Chapter 22 Assessment of Cardiovascular Function
Pediatric patients undergoing surgical treatment for congenital heart disease (CHD) or those with severe systemic illnesses such as sepsis and other causes of multiple organ system failure commonly have impaired cardiovascular function.1,2 In addition to treating the primary disease process, the pediatric intensivist should use strategies to reliably assess and monitor cardiovascular function, which specifically involves assessing adequacy of oxygen delivery (DO2) and systemic perfusion pressure, the primary determinants of tissue oxygenation.
Cardiovascular Function
The function of the heart and vasculature is to deliver oxygen (O2) and other nutrients to various tissues in order to meet the metabolic demands of the organism. Mild to moderate depression of DO2 normally is compensated by augmented O2 extraction at the tissue level, thereby maintaining a stable level of oxygen consumption (VO2). When DO2 falls below some critical level, this compensatory mechanism fails and a state of O2 supply dependency exists3 such that any further drop in DO2 leads to a parallel fall in VO2.4–6 Under a state of supply-dependent O2 consumption, affected tissues and organs attempt to maintain homeostasis partly through anaerobic metabolism. Several studies suggest the initial metabolic response to hypoxemia or decreased DO2 differs between the newborn and older ages and varies among different vascular beds. In adults at rest, DO2 is in great excess of VO2. This “O2 surplus” means moderate reductions of O2 transport are generally well tolerated without compromise of VO2. In contrast to the adult, the metabolism of the newborn may be particularly susceptible to modest alterations in O2 transport because of the high resting demands for O2, the ease with which these demands can be increased by small environmental changes, and the apparently limited reserve for augmenting cardiac output (CO) or O2 extraction acutely.7,8 Thus it is crucial that cardiovascular assessment and monitoring in the pediatric intensive care unit (ICU) involve continuous and reliable evaluation of the adequacy of systemic perfusion and DO2 in order to select appropriate hemodynamic support strategies.
Quantity of Therapy
If one considers hemodynamic monitoring not only in terms of DO2 and perfusion pressure but also in terms of what therapy is required to produce a given level of tissue oxygenation, one gains a much better understanding of the overall “condition” of the patient. It is therefore important to monitor not only DO2 and perfusion pressure but also the “quantity of therapy” (QOT) needed to procure and maintain adequate tissue oxygenation. Consider two hypothetical 6-month-old infants, Destiny and Dakota, 2 hours after repair of tetralogy of Fallot. They have identical (and adequate) DO2 and perfusion pressure, but Destiny has a left atrial (LA) pressure of 6 mm Hg and a right atrial (RA) pressure of 8 mm Hg, whereas Dakota has received volume infusion to achieve an LA pressure of 6 mm Hg and an RA pressure of 15 mm Hg. Assuming that the levels of intravascular volume provided are exactly those needed to achieve the (identical) DO2 values and perfusion pressures, it is clear that the physiologies of these two patients are different. The clinician who has learned what to expect relative to the QOT in any given set of circumstances will find Dakota’s sufficient tissue oxygenation only mildly reassuring and asks: Is there substantial residual right ventricular (RV) outflow tract obstruction or other problem(s) that I need to know about? Will Dakota have sufficient DO2 in 10 hours when post-bypass myocardial depression is at its worst? Might additional therapy secure adequate DO2 at a lower filling pressure, thereby minimizing adverse effects of systemic venous hypertension? As this example illustrates, the QOT concept is useful for three reasons:
Almost any form of therapy might be included in the QOT concept, but we will focus on medical therapies that have the most important effect on hemodynamics, including DO2 and perfusion pressure. These therapies include inotropic/vasoactive agents, volume infusion, and airway pressures used during mechanical ventilation. The amount of inotropic and vasoactive drugs administered, assuming that they are used appropriately, seems to be a crude indicator of patient illness.2 Volume infusion to achieve adequate filling pressure is required even for the normal heart, but the QOT concept applies when higher than normal filling pressure is needed to maintain adequate CO. The consequences of high filling pressures are body edema (especially in infants), pleural and other cavity space effusions, and pulmonary edema. If high venous pressure is coupled with systemic hypotension (e.g., with a “failing” Fontan circulation), there may be critically reduced trans-tissue perfusion pressure with a potentially negative impact on cerebral and splanchnic perfusion.
Variables that Determine Tissue Oxygenation
where CO is cardiac output or SBF in L/min or L/min/m2 and CaO2 is quantity of O2 bound to hemoglobin plus the quantity of O2 dissolved in the plasma in arterial blood. The O2 content of arterial blood (mL O2/dl blood) equals:
where SaO2 is arterial O2 saturation, Hgb is hemoglobin concentration (g/dL), 1.36 (constant) is the amount of O2 bound per gram of hemoglobin (mL) at 1 atm of pressure, PaO2 is arterial partial pressure of O2, and 0.003 (constant) multiplied by the PaO2 equals amount of O2 dissolved in plasma at 1 atm. The quantity of dissolved O2 is generally considered to be negligible in the normal range of PaO2. Hypoxia, because of poor gas exchange within the lungs (i.e., intrapulmonary shunt), or in the setting of CHD with right-to-left shunting, is an important determinant of blood O2 content.
Monitoring Tissue Oxygenation
CO can be assessed qualitatively by physical examination and other modalities and quantitatively by a variety of techniques using invasive and noninvasive devices, laboratory data, and other clinical indicators. DO2 is easily derived if systemic blood flow can be measured, but it is only indirectly inferred if this information is lacking.
Quantitative Assessment of Cardiac Output
Thermodilution Technique
where Vi is injectate volume (mL), Tb is temperature of blood, Ti is injectate temperature, and Tb(t)dt is area under the curve. In general, thermodilution CO measures are performed using a completely automated system, and the calculations are performed by a computer. This method (as with any indicator dilution method) requires complete mixing and thus is most accurate in situations where a mixing chamber is located proximal to the thermistor. It is generally used only in patients who do not have intracardiac or great vessel–level shunts or an insufficient valve between the injection site and the sampling site. The injection must be made rapidly because a slow injection will give a falsely elevated CO. Possible sources of error with this method include inaccurate measurement of the volume of injectate or of the temperature of the blood or injectate, close approximation of the thermistor to a vessel wall, and inadequate mixing, as is sometimes seen in venous systems with low flow.
Fick Method
where VO2 is O2 consumption (mL/min) and CaO2 and CvO2 are arterial and venous O2 content (mL O2/100 mL blood), respectively. Care must be taken to select the appropriate sampling site for a true mixed venous blood sample. With a normal heart, the best site to obtain a mixed venous sample is within the PA. If a left-to-right shunt is present, however, the mixed venous site should be the cardiac chamber proximal to the site of the shunt. When a site other than the PA is used for the mixed venous site, the resultant value for arteriovenous O2 difference is a less reliable reflection of the absolute CO, but it can be used for serial observations and for monitoring response to therapy over time.
Because measuring VO2 in the intensive care setting requires special equipment and is somewhat cumbersome, the arteriovenous O2 difference is often used as an indirect measure of CO. A wide arteriovenous O2 difference generally reflects a low CO and indicates a large O2 extraction by the tissues, whereas a narrow arteriovenous O2 difference usually reflects a high CO. Unfortunately, studies suggest VO2 is quite variable for any individual patient in an intensive care setting.9 Furthermore, mixed venous O2 saturation (and, hence, arteriovenous O2 difference) may be misleading in patients with decreased tissue O2 extraction.10,11
Doppler Echocardiography
where A is the area of the orifice, V is integrated flow velocity, and HR is heart rate. To determine the integrated flow velocity, the area under the Doppler curve must be measured. The area of the aortic orifice is commonly obtained by measuring the aortic diameter from the two-dimensional image, where A = 0.785 ∞ d2. This technique requires special care for accurate Doppler interrogation of blood flow and is seldom used in the critical care unit.

Full access? Get Clinical Tree
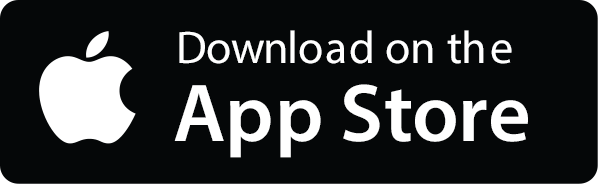
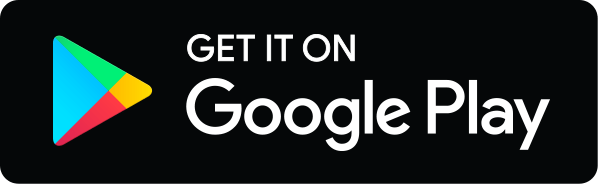