Arterial Line Placement and Care
Jason Lee-Llacer
Michael G. Seneff
Arterial catheterization remains an extremely important skill for critical care physicians. The most common indications for inserting an arterial catheter remain the need for close blood pressure monitoring and frequent blood gas sampling in unstable and ventilated patients. Newer technologies that necessitate arterial access continue to mature. For example, arterial pulse contour analysis can now be used to predict fluid responsiveness and compute cardiac output more reliably and less invasively in appropriately selected patients [1]. Although it is likely that advancements in current noninvasive technology, such as transcutaneous PCO2 monitoring and pulse oximetry, will decrease the need for arterial catheter placement, intensivists will always need to be knowledgeable in the setup and interpretation of arterial catheter systems. In this chapter, we review the principles of hemodynamic monitoring and discuss the indications, routes, and management of arterial cannulation.
Indications for Arterial Cannulation
Arterial catheters should be inserted only when they are specifically required and removed immediately when no longer needed. Too often they are left in place for convenience to allow easy access to blood sampling, which leads to increased laboratory testing and excessive diagnostic blood loss [2,3]. Protocols incorporating guidelines for arterial catheterization and alternative noninvasive monitoring, such as pulse oximetry and end tidal CO2, have realized significant improvements in resource utilization and cost savings, without impacting the quality of care [4].
The indications for arterial cannulation can be grouped into four broad categories (Table 3.1): (1) hemodynamic monitoring (blood pressure and/or cardiac output/pulse contour analysis); (2) frequent arterial blood gas sampling; (3) diagnostic or therapeutic/interventional radiology procedures, including intra-aortic balloon pump (IABP) use, arterial administration of drugs, vascular stenting and embolization, and (4) continuous cardiac output monitoring.
Noninvasive, indirect blood pressure measurements determined by auscultation of Korotkoff sounds distal to an occluding cuff (Riva–Rocci method) are generally accurate, although systolic readings are consistently lower compared to a simultaneous direct measurement. In hemodynamically unstable patients, however, indirect techniques may significantly underestimate blood pressure. Automated noninvasive blood pressure measurement devices can also be inaccurate, particularly in rapidly changing situations, at the extremes of blood pressure, and in patients with dysrhythmias [5]. For these reasons, direct blood pressure monitoring is usually required for unstable patients. Rapid beat-to-beat changes can easily be monitored and appropriate therapeutic modalities initiated, and variations in individual pressure waveforms may prove diagnostic. Waveform inspection can rapidly diagnose electrocardiogram lead disconnect, indicate the presence of aortic valve disease, help determine the effect of dysrhythmias on perfusion, and reveal the impact of the respiratory cycle on blood pressure (pulsus paradoxus). In addition, in mechanically ventilated patients, responsiveness to fluid boluses may be predicted by calculating the systolic pressure variation (SPV) or pulse pressure variation (PPV) from the arterial waveform, and stroke volume variation (SVV) from the pulse contour analysis. In patients on volume-controlled mechanical ventilation, all of these techniques have been shown to predict, with a high degree of accuracy, patients likely to respond (with an increase in stroke volume) to fluid volume challenge [1].
Recent advances allow continuous CO monitoring using arterial pulse contour analysis. This method relies on the assumption that the contour of the arterial pressure waveform is proportional to the stroke volume [6]. This, however, does not take into consideration the differing impedances among the arteries of individuals and different disease states and therefore requires calibration with another method of determining cardiac output [7]. This is usually done with lithium dilution or transpulmonary thermodilution methods. A different pulse contour analysis device has been introduced which does not require an additional method of determining CO for calibration, but instead estimates impedance based upon a proprietary formula that uses waveform and patient demographic data [7]. This method has significant limitations (i.e., atrial fibrillation) and there is concern that the device may not be accurate in clinical situations with dynamic changes in vascular tone (i.e., sepsis) [8]. Further data and comparison among the methods in authentic and diverse clinical situations are required before definitive recommendations can be made.
Management of complicated patients in critical care units typically requires multiple laboratory and arterial blood gas determinations. In these situations, arterial cannulation permits routine laboratory tests without multiple needle sticks and vessel trauma. In our opinion, an arterial catheter for blood gas determination should be placed when a patient requires two or more measurements daily.
Equipment, Monitoring, Techniques, and Sources of Error
The equipment necessary to display and measure an arterial waveform has not changed and includes (a) an appropriate intravascular catheter; (b) fluid-filled noncompliant tubing with stopcocks; (c) transducer; (d) a constant flush device; and (e) electronic monitoring equipment. Using this equipment, intravascular pressure changes are transmitted through the hydraulic (fluid-filled) elements to the transducer, which converts mechanical displacement into a proportional electrical signal.
The signal is amplified, processed, and displayed as a waveform by the monitor. Undistorted presentation of the arterial waveform is dependent on the performance of each component, and an understanding of potential problems that can interfere with overall fidelity of the system.
The signal is amplified, processed, and displayed as a waveform by the monitor. Undistorted presentation of the arterial waveform is dependent on the performance of each component, and an understanding of potential problems that can interfere with overall fidelity of the system.
Table 3.1 Indications for Arterial Cannulation | |
---|---|
|
The major problems inherent to pressure monitoring with a catheter system are inadequate dynamic response, improper zeroing and zero drift, and improper transducer/monitor calibration. Most physicians are aware of zeroing techniques but do not appreciate the importance of dynamic response in ensuring system fidelity. Catheter-tubing-transducer systems used for pressure monitoring can best be characterized as underdamped second-order dynamic systems with mechanical parameters of elasticity, mass, and friction [9]. Overall, the dynamic response of such a system is determined by its resonant frequency and damping coefficient (zeta). The resonant or natural frequency of a system is the frequency at which it oscillates when stimulated. When the frequency content of an input signal (i.e., pressure waveform) approaches the resonant frequency of a system, progressive amplification of the output signal occurs—a phenomenon known as ringing [10]. To ensure a flat frequency response (accurate recording across a spectrum of frequencies), the resonant frequency of a monitoring system should be at least five times higher than the highest frequency in the input signal [9]. Physiologic peripheral arterial waveforms have a fundamental frequency of 3 to 5 Hz and therefore the resonant frequency of a system used to monitor arterial pressure should ideally be greater than 20 Hz to avoid ringing and systolic overshoot.
The system component most likely to cause amplification of a pressure waveform is the hydraulic element. A good hydraulic system will have a resonant frequency between 10 and 20 Hz, which may overlap with arterial pressure frequencies. Thus amplification can occur, which may require damping to accurately reproduce the waveform [11].
The damping coefficient is a measure of how quickly an oscillating system comes to rest. A system with a high damping coefficient absorbs mechanical energy well (i.e., compliant tubing), causing a diminution in the transmitted waveform. Conversely, a system with a low damping coefficient results in underdamping and systolic overshoot. Damping coefficient and resonant frequency together determine the dynamic response of a recording system. If the resonant frequency of a system is less than 7.5 Hz, the pressure waveform will be distorted no matter what the damping coefficient. On the other hand, a resonant frequency of 24 Hz allows a range in the damping coefficient of 0.15 to 1.1 without resultant distortion of the pressure waveform [9].
Although there are other techniques [12], the easiest method to test the damping coefficient and resonant frequency of a monitoring system is the fast-flush test (also known as the square wave test). This is performed at the bedside by briefly opening and closing the continuous flush device, which produces a square wave displacement on the monitor followed by a return to baseline, usually after a few smaller oscillations (Fig. 3.1). Values for the damping coefficient and resonant frequency can be computed by printing the wave on graph paper [9], but visual inspection is usually adequate to ensure a proper frequency response. An optimum fast-flush test results in one undershoot followed by small overshoot, then settles to the patient’s waveform.
For peripheral pulse pressure monitoring, an adequate fast-flush test usually corresponds to a resonant frequency of 10 to 20 Hz coupled with a damping coefficient of 0.5 to 0.7. To ensure the continuing fidelity of a monitoring system, dynamic response validation by fast-flush test should be performed frequently: at least every 8 hours, with every significant change in patient hemodynamic status, after each opening of the system (zeroing, blood sampling, tubing change), and whenever the waveform appears damped [9].
With consideration of the above concepts, components of the monitoring system are designed to optimize the frequency response of the entire system. The 18- and 20-gauge catheters used to gain vascular access are not a major source of distortion but can become kinked or occluded by thrombus, resulting in overdamping of the system. Standard, noncompliant tubing is provided with most disposable transducer kits and should be as short as possible to minimize signal amplification [10]. Air bubbles in the tubing and connecting stopcocks are a notorious source of overdamping of the tracing and can be cleared by flushing through a stopcock. Currently available disposable transducers incorporate microchip technology, are very reliable, and have relatively high resonant frequencies [13]. The transducer is attached to the electronic monitoring equipment by a cable. Modern monitors have internal calibration, filter artifacts, and print the display on request. The digital readout display is usually an average of values over time and therefore does not accurately represent beat-to-beat variability. Monitors provide the capability to freeze a display with on-screen calibration to measure beat-to-beat differences in amplitude
precisely. This allows measurement of the effect of ectopic beats on blood pressure, PPV, SPV, or assessment of the severity of pulsus paradoxus.
precisely. This allows measurement of the effect of ectopic beats on blood pressure, PPV, SPV, or assessment of the severity of pulsus paradoxus.
When presented with pressure data or readings believed to be inaccurate, or which are significantly different from indirect readings, a few quick checks can ensure system accuracy. Improper zeroing of the system, because of either change in patient position or zero drift, is the single most important source of error. Zeroing can be checked by opening the transducer stopcock to air and aligning with the midaxillary line, confirming that the monitor displays zero. Zeroing should be repeated with patient position changes, (a transducer that is below the zero reference line will result in falsely high readings and vice versa), when significant changes in blood pressure occur, and routinely every 6 to 8 hours because of zero drift. Disposable pressure transducers incorporate semiconductor technology and are very small, yet rugged and reliable, and due to standardization, calibration of the system is not necessary [13]. Transducers are faulty on occasion, however, and calibration may be checked by attaching a mercury manometer to the stopcock and applying 100, 150, and/or 200 mm Hg pressure. A variation of ±5 mm Hg is acceptable. If calibration is questioned and the variation is out of range, or a manometer is not available for testing, the transducer should be replaced.
If zero referencing and calibration are correct, a fast-flush test will assess the system’s dynamic response. Overdamped tracings are usually caused by problems that are correctable, such as air bubbles, kinks, clot formation, overly compliant tubing, loose connections, a deflated pressure bag, or anatomical factors affecting the catheter. An underdamped tracing results in systolic overshoot and can be secondary to excessive tubing length or patient factors such as increased inotropic or chronotropic state. Many monitors can be adjusted to filter out frequencies above a certain limit, which can eliminate frequencies in the input signal causing ringing. However, this may also cause inaccurate readings if important frequencies are excluded.
Technique of Arterial Cannulation
Site Selection
Several factors are important in selecting the site for arterial cannulation. The ideal artery has extensive collateral circulation that will maintain the viability of distal tissues if thrombosis occurs. The site should be comfortable for the patient, accessible for nursing care and insertion, and close to the monitoring equipment. Sites involved by infection or disruption in the epidermal barrier should be avoided. Certain procedures, such as coronary artery bypass grafting, may dictate preference for one site over another. Larger arteries and catheters provide more accurate (central aortic) pressure measurements. Physicians should also be cognizant of differences in pulse contour recorded at different sites. As the pressure pulse wave travels outward from the aorta, it encounters arteries that are smaller and less elastic, with multiple branch points, causing reflections of the pressure wave. This results in a peripheral pulse contour with increased slope and amplitude, causing recorded values to be artificially elevated. As a result, distal extremity artery recordings yield higher systolic values than central aortic or femoral artery recordings. Diastolic pressures tend to be less affected, and mean arterial pressures measured at the different sites are similar [14].
The most commonly used sites for arterial cannulation in adults are the radial, femoral, axillary, dorsalis pedis, and brachial arteries. Additional sites include the ulnar, axillary and superficial temporal arteries. Peripheral sites are cannulated percutaneously with a 2-inch, 20-gauge, nontapered Teflon catheter-overneedle and larger arteries using the Seldinger technique with a prepackaged kit, typically containing a 6-inch, 18-gauge Teflon catheter, appropriate introducer needles, and guidewire.
Arterial catheterization is performed by physicians from many different specialties and usually the procedure to be performed dictates the site chosen. For example, insertion of an IABP is almost always performed through the femoral artery regardless of the specialty of the physician performing the procedure. Critical care physicians need to be facile with arterial cannulation at all sites, but the radial and femoral arteries are used successfully for more than 90% of all arterial catheterizations performed in the ICU. Although each site has unique complications, available data do not indicate a preference for any one site [15,16,17]. Radial artery cannulation is usually attempted initially unless the patient is in shock, on high dose vasopressors, and/or pulses are not palpable. If this fails, femoral artery cannulation should be performed. If catheterization at these two sites proves unsuccessful or not appropriate, then the dorsalis pedis, brachial, and axillary artery are the recommended alternative sites. Which of these is chosen depends on the exact clinical situation and the experience and expertise of the operator.
Use of Portable Ultrasound
Bedside ultrasound has not had as great an impact on arterial as it has on venous catheterization because vessel puncture is based on a palpable “landmark” that guides needle placement, and the complication rate during insertion is much lower. However, we have found ultrasound guidance to be very useful and efficient in assisting with brachial and femoral artery catheterizations, and have even used it successfully for selected difficult radial artery procedures. In our experience, ultrasound has the same impact with arterial as it does with venous catheterizations; higher success rate with less procedure time, number of attempts, and complications. Operator technique of ultrasound for arterial is the same as for venous catheterization and the reader is referred to Chapter 2 for a description of ultrasound equipment and technique. Ultrasound images for each of the major arterial routes are shown in Figure 3.2.
Radial Artery Cannulation
A thorough understanding of normal arterial anatomy and common anatomical variants greatly facilitates insertion of catheters and management of unexpected findings at all sites. The radial artery is one of two final branches of the brachial artery. It courses over the flexor digitorum sublimis, flexor pollicis longus, and pronator quadratus muscles and lies just lateral to the flexor carpi radialis in the forearm. As the artery enters the floor of the palm, it ends in the deep volar arterial arch at the level of the metacarpal bones and communicates with the ulnar artery. A second site of collateral flow for the radial artery occurs via the dorsal arch running in the dorsum of the hand (Fig. 3.3).
The ulnar artery runs between the flexor carpi ulnaris and flexor digitorum sublimis in the forearm, with a short course over the ulnar nerve. In the hand the artery runs over the transverse carpal ligament and becomes the superficial volar arch, which forms an anastomosis with a small branch of the radial artery. These three anastomoses provide excellent collateral flow to the hand [18]. A competent superficial or deep palmar arch must be present to ensure adequate collateral flow.
At least one of these arches may be absent in up to 20% of individuals.
At least one of these arches may be absent in up to 20% of individuals.
![]() Figure 3.2. Portable ultrasound images. A. Radial artery longitudinal view. B. Brachial artery axial view. C. Femoral artery axial view. D. Axillary artery axial view. See text for details. |
Modified Allen’s Test
Hand ischemia is a rare but potential devastating complication of radial artery catheterization that may require amputation [19]. Hand ischemia is rare because of the rich collateral circulation described earlier that insures perfusion even if one of the main arteries thrombose. Historically, the modified Allen’s test [20], described in previous editions of this text, was used prior to radial catheterization to detect patients’ in whom the collateral circulation may not be intact and presumably at increased risk for hand ischemia. However, as a screening tool the Allen’s test has never had very good predictive value [21] and our institution, as well as many others, has abandoned its routine use. The best way to prevent hand ischemia is to avoid radial catheterization in patients at increased risk (i.e., high dose vasopressor therapy, scleroderma, vasculopathy) and to perform clinical evaluation of hand perfusion at each nursing shift change. Any change in the hand distal to a radial artery catheter that suggests decreased perfusion (color or temperature change, paresthesias, loss of capillary refill) should prompt immediate removal of the catheter and further investigation if the changes do not reverse.

Full access? Get Clinical Tree
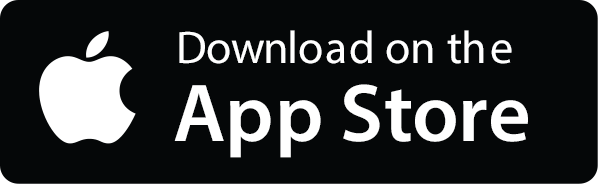
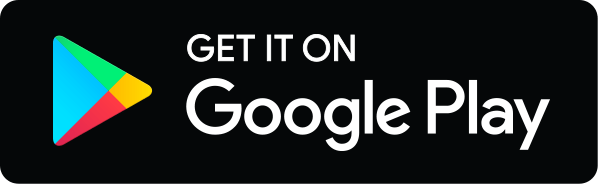
