thereby reducing the occurrence of acute lung disorders. Similarly, HSP-32 (heme oxygenase-1) is also expressed to reduce apoptosis and decrease tissue damage in the intestinal tract [14]. A report indicated that administration of glutamine inhibits apoptosis following stress or injury, and depletion of glutamine triggers apoptosis, which then activates caspase-3 and -9. Intravenous administration of glutamine inhibits the activation of nuclear factor-κB in the septicemia model [15]. It also inhibits activation of the stress-related p38 mitogen-activated protein kinase and extracellular signal-related kinase, resulting in reduced TNFα, IL-6, and IL-18. Similarly, enteral administration of glutamine has been shown to reduce serum IL-6 levels, increase IL-4 production by lymphocytes, and reduce interferon-γ production [16]. In an ischemia reperfusion model where a jenunal pouch was prepared and glutamine was administered, researchers confirmed inhibited activation of activator protein-1 and enhanced activation of peroxisome proliferator-activated receptor (PPAR). PPAR has drawn attention as a transcription factor with anti-inflammatory functions that is associated with reduced iNOS, reduced accumulation of neutrophils in the jejunum, retention of adenosine triphosphate levels in the jejunum, inhibition of intestinal tract permeability, and reduced tissue damage. Another study found that syndecan 1 was involved in reducing the action of glutamine in intestinal tract ischemia reperfusion damage [17]. It has also been noted that there is potential for arginine conversion through metabolism of glutamine to produce nitric oxide (NO), which can be harmful.
Clinical Studies Related to Glutamine
Many clinical trials have supported the usefulness of glutamine administration for reducing the infection rate during insult to the body. The results of large-scale clinical studies for determining the effectiveness of glutamine administration (enteral administration or intravenous administration) have been debatable, as some papers have cast doubt on the effectiveness of glutamine. Wernerman et al. [18] conducted a clinical study involving intravenous administration of glutamine (0.28 g/kg/day). The targeted number of cases was 1,000 over a 4-year period at 9 facilities in Sweden, 1 facility in Norway, and 1 facility in Finland (11 facilities in total). However, the study did not reach the target number of subjects; instead, the study included only 413 cases. Intravenous administration of glutamine in targeted patients with Acute Physiology and Chronic Health Evaluation II scores of 10 or higher, as well as in some mild cases, confirmed differences in the ICU death rate and the 28-day death rate; however, no difference was found in the mortality over a longer period. Andrews et al. [19] conducted a clinical study involving intravenous administration of glutamine (20.2 g/day) and intravenous administration of selenium (500 μg/day); this was known as the SIGNET trial. Analysis of 502 cases from ten ICU facilities in Scotland could not confirm that glutamine effectively reduced the infectious disease occurrence rate, number of days in the ICU, or the death rate. Heyland et al. [20] found astounding results when they conducted a large-scale clinical study of glutamine administration (intravenous administration of 0.35 g/kg/day and enteral administration of 30 g/day) along with selenium and antioxidant administration. They analyzed 1,223 critically ill patients with dysfunction of two or more organs from 40 facilities in Canada, the USA, and Europe, and found that the death rate increased with glutamine. The results of this study, known as the REDOX study, indicate that excessive amounts of glutamine must not be administered to patients with highly critical illnesses. It is important to note that this study did not completely refute the benefits of glutamine yet. Additionally SIGNET Trial [19] and REDOX Trial [20] tested the antioxidant nutrient using selenium. There was a reduction in the infection rate after intravenous administration of selenium (500 μg/day) for 5 days or more during the SIGNET Trial. However, in the REDOX study, when intravenous selenium administration (500 g/day) and enteral administration of selenium (300 μg), zinc (2 mg), β-carotene (10 mg), Vitamin E (500 mg), and Vitamin C (1,500 mg) was compared to groups without them, there were no differences in outcomes between the two groups.
Arginine
Pharmacological Effects Related to Arginine
Similar to glutamine, arginine is a conditionally essential amino acid. Arginine is metabolized in the body primarily through two routes [21]. One route leads to ornithine and urea owing to the action of arginase, while the other route leads to NO and citrulline because of the action of nitric oxide synthase (NOS). Arginase I is found primarily in the liver, and ornithine is metabolized further into polyamine and proline to improve collagen synthesis and wound healing. Alternatively, arginase II occurs primarily outside the liver and produces creatine in the small intestine mucous membrane to store muscle energy. Arginine increases the secretion of hormones such as growth hormones, glucagon, prolactin, and somatostatin, raising immune function when insults occur. Although appropriate concentrations of ornithine are essential for sustaining immune function, a report found that the differentiation of B-cells and the number of intestinal Peyer patch cells deceased in mice with a large amount of arginase, inhibiting production of T-cells and the T-cell receptor CD3. An appropriate amount of NO is essential for bactericidal action, and the vasodilation action of NO improves microcirculation, making oxygen transport to the periphery possible. However, excessive NO production leads to peroxynitrite production and tissue damage and can cause septic shock owing to vasodilation. The three arginase I and II enzymes as well as iNOS are all induced when inflammation occurs, and arginase and NOS compete in immune cells. There is a rise in the demand for arginine and because the metabolic behavior of arginine changes according to its concentration, it is difficult to confirm or repudiate the benefits of arginine.
Trends of Clinical Studies Related to Arginine
There have not been any clinical studies of arginine administration alone; clinical studies have used arginine-containing nutritional supplements that are commercially available. Galban et al. [22] studied 176 septicemia patients and reported that the group that received a nutritional supplement with enhanced arginine had a significantly reduced death rate compared to the control nutritional supplement group (19 % vs. 32 %). Kieft et al. [23] studied 597 ICU patients and compared a group that received a nutritional supplement containing enhanced arginine to a control group. They found that there were no differences between the groups in death rate, infection rate, or the number of days in the ICU. A meta-analysis of septicemia patients and critically ill patients except those with external injuries or burns, who received arginine found no differences in mortality or infection rate [24]. However, arginine administration in patients undergoing elective surgery has been found to reduce the infection rate and shorten the number of days in the hospital. It is also economically beneficial. Arginine administration in patients with critical septicemia for whom the hemodynamics may deteriorate is considered a situation to be avoided from a theoretical perspective [25].

Full access? Get Clinical Tree
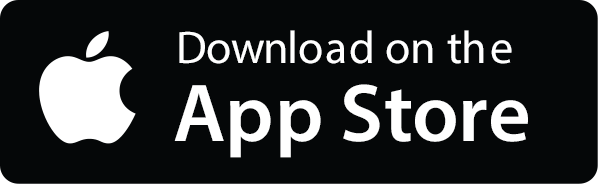
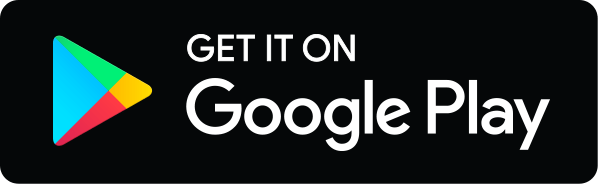