Fig. 26.1
The HeartMate II® (Thoratec Corp., Pleasanton, CA) is currently the device of choice for cardiac assist applications worldwide
The HeartMate II contains a rotor capable of producing flow rates greater than 10 L/min at resolutions ranging from 8,000 to 15,000 rpm [5]. The blood pump itself is made of titanium with a ruby bearing; the straight pump tube is 12 mm in diameter and contains the inlet stator, pump rotor, and outlet stator. The stators are fixed components that form the pivots of the rotor. The rotor contains a magnet, and a magnetic field is generated between the rotor and the motor, which results in rotary motion and torque leading to blood flow. Blood from the LV’s apex is carried to the inflow conduit via the inlet cannula. Blood enters the outflow graft (and then the ascending aorta) via the outflow elbow. The inner surfaces of the inflow elbow, inlet cannula, and outflow elbow contain a textured surface to stimulate a natural endothelial surface for blood flow. When blood enters the HeartMate II, it passes through the inlet stator, where the course that the blood follows is straightened out via three guide vanes. The LVAD rotor’s three curving blades impart kinetic energy to the blood while also generating a radial velocity to the blood flow. Blood flow exits the pump rotor through the outlet stator, which contains three vanes with a twisted shape that convert the radial velocity of the blood flow to an axial velocity [9]. As in other axial-flow pumps, there is a risk of the pump’s generating negative intraventricular pressure, causing the ventricle to collapse. As a result, inflow cannula positioning and ventricular preload are important. In the HeartMate II, the intraventricular portion of the inflow tract has been elongated and, as a result, tends to stent open the middle of the ventricle, thus improving reliability of CF throughout the cardiac cycle [5, 7].
Anticoagulation medication is, at present, recommended to prevent thrombosis by keeping the international normalized ratio of the blood between 2.0 and 2.5. The HeartMate II is placed either above the peritoneum or above the posterior rectus sheath in the left upper quadrant below the diaphragm. Power and control are delivered through a percutaneous cable that is attached to a controller.
A multicenter randomized trial (conducted at 38 US centers) reported results from the CF HeartMate II (n = 133) and the pulsatile HeartMate XVE (n = 59) implanted in almost 200 patients with similar baseline characteristics and DT risk scores [10]. The median duration of support was 1.7 years (range, 0.0–3.7) for the HeartMate II and 0.6 years (range, 0.0–2.1) for the pulsatile XVE, with a cumulative follow-up of 211 and 41 patient-years, correspondingly. All patients were followed up for at least 2 years, or until death, transplant, or device explant. The primary endpoint was a composite survival at 2 years, free of disabling stroke or reoperation to replace the device. The HeartMate II group was superior to the pulsatile device in terms of survival, with estimated 1- and 2-year survival rates of 68 and 58 %.
The functional class and quality of life in BTT (n = 281) and DT (n = 374) patients have been assessed at baseline and post-implant [11]. Post-implant, 82 % (BTT) and 80 % (DT) of patients at 6 months and 79 % (DT) at 24 months improved to NYHA functional class I or II. Mean 6-min walk distance of 204 m (DT) was improved to 350 and 360 m at 6 and 24 months.
Despite the improvements in survival since the approval of the HeartMate II for BTT and DT, there are still adverse effects associated with device use that must be considered [7]. The risk of pump thrombosis and thromboembolism is the biggest concern with the use of CF rotary pumps. An apparent increase in the rate of device thrombosis among patients who had received the HeartMate II, with substantial morbidity and mortality, has recently been observed [12]; this setback makes the search for the causes of thrombosis mandatory so that physicians can predict and prevent this daunting complication. Data from a multicenter clinical trial in 895 patients revealed 72 confirmed pump thromboses (in 66 patients), with 36 incidences of thrombosis. The estimated occurrence of thrombosis in the HeartMate II was reported to be 4.7 %, 7.5 %, and 12.3 %, correspondingly at 6, 12, and 24 months of support [13]. The causes of thrombosis seem multifactorial and yet remain uncertain (among the possibilities are hypercoagulability leading to thrombus deposition, hemodynamic compromise, flow reduction, heat dissipation from the bearing, inadequate anticoagulation, aortic regurgitation, hypovolemia, kinking of the outflow graft, or inflow cannula malposition). Therefore, careful recommendations for LVAD therapy should account for each patient’s risk-benefit profile. Early perioperative pump power elevations can occur frequently, and this observation has been concerning; however, the relationship between early power elevations and subsequent development of stroke and pump thrombosis has yet to be defined [14].
HVAD®
The HVAD® (HeartWare International, Framingham, MA) CF pump weighs 160 g and has a displacement volume of 50 mL and an external diameter of 53 mm (Fig. 26.2). The HVAD operates within the range of 1,800–4,000 rpm and can pump up to 10 L/min of blood. Within its titanium pump housing, the HVAD has a unique wide-blade impeller (a single moving component), suspended by hybrid magnetic/hydrodynamic forces for frictionless rotation [15]. There are no points of mechanical contact within the pump, effectively ensuring a “wearless” system. The design integrates two motor stators for single-motor fault protection to increase reliability. An integrated inflow cannula is inserted into the LV and is held in position by a sewing ring; the pump is positioned in the pericardial space. The 10-mm outflow graft is anastomosed to the ascending aorta. Communication from the pump to the external power and control components is provided by a flexible, polyurethane-covered driveline that is externalized through the abdominal wall. A microprocessor-based controller monitors pump function and regulates power to dual motors within the pump. The controller displays pump speed, blood flow rate, power, alarm conditions, and instructions for resolving such conditions. A continuous power supply is provided by either alternating or direct current (AC or DC) sources, including lithium-ion batteries for portable operation. A system monitor provides a means for adjusting pump operating parameters, monitoring pump function, and storing data. External system components include the microprocessor-based controller, a monitor, lithium-ion battery packs, AC and DC power adapters, and a battery charger. Physiologic control algorithms are incorporated for safe operation.
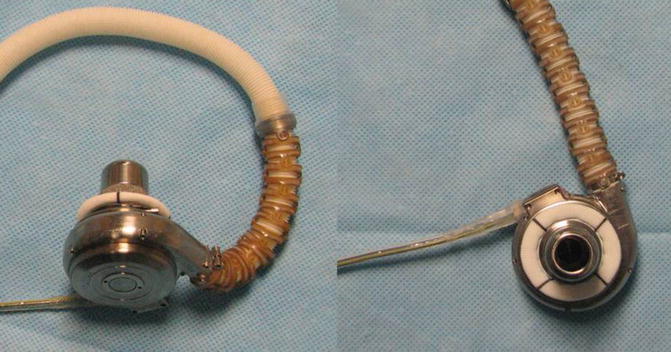
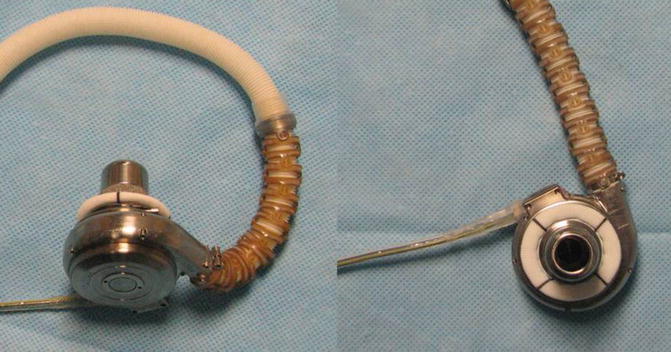
Fig. 26.2
The HVAD® (HeartWare International, Framingham, MA) pump
The biventricular use of two HVADs has been described, but is as yet an “off-label” use. In such cases, the investigators have cautioned that even the lowest deliverable device flow (2,400 rpm) would still be too high in the pulmonary circulation without a systemic afterload and that, theoretically, use of the device would result in pulmonary edema [16]. Therefore, additional modifications, such as narrowing of the outflow graft, may be necessary to increase the afterload when the HVAD is used for right ventricular (RV) assist. The optimal anatomic sites for device insertion have yet to be established.
A large multicenter study (30 US centers) presented post-implant survival in 322 patients with the HVAD [17]. Patients were monitored for 180 days or more, or until heart transplantation or death. The study revealed survival rates at 60, 180, and 360 days as 97 %, 91 %, and 84 %, respectively. The study showed that 67.1 % of patients were alive 180 days after the HVAD was originally implanted, and 21.4 % had either received a donor heart or the device had been explanted during recovery within 6 months post-implant. Survival of patients in these latter groups (transplant or explant) was 90 % at 180 days and 89 % at 360 days. A smaller multicenter HVAD trial conducted in Europe and Australia reported an actuarial survival of 91 % at 6 months and 86 % at 12 months [18]. All pumps were implanted intrapericardially. From a total of 23 patients, at 1-year follow-up, a mean duration of support of 305 ± 105 days and total support of 19.2 patient-years have been reported.
Recently, the analysis of outcomes in 382 patients who underwent an HVAD implantation as part of a HeartWare BTT trial reported that pump thromboses were observed in 8.1 % (34 thrombotic events) of patients (n = 31) [19]. These events occurred at an average of about 1 year (353 ± 329 days) after implant. The freedom from any pump thrombosis was 98 %, 96 %, and 92 %, respectively, at 3, 6, and 12 months. The investigators found no statistically significant difference (p = 0.21) in survival at 1 year between the groups with thrombus (85.4 %) and without thrombus (69.4 %). Unexpected power increases and flow estimation beyond that predicted from power consumption were observed. Fibrin or clot on the surface of the impeller may have caused an increase in power consumption, resulting in a decrease in pump efficiency. Depending on the severity and formation characteristics of the thrombus, an increased trend and rapid rise in LVAD power consumption were noted.
Jarvik 2000
The Jarvik 2000 FlowMaker® LVAD (Jarvik Heart, Inc., New York, NY) is a compact axial-flow pump (Fig. 26.3) that provides continuous blood flow from the LV to the descending or ascending aorta [20]. The device for adults measures 2.6 cm in diameter by 7.8 cm in length and weighs 85 g (90 g with a pre-attached 16-mm Hemashield outflow graft and small-bore percutaneous lead) and is about the size of a common C-cell battery. The pump has a volume of 30 mL and a priming volume of 7 mL [21]. The apical cuffed sewing ring secures the pump in place. The Jarvik 2000 is the only blood pump to be placed within the native heart’s ventricle, and thus no inflow cannula is required. The blood-contacting surfaces within the pump are made of smooth titanium. The pump comprises a single rotating impeller, located in the center of the titanium housing. A brushless DC motor, also positioned within the housing, creates the electromagnetic force necessary to rotate the impeller. The impeller consists of a neodymium-iron-boron magnet and two titanium impeller blades that are suspended by ceramic cone bearings. Blood is directed through the outflow graft by stator blades located near the pump outlet. In addition to the blood pump, the Jarvik 2000 system comprises a 16-mm outflow graft, percutaneous power cable, speed controller, and DC power supply. The external speed controller powers the pump via a series of wires contained in a silicone tube, which is covered with Dacron. The pump can be operated at 8,000–12,000 rpm and can generate an average flow rate of 3–7 L/min at 4–8 W of power under optimal conditions [22].
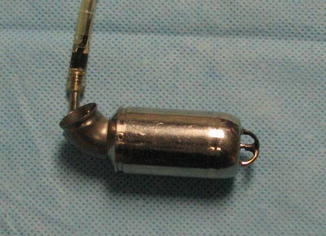
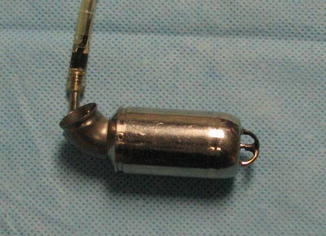
Fig. 26.3
The Jarvik 2000 FlowMaker® (Jarvik Heart, Inc., New York) axial continuous-flow pump. The device is inserted into the LV apex
Two smaller versions of the device are in development for pediatric patients. Specifications for the child and infant pumps are, respectively, as follows: diameter, 17.5 and 10.5 mm; length, 5.2 and 3.2 mm; displacement volume, 10 and 3 mL; and flow 1.0–1.5 L/min and 0.25–0.75 L/min [21].
The simplified configuration of the Jarvik 2000 makes an off-pump implantation feasible. The ability of the pump to be inserted through a thoracotomy has been reported as one of the key surgical advantages in patients who have not had previous cardiac surgery [23]. However, the difficulty of explanting the device implanted through a left thoracotomy may become very challenging after a median sternotomy at the time of transplantation.
Several trials have reported the safety and effectiveness of Jarvik 2000 for HF patients, both as a BTT and as DT. The device has been associated with a low incidence of thrombosis [24, 25], which is believed to be a result of the high-flow blood stream that washes the miniature bearings of the device [22].
New Devices and Concepts
MVAD®
HeartWare (HeartWare International, Framingham, MA) has in preclinical testing a next-generation device, the MVAD, a wide-blade axial CF pump (Fig. 26.4). The MVAD is approximately half the size of the HVAD, with 22 mL of displacement volume and 5 mL of priming volume. The MVAD is designed for versatility: it can be used as partial or full support for left, right, or biventricular applications, via either sternotomy or thoracotomy. The MVAD features a magnetic axial rotor and uses a combination of passive permanent magnets and hydrodynamic bearing surfaces to totally suspend the rotor in operation, with a displacement volume of only 15 mL. This is accomplished by a unique rotor with impeller blades that are wider than the blood flow channel between them, affording sufficient surface area for passive hydrodynamic radial rotor suspension. This design eliminates the mechanical pivot bearings typical of axial pumps and significantly reduces pump size by using passive rotor suspension rather than the larger and more complex active electromagnetic bearing designs. Also not typical of axial pump design is an outflow port, angled 90° from the pump housing inlet port, similar to centrifugal pump designs [26]. Various versions of the MVAD are being tested, implanted within the LV or at the LV apex, in animal studies (Fig. 26.5). The MVAD is currently not available for clinical application, but a clinical trial is planned soon for this investigational device.
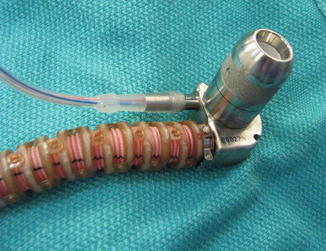
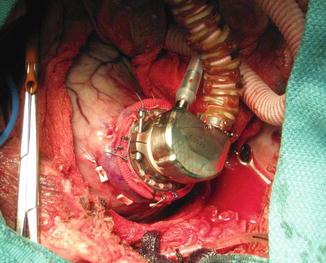
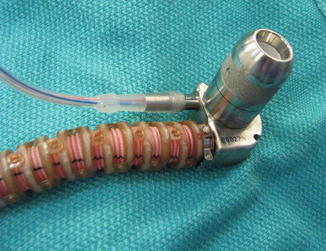
Fig. 26.4
Miniature ventricular assist device (MVAD®; HeartWare International, Framingham, MA). The inflow cannula, the largest portion of the device, is placed completely inside the ventricle
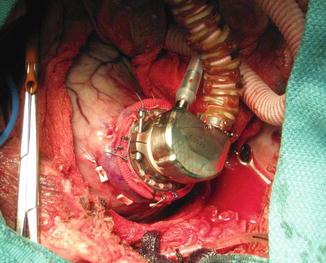
Fig. 26.5
The MVAD® (HeartWare International, Framingham, MA). The extremely small and low-profile pump design may enable its implantation using a minimally invasive approach
HeartMate III™
The HeartMate III (Thoratec Corp., Pleasanton, CA) centrifugal CF rotary pump (Fig. 26.6) is now in preparation for clinical testing and Conformité Européenne mark clinical trials (expected to start by the last quarter of 2014). The pump incorporates many critical family design elements of the HeartMate II (e.g., large gaps and textured blood-contacting surfaces) and has been designed to reduce the potential for adverse events (aortic insufficiency, bleeding, thrombus, and stroke). Its large gaps also enable rapid speed changes used by the artificial pulse feature without rotor and/or housing contact. The HeartMate III addresses many of the limitations of axial pump design: it features a bearingless magnetic levitation of the pump rotor (rather than mechanical pivot bearings) to increase reliability out to 10 years, has an accurate sensorless flow estimator, and incorporates a pulse mode to increase the level of pulsatility and even produce physiological pulse pressures. This new pump may potentially reduce the need for anticoagulation agents. Wedging surfaces and other features required for hydrodynamic bearings are not required. The device’s rotor and volute have been designed to minimize shear and avoid stasis over the entire range of operation (2–10 L/min). Rotor levitation is independent of rotor speed; levitation is maintained at any rotor speed, even zero. Rotor speed independence permits flexibility in operating speeds, which could in the future enable use of the HeartMate III under low-speed conditions, e.g., pulmonary circulation and weaning protocols. These features make it feasible to implement physiologic control algorithms based on accurate flow estimates. In addition, centrifugal pumps have characteristics that generate lower pressures over a wider flow range. This capability translates to more favorable outcomes owing to much lower inlet suction at low flows; lower outlet pressures at high levels of systemic vascular resistance, preventing the creation of exceedingly high systemic arterial pressures; higher flow pulsatility that is more sensitive to LV pressure swings, providing both a more sensitive pre-suction detection capability and increased diagnostic feedback about the functional status of the LV; and safer chronic management of pump speed and output optimization. These features suggest that centrifugal rotary pumps may overtake axial-flow rotary pumps in the CF rotary pump revolution that has made first-generation pulsatile pumps all but obsolete [7].
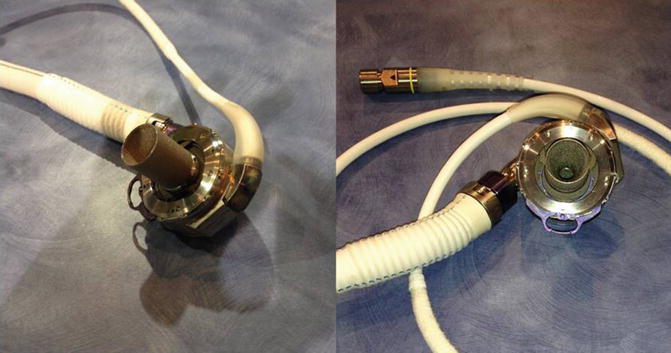
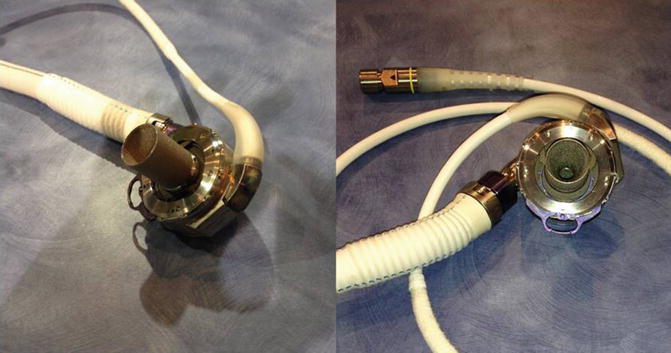
Fig. 26.6
The new centrifugal HeartMate III™ (Thoratec Corp, Pleasanton, CA) device. The inflow cannula design (concave) is similar to the one used in its predecessor
HeartMate X
The HeartMate X (Thoratec Corp., Pleasanton, CA), a highly energy-efficient axial-flow pump, leverages core HeartMate II bearing technology with a dramatic size reduction for rapid, less invasive implantation. The HeartMate X offers versatile cannulation options and, in this ultra-compact dimension, is considered suitable for partial to full ventricular support configurations for patients needing LVADs as well as the populations needing right or bi-ventricular assist devices (RVADs or BiVADs) [27]. The device can provide a stable 8 L/min of CF. The HeartMate X may thus further expand potential approaches for truly minimally invasive implantation techniques, especially in small size and younger patient populations.
SYNERGY®
In 2013, HeartWare International announced that it had acquired CircuLite®, Inc. (Saddle Brook, NJ), developer of the SYNERGY® Circulatory Support System, a “micro-pump” designed to treat less sick, ambulatory patients with chronic HF who are not yet inotrope dependent. The CircuLite Synergy LVAD (HeartWare) is a novel pump that combines axial, centrifugal, and orthogonal flow paths at a 7.5 mL volume and operating speeds of 20,000–28,000 rpm. The device is the size of a double-A battery, connects to the heart via an inflow cannula (8 mm diameter by 20 cm long), has a pumping capacity of up to 4.25 L/min, and is small enough to be placed in a subcutaneous pocket. The blood passes through narrow channels around the motor (which serves to cool the motor) and leaves the pump at a right angle to the inlet [28]. It uses a magnetically and hydrodynamically stabilized rotor design. The Synergy’s pump body is implanted via a small right-sided thoracotomy into a subcutaneous pacemaker-like pocket in the right subclavian groove. It is implanted without the use of extracorporeal circulation, with the inflow cannula placed into the left atrium and the outflow graft connected to the right subclavian artery. The device has been implanted in 25 patients on the waiting list for a heart transplant as a partial support device. With flows recorded between 2.3 and 3.5 L/min, the applications of the pump may be extended for support in less sick patients (those considered in New York Heart Association functional class III) [29].
Although clinical experience is currently limited to pumping blood from the left atrium to the subclavian artery, recent cadaveric research has indicated that this micropump can also be easily positioned as an RVAD.
The SYNERGY® Circulatory Support System is currently undergoing a redesigning process related to such issues as device thrombosis and mechanical fractures of the inflow cannula portion. The additional device development and optimization will be critical to provide proper pump reliability and function. However, the US clinical trial that has been put on the company’s agenda will most likely be further delayed.
Future Perspectives
Options for RVAD/BiVAD
The application of CF LVADs for refractory end-stage HF is increasing. When LV filling remains inadequate despite pharmacotherapy to enhance RV function and lower pulmonary vascular resistance, an RVAD may be required [20, 30]. The primary reason VADs have not been designed specifically for RVAD applications is that there is much less clinical need (and therefore a limited market) compared with that for LVADs [7, 30]. Although clinically approved implantable LVADs are not designed for right-sided support, LVAD implantation for right heart support is being considered simply because an effective, easily implantable RVAD is not yet available. The absence of a dedicated, clinically evaluated, long-term RVAD significantly diminishes the treatment options for patients with isolated and post-LVAD RV failure, as well as for those with biventricular HF requiring two pumps (BiVADs) [31–33]. The inherent CF pump mechanics and translation of clinical features, pump selection, and patient management issues have previously been detailed [26].
The first use of an implantable CF pump for RV support was reported in 2004; a 21-year-old patient with worsened RV failure and elevated peripheral vascular resistance after implantation of a Jarvik 2000 FlowMaker was treated by implanting a second one [20]. By directing the inlet toward the inferior vena caval-right atrial junction and keeping right atrial pressures at 5–10 cm H2O, surgeons achieved stable RV unloading and satisfactory blood flow to the left-sided pump. Other groups consecutively reported on limited, off-label, clinical use of two LVADs. Saito et al. [34] converted a 6-month survival patient from a Toyobo paracorporeal device (Toyobo-Nipro, Osaka, Japan) to a Jarvik 2000 as RVAD and a DuraHeart (Terumo Heart, Inc., Ann Arbor, MI) for LVAD support.
Successful application of two HVADs for treatment of severe biventricular failure was reported by Strueber et al. [15] and Hetzer and collaborators [35]. Additional case reports of intrapericardial implantation of the HVAD followed, the surgeons using a similar technique of placing the pump on the RV diaphragmatic surface just below the acute margin. Two additional silicone rings were placed under the fixation ring. Artificial restriction applied to the outlet graft of the pump may compensate for the lower outlet pressures seen at the pulmonary artery [36]. HeartWare is currently working to obtain regulatory approval for modifications to the HVAD for use as RVAD support [7]. Potapov et al. [37] from the German Heart Institute in Berlin reported on ten patients with idiopathic dilated cardiomyopathy patients who received biventricular HVADs. In three of these patients, physicians have been able to turn the RVAD off following long-term biventricular support, having confirmed via echocardiography that the patients had stable hemodynamics and that no thromboembolic events and no regurgitation through the right pump had occurred. Krabatsch et al. [38] reported implantation of an HVAD, first on the RV free wall at the maximal distance from the interventricular septum. Because severe compression of the RV caused low flow of the right pump of maximally 2 L/min, the RVAD was reimplanted to the right atrium through the layer of pericardium (opening created from the right pleura) and by using two silicone rings (to prevent the inflow cannula being introduced too deeply into the chamber). That group subsequently reported 17 patients implanted with two HVADs [16]. Deuse at al. [39] recently reported the HVAD use for permanent RV support with no spacers (rings) used.
Recently, Schmitto et al. [28] investigated the use of two Synergy Micro-Pumps (CircuLite Inc., Saddle Brook, NJ) for full biventricular support in ovine models. Such use may provide another option for partial (biventricular) support in patients and, as the authors stated, for full support in small adults and children.
Currently, temporary support via RVADs/BiVADs is available by CF extracorporeal or paracorporeal circulation support systems [30, 33] such as the CentriMag® (Thoratec Corp., Pleasanton, CA), the Bio-Pump (Medtronic, Minneapolis, MN), and the TandemHeart (CardiacAssist, Inc., Pittsburgh, PA). Progress in the development of clinically available rotary pumps now permits physicians to choose a hybrid approach for BiVAD placement, using both an implantable LVAD and temporary extracorporeal RVAD [33, 40, 41]. Since the first reported application of two Jarvik 2000 FlowMaker pumps [20], several groups have adopted this approach for biventricular assist. The adaptation of an LVAD to function as an RVAD is a technical challenge because of both ventricular distinctions and pump characteristics [30, 42].
RVAD design challenges involve pump dimensions and fit, as well as adaptability to operating flows. RVADs operate at much lower afterloads (pulmonary arterial pressures) than LVADs do and at significantly reduced hydraulic loads and power consumption rates. Because of its low-pressure working conditions and complex geometry, the RV stands in stark contrast to the LV. Under normal baseline conditions, the unique anatomy, myocardial ultrastructure, and coronary physiology of the RV reflect a high-volume, low-pressure pump. Once surgeons introduce an LVAD, they raise the expected output of the RV to that of the LVAD [43].

Full access? Get Clinical Tree
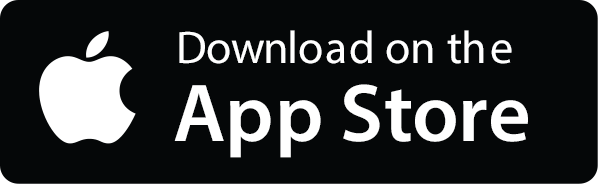
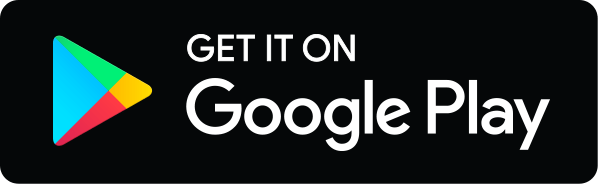