Fig. 49.1
(a) Humeral osteotomy: a section of humerus is removed and the remaining segments fixated (shown here with fixation screws), shortening the transhumeral limb to make room for a prosthetic elbow joint, yet retaining the epicondyles for improved socket suspension and rotational control. (b) Angulation osteotomy: a section of the distal humerus is fixated at an angle (with a plate as illustrated, or with a fixation screw). (c) Transhumeral socket with much lower proximal trimlines on the left is for a patient with angulation osteotomy, compared to traditional transhumeral socket with high trimlines and anterior and posterior flares for rotational control shown on the right
Recent advances in limb reconstruction for military casualties with complex injuries have also improved outcomes for prosthetic use. Limb reconstruction techniques that fix proximal fractures specifically to conserve length have allowed for longer residual limbs with better soft tissue coverage. Furthermore, advances in skin grafting techniques have improved salvage of longer residual limbs. This is particularly important for the upper limb where partial thickness grafts with less durability can tolerate socket fittings due to advanced interface materials and new socket technology.
Socket Technology
Modern prosthetic limbs utilize a rigid thermoplastic or laminated (resin impregnated carbon and nyglass) socket that surrounds the residual limb and distributes the mechanical loads through the soft tissue to the underlying bone. Because of this, the soft tissue must bear the stress of serving as a structural interface between the prosthesis and the remaining bone. The prosthetic socket is held in place on the limb through a combination of suction, silicone sleeves, and/or harnesses. While the construction of the socket has improved with modern materials such as thermoplastics and carbon fiber, the basic idea of the socket has remained essentially unchanged since the earliest documented advent of prosthetic limbs in 300 BC and perhaps as early as the ninth century BC [1, 2].
The socket is the foundation of the prosthesis and if it does not fit appropriately the wearer will have comfort issues, skin breakdown, suspension loss, and ultimately they will reject the prosthesis. Traditionally, the socket is made by taking an impression of the amputee’s residual limb (negative model) with Plaster of Paris or fiberglass casting material. Then a positive model of the residuum is made and modified, creating voids or areas of relief for sensitive areas, to serve as a base to form the socket. Recently, digital laser scanning approaches have been developed that reduce the need for plaster casting. The scanner creates a 3-d image of the limb for the prosthetist to manipulate using software. Care is taken to highlight areas that can withstand more pressure, usually muscle tissue, and areas that cannot, such as bony prominences, scar tissue, and neuromas. The load tolerant areas are compressed in order to gain suspension and reduce excess movement between the socket and the residuum. If this is done digitally, the resulting computer file is then uploaded to a robotic carving machine that produces a blank of the residual limb from rigid polyurethane foam.
Modern socket designs rely on total contact with the limb while accommodating for load tolerant or intolerant areas as described above. By loading on a majority of the soft tissue surface area, the limb weight is transferred more evenly throughout the socket. However, as the bony residuum is not directly secured to the prosthesis, rotation and movement of the limb in relationship to the socket can cause loss of socket fit or poor suspension. These problems are magnified with limb atrophy, changes in volume, or physical load on the limb. High-compression socket designs came about to reduce the amount of socket motion that may occur within the prosthesis. When motion is eliminated and better suspension is achieved, the prosthesis feels “a part of the patient.” The weight of the device feels less and the patient will likely tolerate wearing the device for longer amounts of time. The more the device is used and incorporated into the patient’s activities of daily living, the more successful the prosthesis is deemed.
In order to maximize comfort and function with the development of new highly functional prosthetic limbs, the systems that attach the devices to the amputated limb must evolve. New high-compression or compression/release-stabilized sockets squeeze the soft tissue of the limb through longitudinal depressions or bands that compress and displace the tissue between the residual bone and the socket to reduce motion. In effect, tolerant areas are loaded aggressively with alternating relief areas to provide the patient more comfort and control. Care must be taken with this design to ensure that compression is maintained at a safe level for the patient. One design incorporates a blood volume perfusion sensor to ensure that there is adequate/safe blood flow to the residual limb.
In addition to new compression type approaches, dynamic systems that are able to adjust to the amputee over the course of the day are beginning to be realized. A recent advanced shoulder disarticulation prosthetic system utilized inflatable socket bladders that could be adjusted to increase stability under loaded conditions, account for changes in volume, and aid donning and doffing [4]. The pneumatic inflation and deflation of the individual bladders of the system were dynamically controlled by a belt-worn unit. This type of adjustability within the socket system could potentially address concerns with fluctuations in socket fit and comfort throughout the day.
Prosthetic Suspension
The rigid prosthetic socket must be suspended from the residual limb in order to functionally approximate the lost limb. Multiple modes of suspension are available for upper extremity sockets and therefore residual limb quality, patient tolerances, and prosthetist preference influence the final design choices.
Harness suspensions utilize a series of straps and buckles that attach to the socket and anchor to the trunk and contralateral limb. The straps of the harness are typically routed in the form of a figure eight across the back and this type of suspension is most commonly used in conventional body-powered style prosthetic limbs (Fig. 49.2). The harness provides both an anchor for the socket and an attachment for cables to gain excursion to operate a terminal device.
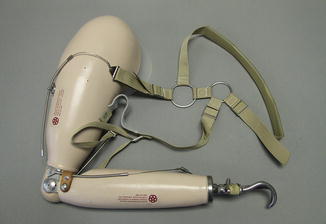
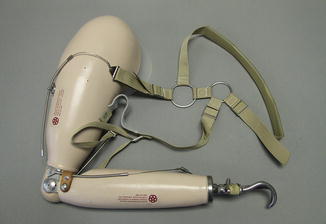
Fig. 49.2
A traditional body-powered transhumeral socket, with a “Figure of 8” harness system used for suspension and cable control of the terminal device and elbow
A suction fit socket attaches directly over the amputee’s residual limb. An intimate airtight fit retains adhesion to the residual limb by using a one-way expulsion valve that is built into the socket. This negative pressure within the socket provides a stable attachment and prevents the limb from slipping off during use.
Silicone suction liners provide a mechanical connection to the socket by “gripping” the residual limb of the amputee (Fig. 49.3). The advent of the silicone suction socket liner in the late 1980s revolutionized the fit of prosthetic limbs [5]. The use of a gel liner against the skin greatly reduces the shear forces transferred to the residual limb in comparison to the earlier leather liner and prosthetic sock system. This has resulted in much more comfortable prosthetic limbs, better skin management, and less problematic fitting issues. Silicone and related polyurethanes are excellent liner materials. They are more comfortable next to the skin than most materials. They are also compliant, strong, and maintain an airtight seal in both suction and traction applications. One particular advantage of these liners is that they provide comfort by creating a soft barrier between the hard socket and sensitive or scarred skin and bony prominences. These liners can also effectively have numerous adjunct suspension mechanisms incorporated into their design such as locking pins, valves, or lanyards.
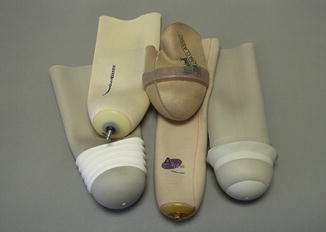
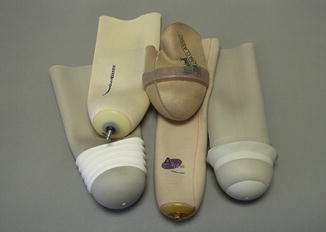
Fig. 49.3
Examples of liners typically used as an interface between the residual limb and the hard socket. Suspension can be provided by a pin affixed to the bottom of the liner matched to a shuttle lock in the socket, or by various ring seals around the outside of the liner to form a vacuum seal
Self-suspending sockets interconnect with the remaining bony anatomy of the residual limb through contours built into the socket. For example, these sockets can be used to apply medial and lateral pressure over the humeral epicondyles in transradial level amputation. In this type of fitting, the socket terminates proximal to the epicondyles to create a bony lock or anchor that helps reduce soft tissue movement and enhances suspension.
Other Attachment Strategies: Osseointegration
One strategy that has been used to overcome the above-mentioned socket limitations is direct skeletal attachment, or osseointegration. This technique for prosthetic limb attachment was pioneered in Sweden in the 1950s, with the first clinical patients treated in the 1990s [6]. This is now an accepted treatment technique in several European centers. In this approach the use of a rigid socket is eliminated entirely by attaching the prosthesis to a metal abutment that passes through the skin and is anchored within the residual limb bone. This type of approach has been used successfully for many years in dental implants, facial reconstructions, and prosthetic digits. In the modern implementation, a titanium fixture is placed down the center of the amputated limb bone and the terminal bone encapsulates the device. This bone anchored attachment then accepts a replaceable titanium abutment that transverses the skin. At the point where the percutaneous abutment traverses the skin, the skin is encouraged to adhere to the bone in a junction that is similar in rigidity to the base of the fingernail. This prevents breakdown at the percutaneous junction. The distal end of the abutment serves as the mounting point for a prosthetic limb. This type of attachment is highly robust and able to bear considerable forces beyond simple weight bearing. The main persisting complication is that of intermittent superficial skin infections that can occur at the percutaneous junction [7].
The primary advantage of osseointegration is that the weight and functional leverage of the prosthetic limb is transferred directly to the skeleton. This direct bone attachment approach has recently been applied in upper limb prosthetics with high success in individuals who have highly demanding physical occupations. There has also been described a phenomenon of “osseoperception,” whereby the osseointegrated fixture is able to supply tactile or proprioceptive feedback to the individual through vibrations transmitted to the direct skeletal attachment. One of the exciting recent developments in transdermal bone-integrated upper limbs is the inclusion of an internally routed feedback and control system that will be discussed in more detail later in this chapter.
Prosthetic Power and Control Strategies
There are three current control strategies for most standard-of-care functional (active) prosthetic limbs: body-powered, externally powered (myoelectric), and hybrid design. Body-powered devices mechanically harness the movement of proximal joints through a single or dual cable linkage to actuate the joints of the prosthesis. These designs reached their zenith after World War II and were based on cable and housing technology that originally came from bicycle brakes and aircraft control systems. Body-powered limb systems with a cable-actuated split hook are highly functional and are still employed widely in the clinic today. These devices are simple, intuitive to operate, robust, and interestingly, they provide a high level of useful position and movement feedback that can be felt through the excursion of the cables.
The joints of myoelectric devices are driven by battery powered motors. These systems are controlled by electrodes that are placed on the skin to read and amplify the EMG signals that are generated from volitional contraction of the underlying muscles. These systems typically utilize preserved antagonistic muscle groups to operate up to 3 degrees of freedom: hand open/close, wrist rotation, and elbow flexion/extension. The movement at each joint is sequentially operated and the individual functions are selected by a switch strategy, for example co-contracting the muscle pairs, or pushing an externally mounted electric switch.
Hybrid prosthetic limbs combine both power and cable control systems. For instance a typical setup will utilize myoelectric componentry for the hand and wrist, with elbow function operated by body-powered cable actuation. This approach simplifies the control strategy for the user and often makes the prosthesis less heavy than a full myoelectric system, and therefore more tolerable to wear for extended use.
Terminal Device Advancements
The human hand is a sophisticated anatomical structure that is highly dexterous. It is used to manipulate a variety of objects, to interact with our environment and even to communicate. There have been limited prosthetic options for replacing even a fraction of the functionality of a human hand. However, one of the most successful and functional devices is the common body-powered hook (Fig. 49.4). This replacement for the hand provides a fine “tip-to-tip” pinch, or a canted “lateral key pinch” grip. Unfortunately, the primary drawback of this simple, robust, and highly dexterous device is its aesthetic appearance.