KEY POINTS
1. Patients with moderate to severe left ventricular dysfunction are at a higher risk for sustained monomorphic VT than those with preserved left ventricular function.
2. VT more commonly causes syncope and when it is associated with structural heart disease it is also associated with a high risk of sudden cardiac death.
3. Symptomatic patients with SND or evidence of conduction disease such as second- or third-degree AV block almost always need a permanent pacemaker preoperatively.
4. For VT, IV amiodarone is the initial drug of choice, but lidocaine is also considered especially if there is concern for ongoing ischemia. For torsades de pointes, management includes eliminating offending drugs in the setting of the long QT syndromes. Correcting electrolyte deficiencies with IV magnesium and potassium are particularly helpful in correcting the prolonged QT interval, as possibly stopping medications contributing to bradycardia. Amiodarone in the setting of torsades de pointes can prolong the QT interval and make the problem worse.
5. Bifascicular block with periodic third-degree AV block and syncope is associated with an increased incidence of sudden death. Prophylactic permanent pacing is indicated in this circumstance.
6. The requirement for temporary pacing with acute MI by itself does not constitute an indication for permanent pacing.
7. Sensor-driven tachycardia may occur with adaptive-rate devices that sense vibration, impedance changes, or the QT interval if they sense mechanical or physiologic interference, which leads to inappropriate high-rate pacing. Thus, it is advised that ARP be disabled in perioperative settings.
8. Because of the unpredictable interaction between the MRI and CIEDs, MRIs are generally contraindicated in individuals with CIEDs. There is now an approved compatible pacemaker generator and lead system for individuals likely to need MRI.
9. Most contemporary pacemaker devices respond to magnet application by a device-specific single- or dual-chamber asynchronous pacing mode. Adaptive-rate response is generally suspended with magnet mode as well. With asynchronous pacing, the pacemaker will no longer be inhibited by sensed activity and instead pace at a fixed rate regardless of underlying rhythm.
10. Some manufacturers, Biotronik, St. Jude Medical, and Boston Scientific devices, have a programmable magnet mode that may make response to magnet application different than anticipated. Although rarely used, this feature may be programmed to save patient-activated rhythm recordings with magnet application rather than revert the pacemaker to asynchronous pacing.
11. EMI signals between 5 and 100 Hz are not filtered, because these overlap the frequency range of intracardiac signals. Therefore, EMI in this frequency range may be interpreted as intracardiac signals, giving rise to abnormal behavior. Possible responses include (i) inappropriate inhibition or triggering of stimulation, (ii) asynchronous pacing (Fig. 17.5), (iii) mode resetting, (iv) direct damage to the pulse generator circuitry, and (v) triggering of unnecessary ICD shocks.
12. Treatment options for VT include antitachycardia pacing, cardioversion, or defibrillation. Up to 90% of monomorphic VTs can be terminated by a critical pacing sequence, reducing the need for painful shocks and conserving battery life. With antitachycardia pacing, trains of stimuli are delivered at a fixed percentage of the VT cycle length.
13. Acute MI, severe acute acid–base or electrolyte imbalance, or hypoxia may increase defibrillation thresholds, leading to ineffective shocks. Any of these also could affect the rate or morphology of VT and the ability to diagnose VT.
14. Response of an ICD to magnet application [8]. Magnet application does not interfere with bradycardia pacing and does not trigger asynchronous pacing in an ICD. Magnet application in contemporary ICDs causes inhibition of tachycardia sensing and delivery of shock only. All current ICDs remain inhibited as long as the magnet remains in stable contact with the ICD. Once the magnet is removed, the ICD reverts to the programmed tachyarrhythmia settings.
15. Baseline information about the surgery is needed by the CIED team (cardiologist, electrophysiologist, and pacemaker clinic staff managing the device) such as (i) type and location of the procedure, (ii) body position at surgery, (iii) electrosurgery needed and site of use, (iv) potential need for DC cardioversion or defibrillation, and (v) other EMI sources.
16. For pacemaker-dependent patients: These patients are at particular risk of asystole in the presence of EMI. If EMI is likely (e.g., unipolar cautery in the vicinity of the pulse generator or leads and surgery above the umbilicus), then the device should be programmed to an asynchronous mode. In the case of pacemakers, this can be done with magnet application in most situations, which will also inactivate the rate-responsive pacing.
17. In cases where the pacemaker-dependent patient has an ICD or the location of surgery precludes placement of a magnet, consideration of programming the device to asynchronous mode with the proprietary programmer is recommended. The other alternative if reprogramming is not an option is to limit the EMI to short bursts while watching the response of pacing and minimize episodes of asystole. For patients with adaptive-rate pacemakers (including some ICDs), this capability should be programmed off if EMI causes inappropriate rate response.
18. Use of a magnet eliminates the complexity of reprogramming the CIED in the operating room. The magnet can be easily removed when competing rhythms develop with asynchronous pacing.
19. In a situation with an ICD and no device information, a magnet should not be placed over the ICD pulse generator unless EMI is unavoidable. If EMI is unavoidable, then the patient needs to be placed on cardiac monitor and a magnet will need to be placed on (and kept on) the ICD generator during cautery or RF therapy.
ANY DISTURBANCE OF RHYTHM OR conduction, or arrhythmia that destabilizes hemodynamics perioperatively will need to be addressed. Treatment with antiarrhythmic agents has been the standard approach to manage symptomatic arrhythmias acutely. More recently, electrical therapies have gained wider acceptance in rhythm management. They presently play a premiere role in patients undergoing cardiac surgery and greatly impact a growing number of noncardiac surgery cases. The emphasis in this chapter is on perioperative management of patients with implanted devices. Concepts of arrhythmogenesis, antiarrhythmic action, and drug selection are discussed only briefly. The reader is referred to Chapter 2 for discussion of specific antiarrhythmic drugs and their pharmacology and to Chapter 1 for a discussion of arrhythmogenesis.
I. Concepts of arrhythmogenesis
A. Basic electrophysiology
1. Action potential (AP). A ventricular muscle cell’s AP has five phases caused by changes in the cell membrane’s permeability to sodium, potassium, and calcium (Fig. 17.1). Phase 0 represents depolarization and is characterized by a rapid upstroke as sodium rapidly enters the cell. There is a rapid drop in the cell’s impedance from a resting state at −80 to −85 mV. Phase 1 is an early rapid repolarization period caused by potassium egress from the cell. Phase 2 is a plateau phase representing a slow recovery phase: The slow inward calcium current is counterbalanced by outward potassium current. Phase 3 is a rapid repolarization phase as a result of accelerated potassium efflux. The diastolic interval between APs is termed Phase 4 and is a cell’s resting membrane state in atrial and ventricular muscle.
Figure 17.1 Action potential (AP) and resting membrane potential (RMP) of a quiescent Purkinje fiber. Extracellular and intracellular ion concentrations during Phase 4, and the active and passive ion exchangers that restore intracellular ion concentrations during Phase 4 are shown to the right of the AP. Inward depolarizing and outward repolarizing currents are shown below the AP. The adenosine triphosphate (ATP)-dependent Na/K pump maintains steep outwardly and inwardly directed gradients (arrows) for K+ and Na+, respectively, and generates small net outward current. The passive Na/Ca exchanger generates small net inward current. A small, inward “leak” of Na+ keeps the RMP slightly positive to the K equilibrium potential (−96 mV). AP Phase 0 is the upstroke, Phase 1 is initial rapid repolarization, Phase 2 is the plateau, and Phase 3 is final repolarization. The cell is unresponsive to propagating AP or external stimuli during the absolute refractory period. A small electrotonic potential (A) occurs in response to a propagating AP or external stimulus during the relative refractory period (RRP). It is incapable of self-propagation. A normal AP is generated at the end of the RRP (B), when the Na channels have fully recovered from inactivation. It is capable of propagation. Note that threshold potential (TP) for excitation is more positive during the RRP.
2. Ion channels. Electrical activation of cardiac cells is the result of membrane currents crossing the hydrophobic lipid membranes through their specific protein channel. Opening and closing of gates in these channels are determined by the membrane potential (voltage dependence) and by the time elapsed after changes in potential (time dependence). Membrane channels cycle through the “activated,” “inactivated,” and “recovery” stages with each AP. Inward currents of sodium and calcium ions enter the cell using this gating mechanism. On the surface electrocardiogram (ECG), rapid-acting sodium currents contribute to the “P” and the “QRS” complexes. Depolarization of the sinoatrial (SA) node and the atrioventricular (AV) node, in contrast, occurs as a result of the slower-opening calcium-dependent channels. The slowly conducting calcium channel in the AV node creates the delay in the node and is responsible for nearly two-thirds of the PR interval during normal conduction. A group of potassium channels are responsible for repolarization and the “T” wave on the electrocardiogram (ECG).
Cardiac arrhythmias occur when abnormal channel proteins are substituted for the normal protein and the ion channel is altered. For example, QT prolongation may be inherited as a result of encoding an altered gene or it may be acquired as a consequence of an antiarrhythmic agent inhibiting a specific ion channel [1].
3. Excitability. Reducing the cell’s transmembrane potential to a critical level will initiate a propagated response and this level is termed the threshold potential. There are two mechanisms by which a propagated AP is developed: (i) a natural electrical stimulus and (ii) an applied electrical current. For an applied stimulus, threshold of a tissue is defined as the minimum amount of energy that will elicit a response. When external electrodes are used, only that part of the stimulus which penetrates the cell membrane contributes to excitation. The size of the stimulating electrode is critical to threshold. Reducing the size of the stimulating electrode (from 3 mm to 0.5 mm) will increase the current density over a smaller area and decrease the amount of energy needed to achieve threshold. It is common practice in pacing to use a small stimulating electrode and a larger indifferent electrode to reduce threshold and facilitate excitation.
4. Conduction. There are regions of cells with specialized conduction characteristics within the heart that propagate conduction in a preferential direction, spreading to adjacent areas faster though the preferential sites. This allows for a more organized direction of conduction so that, for example, both atria are depolarized prior to the stimulus reaching the AV node to depolarize the ventricles. In the sinus and AV nodes, L- and T-type calcium channels are the source of the propagated current [2]. In the Purkinje fiber the sodium channel is the source of the conducted current. Conduction velocity is much slower at about 0.2 m/s in the node versus 2 m/s in the Purkinje cells. These different characteristics explain the effects of certain antiarrhythmic medications. For instance, blocking the sodium channel with a Class I antiarrhythmic agent will preferentially reduce the conduction velocity in the Purkinje cells compared to the AV node because of this agent’s primary effect on sodium channels.
B. Mechanism of arrhythmia (Table 17.1)
Table 17.1 Confirmed or postulated electrophysiologic mechanisms for clinical arrhythmias
1. Automaticity. Automaticity is a unique property of an excitable cell allowing spontaneous depolarization and initiation of electrical impulse in the absence of external electrical stimulation. The SA node serves as the primary automatic pacemaker for the heart. Subsidiary pacemakers exhibiting automaticity are also found along the crista terminalis, the coronary sinus ostium, within the AV junction and in the ventricular His–Purkinje system. They may assume control of the heart if the SA node falters. Automaticity is either normal or abnormal. Alterations in automaticity due to changes in the ionic currents normally involved in impulse initiation are considered normal. Examples of normal automaticity include sinus tachycardia and junctional tachycardia during catecholamine states that increase current through the T-type calcium channels in the nodal cells. Abnormal automaticity occurs when ionic currents not normally involved in impulse initiation cause spontaneous depolarizations in atrial and ventricular muscle cells that normally do not have pacemaker activity. An example of abnormal automaticity occurs during ischemic injury causing muscle cells to shift their maximum diastolic potential to a more positive resting level and thus facilitate spontaneous depolarization [3].
2. Triggered activity. Triggered activity is the result of abnormal oscillations in membrane potential reaching the threshold to induce another AP following a normal AP depolarization. These after depolarizations can occur before full repolarization has occurred, known as early after depolarizations (EADs), or late after repolarization has occurred, known as delayed after depolarization (DAD). Oscillations of either type of after depolarizations that exceed the threshold potential may trigger an abnormal tachycardia.
EADs occur most frequently with delays in repolarization and prolongation of the QT interval. Acquired and congenital QT prolongation syndromes are predisposed to EADs and can result in torsades de pointes sometimes known as polymorphic ventricular tachycardia (VT) and sudden death. The torsades de pointes, triggered in prolonged QT syndromes, is facilitated by bradycardia and adrenaline. Examples of inherited predisposition to triggered initiation include defects in genes encoding Na and K ion channels that produce a net reduction in outward positive current during repolarization resulting in prolongation of the QT interval. Acquired prolongation of the QT interval from hypokalemia, pharmacologic agents including class IA and III antiarrhythmics, antibiotics such as erythromycin and antifungal agents, antihistamines, and the phenothiazine piperidine class can cause EADs.
Delayed after depolarization potentials cause arrhythmias related to calcium overload. Digoxin toxicity is the most common agent causing DAD, and triggered activity is the common mechanism for digitalis-induced accelerated junctional rhythm and bidirectional VT. Catecholamines facilitate calcium loading and the development of DADs.
3. Re-entry. For either anatomical or functional re-entrant excitation to occur, the electrical wave front must circle around a core of inexcitable tissue at a rate that preserves an excitable gap. For initiation, there must be (i) an area of unidirectional conduction block, (ii) two conduction pathways that are connected at each end, and (iii) an area of slow conduction. Anatomical re-entrant circuits are common to several supraventricular tachycardias (SVTs) including Wolff–Parkinson–White syndrome, with an accessory AV pathway, and typical and atypical AV nodal re-entrant tachycardia. Classic atrial flutter has an anatomical right atrial loop classically involving the area of slow conduction known as the cavotricuspid isthmus. Pathologic sustained monomorphic VT is commonly due to anatomic re-entry from and is frequently associated with scarring from a prior myocardial infarction or fibrosis associated with dilated nonischemic cardiomyopathy.
A functional re-entrant loop involves a small circuit around an area of tissue with an inexcitable core. Functional re-entry may occur with some forms of atrial tachycardia and is a mechanism for the multiple wavelet theory of atrial fibrillation.
C. Anatomical substrates and triggers
1. Supraventricular. The highly complex atria have a heterogeneous branching network of subendocardial muscle bundles that create functional areas of block as well as preferential planes of excitation. Longitudinal connected myocardial fibers conduct faster than fibers connected along transverse or parallel lines (so-called anisotropic conduction). In the right atrium, the crista terminalis and Eustachian ridge tend to act as anatomical barriers that help to facilitate a single re-entrant loop during atrial flutter. An area of slow conduction can usually be found in the isthmus of atrial tissue between the tricuspid annulus and the inferior vena cava. This is a frequent anatomical area for ablation of both clockwise and counterclockwise atrial flutter. The ostia of the pulmonary veins are frequently the sites of the initiation of atrial fibrillation. This chaotic atrial rhythm can be triggered by a single focus frequently localized to a pulmonary vein. Once initiated by a premature depolarization, the multiple wavelets are facilitated by the complex nature of the atria.
2. Ventricular. Myocardial ischemia and infarction can create both acute and chronic substrates for VT and fibrillation. As discussed above, scar from previous infarction can create an anatomic re-entrant circuit providing the substrate for re-entrant VT. In the acute events such as acute coronary occlusion, metabolic derangements occur including local hyperkalemia, hypoxia, acidosis and an increase in adrenergic tone increasing the likelihood for automaticity, triggered activity, and functional re-entry. With acute coronary occlusion, ventricular fibrillation (VF) is common and is directly related to an increase in sympathetic tone. Accelerated idiopathic ventricular rhythm is frequently seen with acute myocardial infarction and is attributed to abnormal automaticity. In the chronic phase, the infarct becomes mottled and over several weeks islands of viable muscle cells are surrounded by electrically inert barriers of scar tissue. Slow conduction is present and allows for unidirectional block. These factors are conducive to re-entry which is the mechanism for sustained monomorphic VT in about 6% to 8% of survivors of myocardial infarction. Myocardial remodeling as a consequence of infarction provides a classic substrate for arrhythmias. Patients with moderate to severe left ventricular dysfunction are at a higher risk for sustained monomorphic VT than those with preserved left ventricular function.
1
D. Neural control of arrhythmias
1. b-Adrenergic modulation. Increased sympathetic tone increases the susceptibility to VF in the early stage of myocardial infarction. In the chronic phase it facilitates initiation of sustained monomorphic VT. β-Adrenergic blocking drugs, propranolol, metoprolol, and nadolol significantly reduce the incidence of VF during acute infarction and reduce the risk of sudden death later. Also, β-blockers do not prevent reperfusion arrhythmias.
2. Parasympathetic activation. During acute infarction, vagal activation exerts a protective effect against VF. Bradycardia appears necessary for this protective effect. Increasing heart rate by pacing will negate this protective effect. Vagal stimulation does little to protect against reperfusion arrhythmias.
E. Clinical approach to arrhythmias
1. Syncope is defined as the loss of consciousness and muscle tone with spontaneous resolution. It may occur in up to 40% of the general population. The most common is vasovagal or neurally mediated syncope which is quite common in the young. This type of syncope is not associated with increased risk of death. Syncope occurs at an annual rate of 6% in patients 75 yrs old or older, but in only 0.7% of those below age 45. The goal is to determine if syncope is of the benign vasovagal variety or of the more dangerous cardiac type [4]. The 1-yr mortality from syncope of a cardiac cause can range from 18% to 33% while the noncardiac group has 0% to 6% mortality. Patients with vasovagal mediated syncope do not die from syncope. The anatomical cardiac causes of syncope result in obstruction to cardiac output, such as aortic stenosis and outflow obstruction in hypertrophic cardiomyopathy. Arrhythmias that suddenly reduce cardiac output and profoundly affect blood pressure can cause syncope. Sinus node dysfunction (SND) and AV block are common causes of bradyarrhythmias that can cause syncope. SVT causes syncope less often. VT more commonly causes syncope and when it is associated with structural heart disease it is also associated with a high risk of sudden cardiac death. In patients with syncope, check the ECG for arrhythmias such as atrial fibrillation, evidence of myocardial infarction, or conduction disease such as bundle branch block. Other important features to evaluate include pre-excitation (delta waves), the QT interval, and ectopy. Any of these abnormalities may predict a greater risk of mortality and a need for further evaluation.
2
2. Bradycardia. Heart rates less than 60 bpm are considered bradycardic. Slow heart rhythms become an issue generally in older patients who may be asymptomatic. Resting slow heart rates in young patients are most likely a result of high vagal tone and are not pathologic. Heart rates greater than 50 tend to be hemodynamically stable while those less than 40 bpm while the patient is awake often are not. If the asymptomatic bradycardic patient has no evidence of conduction disease (normal QRS morphology) and a chronotropic response to exercise, atropine or Isoproterenol, a pacemaker is rarely indicated. On the other hand, symptomatic patients with SND or evidence of conduction disease such as second- or third-degree AV block almost always need a permanent pacemaker preoperatively. Bradycardia secondary to neurocardiogenic syncope, medications, or increased vagal tone will not generally require a pacemaker. Simply removing the offending medicine or treating the inciting condition will be sufficient.
3
3. Tachycardia. Heart rates above 100 bpm are termed “tachycardia.” These can be sinus, a pathologic SVT, or VT. Sinus tachycardia rarely exceeds 140 bpm at rest unless the patient is in distress, shock, acute respiratory failure, or thyroid storm. In adults not in distress, a narrow, regular QRS tachycardia at rates above 150 bpm is rarely sinus. Regular and narrow QRS tachycardia at these rapid rates are frequently paroxysmal supraventricular tachycardia (PSVT) or atrial flutter with 2 to 1 conduction. Irregular SVTs are either the more common atrial fibrillation or multifocal atrial tachycardia. The later is seen in elderly patients with severe chronic pulmonary disease.
Wide QRS tachycardia may be ventricular or supraventricular in origin. In general, if the patient has underlying heart disease, the mechanism of the wide complex tachycardia is VT until proven otherwise. However, there are several conditions in which the mechanism may be SVT including (i) SVT with an underlying or functional bundle branch block, (ii) SVT with nonspecific intraventricular conduction delay, or (iii) pre-excitation syndrome. The ECG diagnosis of VT hinges on seeing AV dissociation or fusion or capture beats. Intraventricular conduction delay can occur with the use of Class I antiarrhythmic agents or in the setting of extreme hyperkalemia. Wolff–Parkinson–White syndrome should be considered in a young healthy individual presenting with atrial fibrillation and a wide QRS rhythm. Functional bundle branch block is seen in the young and rarely in the elderly.
II. Treatment modalities
4
A. Pharmacologic treatment. Algorithms are now in place for acute treatment of SVTs. SVT is usually treated with intravenous (IV) adenosine acutely in the symptomatic patient. For atrial fibrillation the initial focus is on rate control. Agents that are effective acutely include IV diltiazem and the β-blockers metoprolol and esmolol. Esmolol has an extremely short half-life. Digoxin is of little use acutely and is very unpredictable. IV amiodarone is now being used more frequently in the acute management of patients with poor ventricular function and atrial fibrillation with rapid ventricular rates. This medication should be infused through a central line given the risk for tissue necrosis with extravasation. The pharmacologic treatment of ventricular arrhythmias in the hemodynamically stable patient involves treating the underlying causes. For VT, IV amiodarone is the initial drug of choice, but lidocaine is also considered especially if there is concern for ongoing ischemia. For torsades de pointes, management includes eliminating offending drugs in the setting of the long QT syndromes. Correcting electrolyte deficiencies with IV magnesium and potassium are particularly helpful in correcting the prolonged QT interval, as possibly stopping medications contributing to bradycardia. Amiodarone in the setting of torsades de pointes can prolong the QT interval and make the problem worse. For the hemodynamically unstable patient with sustained ventricular arrhythmias, pharmacologic management would follow the current advanced cardiac life support (ACLS) protocols.
Proarrhythmia. Although drugs have proved safe and effective in the normal heart, their safety and efficacy have proved worrisome in the structurally abnormal heart. Chronic drug therapy for arrhythmias in patients with structural heart disease is associated with increased mortality due to proarrhythmic effects. Class IA antiarrhythmic agents are contraindicated in individuals with congestive heart failure and poor left ventricular function (ejection fraction below 0.30). Class IC agents should be avoided in individuals with a prior myocardial infarction because of the increased risk of sudden death [5]. Class III agents will prolong the QT interval and increase the risk of torsades de pointes.
B. Nonpharmacologic treatments. The emphasis on the chronic treatment for arrhythmias, especially ventricular arrhythmias in patients with structural heart disease, has moved from drugs to electricity. Because of technologic advances, cardiac implantable electrical devices (CIEDs) have become smaller and increasingly complex. This complexity has greatly expanded therapeutic options, but it has greatly increased the potential for malfunction in the perioperative setting. Except in infants and small children, a formal thoracotomy is no longer used for implantation of a CIED. Contemporary devices are small enough to be suitable for pectoral implantation.
1. External cardioversion and defibrillation. External direct-current (DC) cardioversion differs from defibrillation only in that the former incorporates a time delay circuit for shock synchronization to the QRS complex of the surface ECG. Current devices employ universal use of biphasic shocks, which lower shock current requirements for DC cardioversion and defibrillation. Automated external defibrillators self-analyze and give instructions for defibrillation [6].
a. Indications: Synchronized shocks are used for most pathologic hemodynamically unstable tachycardias, except VF or VT when the QRS complex cannot be distinguished from T waves. Automatic rhythm disturbances (e.g., accelerated AV junctional or accelerated idioventricular rhythms) are not amenable to DC cardioversion.
b. Procedure: Synchronized cardioversion with the largest R or S wave on the ECG will prevent inadvertent triggering of VF. Improper synchronization may occur when there is bundle branch block with a wide R wave, when the T wave is highly peaked, and with pacing artifacts from a malfunctioning pacemaker (i.e., failure to capture). Synchronization should be checked after each discharge. Electrodes are placed in an anterior-lateral, posterior-lateral, or an anteroposterior (AP) position. Current should pass though the heart’s long axis, depolarize the bulk of myocardium, and minimize flow through high-impedance bony tissue. Electrode paste or gel is used to reduce transthoracic impedance. Bridging of the electrodes by conductive paste or gel should be avoided, because this will reduce the amount of energy delivered to the heart. Present-day units automatically boot to an energy setting of 200 J. This is the starting energy dosage for defibrillating adults. For cardioversion, energy titration (initially use only the lowest possible energies) reduces both energy use and complications. Initial settings of 20 to 50 J may be successful for terminating typical atrial flutter or stable monomorphic VT. DC cardioversion is extremely painful. Patients must be sedated for DC cardioversion at any power setting. Generally, an anesthesiologist or nurse anesthetist will administer a short-acting sedative such as IV propofol or etomidate. The combination of midazolam and fentanyl can be an alternative but is not ideal due to the prolonged duration of action.
c. CIEDs and cardioversion. Older and especially unipolar devices could be easily affected by DC cardioversion. Transient loss of capture and electrical reset were not uncommon. This interference does not happen with modern bipolar and well- protected devices. Using the anterior–posterior pads position and the anterior pad location at least 8 cm from the CIED will prevent malfunction or damage to the device. Directly applied currents of 10 to 30 J to the ventricles during cardiac surgery on occasion can cause reset of the pulse generator.
2. Temporary pacing. Compared to drugs for treating cardiac rhythm disturbances, temporary pacing has several advantages. The effect is immediate, control is precise, and there is reduced risk of untoward effects and proarrhythmia.
a. Indications. Temporary pacing is indicated for rate support in patients with symptomatic bradycardia or escape rhythms. Prophylactic or stand-by pacing is indicated for patients at increased risk for sudden high-degree AV heart block. Temporary pacing can be used to overdrive or terminate atrial flutter and some sustained monomorphic VT. More specific established and emerging indications for temporary pacing are shown in Table 17.2. The endpoint for temporary pacing is resolution of the indication or implantation of a permanent pacemaker for a continuing indication.
Table 17.2 Usual and less-established indications for temporary cardiac pacing
b. Technology. Transvenous endocardial or epicardial leads are usually used for temporary pacing. Temporary endocardial bipolar active fixation wires are available for both atrial and ventricular pacing. Single- or dual-chamber pacing can be achieved. Transvenous leads are passed from above using the internal jugular or subclavian approaches or from below using a femoral vein. Epicardial leads are routinely used in patients having cardiac surgery. The noninvasive transcutaneous and transesophageal routes are also available. Transcutaneous pacing is uncomfortable and used in emergency situations. In the operating room it is for transient backup pacing only. It produces ventricular capture and does not preserve optimal hemodynamics in patients with intact AV conduction. With available technology for transesophageal pacing, only atrial pacing is reliable. Thus, the method is not suitable for patients with advanced AV block or atrial fibrillation.
3. Permanent pacing. Permanent pacemakers are no longer prescribed simply for rate support. They have become an integral part of treatment, along with drugs and other measures, to prevent arrhythmias and improve quality of life in patients with heart failure [7].
a. Indications. The presence or absence of symptoms directly attributable to bradycardia has an important influence on the decision to implant a permanent pacemaker. There is increasing interest in multisite pacing as part of the management for patients with structural heart disease and heart failure. In the past, pacemakers were prescribed to treat re-entrant tachyarrhythmias. Today, this capability can be programmed for either the atrium or ventricle as part of “tiered therapies” with an internal cardioverter–defibrillator (ICD).
(1) AV block. Patients may be asymptomatic or have symptoms related to bradycardia, ventricular arrhythmias, or both. There is little evidence that pacing improves survival with isolated first-degree AV block. With Type I, second-degree AV block due to AV nodal-conduction delay, progression to higher-degree block is unlikely. Pacing is usually not indicated unless the patient has symptoms. With Type II, second-degree AV block within or below the His bundle, symptoms are frequent, prognosis is poor, and progression to third-degree AV block is common. Pacing is recommended for chronic Type II second-degree AV block. It is recommended for Type I second-degree AV block in the presence of symptoms such as syncope. Pacing improves survival in both types of second-degree AV block. Nonrandomized studies strongly suggest that pacing improves survival in patients with third-degree AV block.
(2) Bifascicular and trifascicular block. Although third-degree AV block is commonly preceded by bifascicular block, the rate of progression is slow (years). Further, there is no credible evidence for acute progression to third-degree AV block during anesthesia and surgery. Bifascicular block with periodic third-degree AV block and syncope is associated with an increased incidence of sudden death. Prophylactic permanent pacing is indicated in this circumstance.
5
(3) AV block after acute MI. The requirement for temporary pacing with acute MI by itself does not constitute an indication for permanent pacing. The long-term prognosis for survivors of acute MI is related primarily to the extent of myocardial injury and nature of intraventricular conduction defects, rather than to AV block itself. Acute MI patients with intraventricular conduction disturbances have unfavorable short- and long-term prognoses, with increased risk of sudden death. This prognosis is not necessarily due to the development of high-grade AV block, although the incidence of such block is higher in these patients.
6
(4) SND. SND may manifest as sinus bradycardia, pause or arrest, or SA block, with or without escape rhythms. It often occurs in association with atrial fibrillation or atrial flutter (tachycardia–bradycardia syndrome). Patients with SND may have symptoms due to bradycardia, tachycardia, or both. Correlation of symptoms with arrhythmias is essential and is established by ambulatory monitoring. SND also presents as chronotropic incompetence (inability to increase rate appropriately). An adaptive-rate pacemaker may benefit these patients by restoring more physiologic heart rates. Although symptomatic SND is the primary indication for a pacemaker, pacing does not necessarily improve survival, but it can improve the quality of life.
(5) Hypersensitive carotid sinus syndrome or neurally mediated syndrome. Hypersensitive carotid sinus syndrome is manifest by syncope due to an exaggerated response to carotid sinus stimulation. It is an uncommon cause of syncope. If purely cardioinhibitory (asystole, heart block) and without vasodepressor components (vasodilatation), then a pacemaker can be prescribed. A hyperactive response is defined as asystole for longer than 3 s due to sinus arrest or heart block and an abrupt decrease in blood pressure. With the more common neurally mediated mixed response, attention to both components is essential for effective therapy. Neurally mediated (vasovagal) syncope accounts for nearly 25% of all syncope. The role of permanent pacing is controversial but probably limited.
(6) Pacing in children and adolescents. Indications for pacing are similar in children and adults, but there are additional considerations. For example, what is the optimal heart rate for the patient’s age? Further, what is optimal given ventricular dysfunction or altered circulatory physiology? Hence, pacing indications are based more on correlation of symptoms with bradycardia, rather than arbitrary rate criteria, and include the following:
(a) Bradycardia only after other causes (e.g., seizures, breath holding, apnea or neurally mediated mechanisms) are excluded.
(b) Symptomatic congenital third-degree AV block
(c) Persistent advanced second- or third-degree AV block after cardiac surgery. However, for patients with residual bifascicular block and intermittent AV block, the need is less certain.
(d) Use along with β-blockers in patients with congenital long QT syndrome, especially with pause-dependent VT.
(7) Miscellaneous pacing indications
(a) A dual-chamber pacemaker with short AV delay reduces left ventricular outflow tract obstruction, alleviates symptoms in obstructive hypertrophic cardiomyopathy in some cases, and may improve functional status. Permanent pacing does not reduce mortality or prevent sudden death in this disease.
(b) Bradyarrhythmias after cardiac transplantation are mostly due to SND. Cardiac transplantation today preserves the sinus node so SND is much less likely. Most patients with bradycardia show improvement by 1 yr so that long-term pacing is unnecessary.
(c) A combination of pacing and β-blockers may be used for prophylaxis for tachyarrhythmias in congenital long QT syndrome. Pacing therapy alone is not recommended. Backup dual-chamber defibrillator therapy is now preferred.
b. Technology. Contemporary single- and dual-chamber pacemakers are sophisticated devices, with multiple programmable features, including automatic mode switching, rate adaptive pacing, automatic threshold pacing, and programmable lead configuration. Pacemakers are powered by lithium iodide batteries, with an expected service life of 5 to 12 yrs, depending on device capabilities, need for pacing, and programmed stimulus parameters. Most systems use transvenous leads. Lead configuration is programmable. With the unipolar configuration, the pacemaker housing (can) serves as anode (+) and the distal electrode of the bipolar pacing lead as cathode (–). With the bipolar configuration, proximal and distal lead electrodes serve as anode and cathode, respectively. The ability to program unipolar pacing is necessary if lead insulation or conductor failure occurs in a bipolar lead system. Also, it permits exploitation of either configuration while minimizing its disadvantages (e.g., oversensing with unipolar leads). A dual-chamber pacemaker with automatic mode switching is optimal for patients with AV block and susceptibility to paroxysmal atrial fibrillation. Algorithms detect fast, nonphysiologic atrial rates and automatically switch the pacing mode to one that excludes atrial tracking and the associated risk of upper-rate ventricular pacing.
4. Implantable cardioverter—defibrillator. Contemporary ICDs are multiprogrammable, are longer lived, use transvenous leads, and may incorporate all capabilities of a modern dual-chamber pacemaker. ICDs are powered by combination of both batteries and capacitors. Many current models also have wireless technology that allows patients to have remote follow-up via their home phone lines for routine ICD evaluations limiting the in-person visits to the pacer clinic or doctor’s office to annual or bi-annual evaluations. Additionally, ICDs have multiple tachycardia detection zones, with programmable detection criteria and “tiered therapy” for each (antitachycardia pacing → cardioversion shocks → defibrillatory shocks if necessary). ICDs also store arrhythmia event records and treatment results. Future devices will be tailored to meet all nonpharmacologic aspects of cardiac rhythm management for individual patients. Finally, ICDs have undergone significant downsizing (50 mL or smaller) and nearly all are prepectoral implants.
a. Indications. ICDs are used for secondary or primary prevention of sudden death.
(1) Secondary prevention. ICDs are used for secondary prevention in patients who have survived a cardiac arrest from sustained ventricular arrhythmias. Most commonly these are patients with heart failure and reduced left ventricular systolic function. Of this population, coronary artery disease and ischemic cardiomyopathy are the most common etiologies of the heart failure. Secondary prevention indications for ICDs also include individuals with structural heart disease who have documented sustained ventricular tachyarrhythmias or inducible sustained ventricular tachyarrhythmias by electrophysiologic testing. ICDs are widely accepted for improving outcomes in these patients by preventing sudden cardiac death. Other indications for secondary prevention include patients with long QT syndrome and recurrent syncope, sustained ventricular arrhythmias, or sudden cardiac arrest despite drug therapy. ICD plus Class IA drugs are prescribed for patients with idiopathic VF and Brugada syndrome with recurrent ventricular arrhythmias. Other indications are (i) sudden death survivors with hypertrophic cardiomyopathy; (ii) prophylaxis for syncope and sudden death with drug-refractory arrhythmogenic right ventricular dysplasia; and (iii) children with malignant tachyarrhythmias or sudden death and congenital heart disease, cardiomyopathies, or primary electrical disease (e.g., long QT syndrome).
(2) Primary prevention. ICDs are used for primary prevention of sudden death in patients who are at high risk for sudden cardiac death. This population mainly includes those with systolic heart failure with ejection fractions ≤35% that has not improved despite medical therapy. The cause of heart failure may be from coronary artery disease or of nonischemic origin. Other indications for primary prevention ICDs include patients with high risk features with inherited or acquired conditions that place them at increased risk for life-threatening ventricular arrhythmias including long QT syndrome, hypertrophic cardiomyopathy, arrhythmogenic right ventricular dysplasia, cardiac sarcoid, Brugada syndrome, and congenital heart disease.
b. Technology. The ICD pulse generator is a self-powered minicomputer with one or two (in series) batteries that power the pulse generator, circuitry, and aluminum electrolytic capacitors. The batteries may be lithium–silver vanadium oxide or evolving hybrid technology and vary between the manufacturers. A major challenge in ICD design is the large range of voltages within a very small package. Intracardiac signals may be as low as 100 μV, and therapeutic shocks approach 750 V. Further, ICD batteries contain up to 20,000 J, and a potential hazard exists if the charging and firing circuits were to electrically or thermally unload all this energy into the patient in a brief time period. The number of shocks delivered during treatment is usually limited to five or six per arrhythmia. The expected service life is 5 to 8 yrs.
III. Device function, malfunction, and interference [8]
A. Pacemakers. A single-chamber pacemaker stimulates the atria or ventricles at programmed timing intervals. Sensing spontaneous atrial or ventricular depolarizations inhibit the device from delivering unnecessary or inappropriate stimuli. Dual-chamber devices time the delivery of ventricular stimuli relative to sensed atrial depolarizations to maintain proper AV synchrony. Figure 17.2 illustrates how a pacemaker might be configured to pace in patients with SND or atrioventricular heart block (AVHB).
In Figure 17.2 and throughout this chapter, the North American Society for Pacing and Electrophysiology–British Pacing and Electrophysiology Group (NASPE/BPEG) pacemaker code (also known as the NBG code) is used as a short-hand to describe pacing modes (Table 17.3).
Table 17.3 NASPE/BPEG (NBG) pacemaker code used as shorthand to designate pacing modes

Full access? Get Clinical Tree
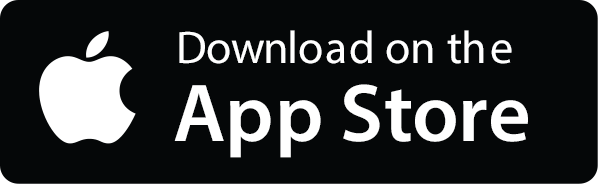
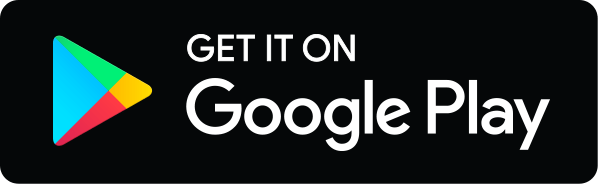