Chapter 9 Automatic ventilators
In order to inflate a patient’s lungs adequately with a mechanical ventilator, sufficient pressure must be generated in the respirable gas within a ventilator or resuscitator (positive pressure ventilation) to overcome the elastic recoil of the lungs and chest wall (their elastance) and the resistance to flow within the airways. These may be normal in healthy patients, requiring the generation of only modest pressures for inflation, or may be grossly abnormal in disease, requiring the generation of much higher pressures in order to provide the same degree of ventilation. Furthermore, some surgical procedures may make it more difficult to inflate the lungs, for example, by restricting the movement of the diaphragm, due to posture or internal intervention. An additional factor during anaesthesia is the resistance of the artificial part of the airway, which may increase accidentally, for example, by mucus accumulation or kinking of the endotracheal tube.
Positive pressure ventilators
Methods of pressure generation
Respirable gas with sufficient pressure to ventilate a patient’s lungs may be supplied by a ventilator that obtains gas at atmospheric pressure and compresses and stores this in a bellows or bag by mechanical means (Fig. 9.1A, B and C). Alternatively, a ventilator can be designed to utilize a compressed gas from a cylinder or pipeline supply (Fig. 9.1D) and reduce this to suitable respirable pressures without the requirement of a storage bellows.
Classification of ventilators
Power
Low-powered ventilators
With the advent of modern electronics, many ventilators that fit this classification exclusively have become obsolete in the developed world. Some older ventilators and some of those designed for the developing world are so constructed that they can only deliver modest pressures (by using weak springs or light weights to compress the respirable gas in a bellows (Fig. 9.1A)). Such machines are simple to operate, reduce the potential incidence of barotrauma to lungs and, more pertinently, do not require an electrical power supply and are essentially user serviceable.
However, many current electronic high-powered ventilators have a pressure-controlled mode that allows operative characteristics similar to low-powered ventilators. When used in this mode and the inspiratory pressure is limited to 15–25 cm H2O via the machine’s electronics, the ventilator may be considered as low powered.
High-powered ventilators
In order to prevent a reduction in ventilator performance in the presence of deteriorating lung conditions, a ventilator needs to be powerful enough to overcome the increases in airways resistance and reduction in compliance with little alteration in desired gas flow. These ventilators require also the addition of certain safety features to protect patients with both normal and abnormal lungs from excessive pressures. For example, an overpressure safety valve is always included in the gas pathway to the patient to release any build-up of potentially dangerous pressures that might damage the lungs. Fig. 9.1B shows an example of a typical high-powered ventilator. The pressure-relief valve (S) can either be pre-set (usually at 4.4 kPa/45 cm H2O) or, in more sophisticated machines, can be adjustable (up to 7.8 kPa/80 cm H2O) to cope with severe conditions such as asthma and the adult respiratory distress syndrome. Higher-pressure relief settings, however, equate to increased risk of barotrauma.
Those high-powered ventilators that always generate high pressure of gas in the ventilator system prior to its delivery (by using powerful springs, heavy weights or a pipeline gas source (Fig. 9.1C and D) ), require the presence of a further safety device, a flow restrictor (see below), in the inspiratory pathway. This reduces the flow to the patient and prevents too rapid a build-up of pressure in the lung.
Alternative classifications
A popular classification with British anaesthetists has been described by Mushin.1 Those ventilators that by their design produce a pressure sufficient only to ventilate normal or mildly abnormal lungs are classified as pressure generators, i.e. the tidal volume delivered to the patient is limited by the pressures generated. Those ventilators that develop pressures sufficiently high enough to deliver a desired flow even to grossly abnormal lungs are deemed flow generators. However, as most other electromechanical devices in common usage are described in terms of power, the author prefers the first classification.
lnspiratory characteristics of ventilators
Low-powered ventilators
Low-powered ventilators deliver gas at modest pressure. This pressure is normally constant (Fig. 9.2A) and will produce an inspiratory flow rate of gas that is greatest in early inspiration, when the pressure differential between the ventilator and the lung is wide, but that slows during inflation of the lung as the pressures approximate (Fig. 9.2B).
High-powered ventilators
Inspiratory characteristics will depend on a number of factors. The high driving pressure from a pipeline source or heavy weighted/spring-loaded storage bellows requires some form of flow restriction to prevent too rapid a rise or an excessive pressure transmitted to the patient’s lungs that could produce barotrauma. This may take the form of a fixed orifice restrictor. Here the flow will be constant (pipeline supply or weighted bellows) or gradually decreasing (spring loaded bellows) as the tension in the spring reduces with emptying of the bellows (Fig. 9.3A). However, practically in the case of the latter the reduction is insignificant and flow is virtually constant.
In more sophisticated ventilators, the inspiratory flow valve acts as a variable flow restrictor (Fig. 9.3B). These are able to respond to user-programmed inspiratory flow patterns.
Ventilators may be designed to force their bellows to be compressed either mechanically, via a linkage from a suitable power source, or pneumatically, by placing the bellows in a gas-tight container into which a pressurized gas source is fed (bag-in-bottle arrangement). The bellows in this type of ventilator normally fills with gas at near atmospheric pressures, so that when it is compressed, the pressure developed rises as it overcomes the resistive properties of the lungs. The resultant pressure and flow waveforms are dependent on the type of mechanical linkage (e.g. rotating cam/linear motor) or the type of pneumatic drive producing any of the waveforms seen in Fig. 9.3. Although these ventilators are classified as high powered, they do not require flow restrictors as there is no initial very high-pressure source present. However, they do need overpressure relief valves to protect against high pressures that might develop unexpectedly.
Classification of ventilators according to cycling
Expiratory cycling
• an expiratory volume-cycled ventilator may have a mechanism for terminating the expiratory phase when the reservoir bellows has filled to the desired tidal volume required for the next inspiration
• an expiratory pressure-cycled ventilator would be able to identify a selected airways pressure at the end of exhalation that would trigger the next inspiratory phase
• an expiratory flow-cycled ventilator would switch to the inspiratory phase when the desired flow rate at the end of exhalation was reached, or
• an expiratory time-cycled ventilator that terminates the expiratory phase after a predetermined time. This is the most versatile type as its phase may extend beyond the end of patient exhalation, unlike the others. It is, therefore, the most popular method of expiratory cycling and is achieved by using electronic or pneumatic timers within the ventilator to switch phases.
Further explanations are included in the individual ventilators mentioned below.
Cycling mechanisms in ventilators
Examples of these will be described where appropriate in the section on individual ventilators.
Ventilation modes
As ventilation strategies developed to include the ability to synchronize delivered tidal volume with the patient’s respiratory effort, the term synchronized intermittent mandatory ventilation (SIMV) became ubiquitous. A variety of approaches – many fundamentally similar – exist to the delivery of this type of ventilation by the different manufacturers, each, unfortunately, tending to use their own proprietary nomenclature (see Chapter 10).
In addition, a sophisticated ventilator may have a facility that supports spontaneous respiration by sensing an inspiratory breath and assisting it by adding extra gas from the device. This may be termed assisted spontaneous breathing (ASB) or pressure support ventilation (PSV). This may also be used in conjunction with SIMV. There are a number of other strategies used by manufacturers, which are explained in more detail in Chapter 10.
Ventilator controls (general principles)
For example, if the inspiratory time is 2 s and the expiratory time is 4 s then:
Classification of ventilators according to application
Mechanical thumbs
The most common source of pressurized gas is that found in cylinders and pipelines. This may be administered to a patient most easily as a continuous flow into the simplest of breathing systems, the T-piece (Fig. 9.4A). In Fig. 9.4B, the anaesthetist has occluded the open end of the T-piece with his thumb. The force of the fresh gas flow (FGF) inflates the patient’s lungs until the anaesthetist removes his thumb from the open end, which allows expiration to occur (Fig. 9.4C). By rhythmical application of the thumb to occlude the T-piece, intermittent positive pressure ventilation (IPPV) is achieved. The FGF has to be high enough to inflate the lungs during inspiration and, as it is not stored during exhalation, this method is wasteful of gas. Therefore, it is suitable only for use in neonatal anaesthesia. Furthermore, the advent of more efficient ventilators and gas monitoring has seen the usage of this type of ventilation decline.
In ventilators such as the Sechrist (Fig. 9.5D), the anaesthetist’s thumb is replaced by a pneumatically operated valve (Fig. 9.5E), the cycling of which is determined by the settings on the ventilator controls.
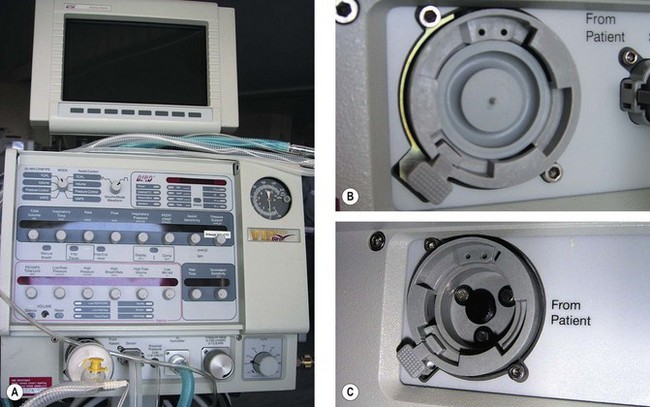
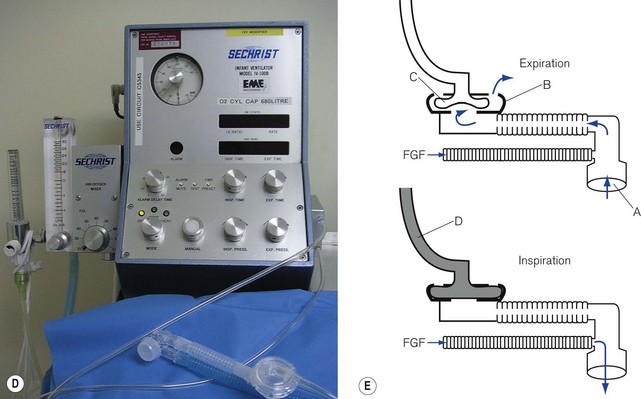
Figure 9.5 A. Bird VIP neonatal ventilator; B. Silicone diaphragm of Bird VIP acting as a variable flow valve; C. Bird VIP Piston from flow valve that moves the diaphragm. D. Seechrist infant ventilator; E. schematic diagram of the ‘mechanical thumb’. A, patient connection; B, expiratory valve; C, deflated pneumatic valve; D, gas supply tube to pneumatic valve, which is now inflated.
The exhalation valve may be electronically controlled (see Solenoids and Variable flow control valves, below) and by varying the degree of occlusion of the FGF is able to produce different types of inspiratory waveform (Bird VIP, Figs 9.5A, B and C). Some designs use gas jets in the opposite direction to the fresh gas in place of the valve for this purpose (SLE 2000).