Approach to the Patient with Hypotension and Hemodynamic Instability
Michael M. Givertz
James C. Fang
Hypotension and hemodynamic instability are frequently encountered clinical problems in the intensive care setting. When the mean arterial blood pressure falls below approximately 60 mm Hg, end-organ perfusion becomes compromised and is manifested clinically as cool skin, decreased urine output, and altered mental status. Cornerstones of management include volume resuscitation and therapy directed toward the underlying cause of hypotension (e.g., cardiac pacing for bradycardia, cardioversion or defibrillation for tachyarrhythmias, blood transfusion for gastrointestinal bleeding, corticosteroids for adrenal insufficiency). When these measures fail to restore blood pressure and vital organ perfusion or while awaiting their availability, administration of intravenous vasoactive agents may be necessary. This chapter reviews the general management of the hypotensive patient with an emphasis on coronary care and the pharmacologic properties of commonly used vasopressor and positive inotropic agents. An overview of shock (see Chapter 157), volume resuscitation (see Chapter 158), sepsis (see Chapter 159), the use of intra-aortic balloon counterpulsation and mechanical circulatory support devices (see Chapter 45) are discussed elsewhere.
General Approach to the Hypotensive Patient in the Coronary Care Unit
The assessment of the hypotensive patient begins with accurate measurement of the blood pressure and rapid correlation with clinical signs of hypoperfusion. Blood pressure should be measured in both arms and confirmed by another examiner. This practice is especially important when automated devices are used to make these measurements in the setting of tachyarrhythmias or respiratory distress. In patients with peripheral arterial disease, upper extremity blood pressure should also be compared to measurements in the legs in the supine position. In rare circumstances, true central aortic pressure may differ significantly from peripherally obtained blood pressures and can only be confirmed by invasive measurement during diagnostic catheterization. This situation should be suspected when clinical features of hypoperfusion do not accompany low blood pressure.
Hypotension is generally defined as a mean arterial pressure of less than 60 mm Hg and/or a systolic blood pressure less than 100 mm Hg. However, higher values may be consistent with clinically relevant hypotension if there are concomitant clinical signs of hypoperfusion such as mental confusion, oliguria, pallor, and cool extremities. If clinically relevant hypotension cannot be rapidly corrected, invasive monitoring with an arterial line should be considered, especially if vasoactive medications are employed. Central venous catheterization should also be considered to monitor intravascular volume, since volume status is often dynamic in the hypotensive patient and multiple mechanisms of hypotension may be simultaneously present. Foley catheterization should also be employed to assess hourly urine output as a surrogate for end-organ perfusion.
The history and physical examination should be directed toward establishing the primary mechanism and etiology of hypotension. Primary mechanisms include hypovolemia, low cardiac output, and vasodilation. Assessing volume status is critical; if not discernible from the bedside evaluation (jugular venous pressure, skin turgor, urine output, orthostasis), invasive measurement of the central venous pressure should be obtained with placement of a central venous catheter. If there are clinical reasons to suggest a dissociation of right and left ventricular hemodynamics (i.e., right ventricular infarction), pulmonary artery catheterization may be required to measure the left ventricular filling pressure. Warm well-perfused skin and extremities despite hypotension may suggest low systemic vascular resistance and a vasodilatory state, whereas cool clammy skin and extremities suggest vasoconstriction as a compensatory response to a low output syndrome. A narrow pulse pressure may also suggest reduced cardiac output. If a putative mechanism of hypotension cannot be ascertained from bedside assessment, pulmonary artery catheterization can be used to characterize the hemodynamic profile. This strategy is especially useful when more than one mechanism is present (for example, a large myocardial infarction complicated by pneumonia, leading to cardiogenic and vasodilatory shock).
Initial management strategies are directed at the primary etiology of hypotension and addressed later in this chapter. In general, therapy is guided by the primary pathophysiologic mechanism underlying the hypotension (e.g., volume resuscitation for hypovolemia, positive inotropes for low cardiac output, vasopressors for vasoplegia). The pace and aggressiveness of therapeutic intervention are guided by the presence or absence of clinical signs of hypoperfusion. For example, holding vasodilators may be sufficient in the hypotensive patient without changes in mental status or urine output. In contrast, the acutely hypotensive patient with clinical shock needs rapid resuscitation with intravascular volume expansion and usually vasoactive therapy.
Adrenergic Receptor Physiology
Most vasopressor and positive inotropic agents currently available for use are sympathomimetic amines that exert their action
by binding to and stimulating adrenergic receptors. To better understand the similarities and differences among these agents, a basic knowledge of adrenergic receptor distribution and function is required [1].
by binding to and stimulating adrenergic receptors. To better understand the similarities and differences among these agents, a basic knowledge of adrenergic receptor distribution and function is required [1].
The adrenergic receptors that are most relevant to the management of hypotension are the α1, β1, and β2 receptors. α1-Adrenergic receptors are present in smooth muscle cells of many vascular beds, including the arterioles supplying the skin, mucosa, skeletal muscle, and kidneys, as well as the peripheral veins. α1-Adrenergic stimulation causes vasoconstriction and is the most common mechanism of vasopressor action. The presence of α1 receptors has also been demonstrated in the myocardium, where stimulation appears to result in a positive inotropic effect with little change in heart rate. β1-Adrenergic receptors are the predominant adrenergic receptor type in the heart and they mediate positive inotropic, chronotropic and lusitropic responses. Stimulation of β2-adrenergic receptors causes relaxation of smooth muscle cells in bronchial, gastrointestinal, and uterine muscle, as well as vasodilation in skeletal muscle. β3-adrenergic receptors, which are located mainly in adipose tissue, are involved in the regulation of lipolysis and thermogenesis and do not play a role in hemodynamic stability. Other relevant receptors are the dopaminergic receptors (DA1 and DA2), which mediate renal, coronary, cerebral, and mesenteric vasodilation, and cause a natriuretic response.
The receptor selectivity of sympathomimetic amines can be drug and dose dependent. For example, β2 receptors are more sensitive to epinephrine than are α1 receptors. Thus, at low doses of epinephrine, the vasodilatory effect of β2 receptors predominates, whereas at high doses, α1-mediated vasoconstriction overcomes the β2 effect and increases systemic vascular resistance. The dose-dependent actions of dopamine have also been well established.
The overall clinical effects of vasoactive agents depend not only on the outcome of direct adrenergic receptor stimulation, but also on the reflex response of homeostatic forces. For example, stimulation of β1-adrenergic receptors by norepinephrine would be expected to cause an increase in heart rate; however, norepinephrine-mediated α1-adrenergic stimulation induces a reflex increase in vagal tone that cancels out its positive chronotropic effects. The action of some drugs (e.g., dopamine and ephedrine) is further complicated by their ability to stimulate release of stored endogenous catecholamines.
Table 32.1 Dose Range, Receptor Activity, and Predominant Hemodynamic Effects of Vasoactive Drugs Commonly Used to Treat Hypotension | |||||||||||||||||||||||||||||||||||||||||||||||||||||||||||||||||||||||||||||||||||||||||||||||||||
---|---|---|---|---|---|---|---|---|---|---|---|---|---|---|---|---|---|---|---|---|---|---|---|---|---|---|---|---|---|---|---|---|---|---|---|---|---|---|---|---|---|---|---|---|---|---|---|---|---|---|---|---|---|---|---|---|---|---|---|---|---|---|---|---|---|---|---|---|---|---|---|---|---|---|---|---|---|---|---|---|---|---|---|---|---|---|---|---|---|---|---|---|---|---|---|---|---|---|---|
|
Commonly Used Vasopressors and Positive Inotropes
The armamentarium of vasoactive agents has changed little since the 1980s. Commonly used drugs with vasopressor activity are dopamine, epinephrine, norepinephrine, phenylephrine, and ephedrine. Vasopressin is a newer alternative to adrenergic vasopressors. Agents with positive inotropic activity that are useful for the treatment of hypotension include dobutamine, dopamine, epinephrine, and isoproterenol. Table 32.1 summarizes the receptor activity and hemodynamic effects of these drugs.
Dopamine
Dopamine is an endogenous catecholamine that functions as a central neurotransmitter and a synthetic precursor of norepinephrine and epinephrine. When administered intravenously, the effects of dopamine are mediated by dose-dependent stimulation of dopaminergic and adrenergic receptors, and by stimulation of norepinephrine release from nerve terminals.
At low doses (less than 5 μg per kg per minute), dopamine predominantly stimulates dopaminergic receptors in renal, mesenteric, and coronary vessels with minimal adrenergic effects. In normal subjects, so-called renal-dose dopamine augments renal blood flow, glomerular filtration rate, and natriuresis, with little effect on blood pressure. Low-dose dopamine has frequently been used by itself or in combination with other drugs as a renoprotective agent. However, the efficacy and safety of this strategy remain controversial [2]. Although a recent study demonstrated renal vasodilatory effects of dopamine in patients with heart failure [3], a randomized placebo-controlled trial in 328 critically ill patients with evidence of early renal dysfunction demonstrated no protective effect of low-dose dopamine on renal function and no difference in ICU or hospital length of stay [4]. Moderate doses of dopamine (5 to 10 μg per kg per minute) stimulate β1-adrenergic receptors in the myocardium, augmenting cardiac output by increasing contractility and, to a lesser extent, heart rate (Fig. 32.1). In addition, venoconstriction mediated by serotonin and dopaminergic receptors may occur [5]. At higher doses (greater than 10 μg per kg per minute), α1-adrenergic
receptor stimulation predominates, resulting in systemic arteriolar vasoconstriction. The overall effects of dopamine at the highest doses resemble those of norepinephrine (see later). However, it should be remembered that there is a great deal of overlap in the dose-dependent effects of dopamine in critically ill patients [1,2].
receptor stimulation predominates, resulting in systemic arteriolar vasoconstriction. The overall effects of dopamine at the highest doses resemble those of norepinephrine (see later). However, it should be remembered that there is a great deal of overlap in the dose-dependent effects of dopamine in critically ill patients [1,2].
Moderate- to high-dose dopamine is a mainstay in the treatment of hypotension. In studies of fluid-resuscitated patients with septic shock, dopamine produced a mean increase in mean arterial pressure of approximately 25%, primarily owing to an increase in cardiac index and, to a lesser extent, systemic vascular resistance [2]. In the setting of hyperdynamic septic shock when excessive vasodilation is the primary source of hypotension, addition or substitution of a more potent α-adrenergic agonist such as norepinephrine may be more effective. Moreover, evidence of worsening splanchnic oxygen utilization with the use of high-dose dopamine has made it a less attractive agent.
By itself or in combination with other agents, dopamine may be used at moderate doses in the management of patients with acute decompensated heart failure and hypotension. Venodilating agents (e.g., nitroprusside and nitroglycerin) may be added to moderate the tendency of dopamine to increase cardiac-filling pressures [6]. Dopamine may also be combined with dobutamine for added inotropic effects or used at low doses to augment diuresis [7], although the benefits of “renal-dose” dopamine remain controversial and other agents may be more effective for preserving renal function in critically ill patients [8].
The use of dopamine is associated with several adverse effects, including tachycardia, tachyarrhythmias, and excessive vasoconstriction. Although these effects are generally dose dependent, in individual patients there may be substantial overlap of receptor affinity such that even at low doses dopamine may result in toxicity. In patients with ischemic heart disease, increased myocardial oxygen consumption coupled with some degree of coronary vasoconstriction with high-dose dopamine can result in myocardial ischemia. As with other positive inotropes, dopamine can increase flow to poorly oxygenated regions of the lung and cause shunting and hypoxemia. In addition, dopamine has been shown to depress minute ventilation in normoxic heart failure patients [9]. When dopamine is used in patients with acute decompensated heart failure, increased venous tone and pulmonary arterial pressure may exacerbate pulmonary edema in the setting of already high cardiac filling pressures. Despite these caveats, oxygen saturation generally remains constant due to improved hemodynamics.
There is mounting evidence that dopamine adversely effects splanchnic perfusion at doses usually required to treat septic shock. A small, randomized study of patients with sepsis using selective splanchnic and hepatic cannulation showed that infusion of dopamine was associated with a disproportionate increase in splanchnic oxygen delivery compared with oxygen extraction (65% vs. 16%). In contrast, norepinephrine produced better-matched increases in oxygen delivery and extraction (33% vs. 28%) [10]. Another study showed that in patients with septic shock randomly assigned to treatment with norepinephrine or dopamine, gastric intramucosal pH worsened significantly in patients treated with dopamine despite similar improvements in mean arterial pressure [11]. Thus, the use of dopamine in septic shock may be associated with splanchnic shunting, impairment of gastric mucosal oxygenation, and increased risk of gastrointestinal bleeding [2].
Epinephrine
Epinephrine is an endogenous catecholamine that is a potent nonselective agonist of α- and β-adrenergic receptors.
Stimulation of myocardial β1 and β2 receptors increases contractility and heart rate, resulting in a rise in cardiac output (Fig. 32.2). Cardiac output is further augmented by an increase in venous return as a result of α1-mediated venoconstriction. Blood flow to skeletal muscles is increased owing to β2-mediated vasodilation. With very low-dose infusions of epinephrine (0.01 to 0.05 μg per kg per minute), β-adrenergic–mediated positive chronotropic and inotropic effects predominate. Diastolic blood pressure and overall peripheral vascular resistance may actually decrease owing to vasodilation in skeletal muscle. With higher doses of epinephrine, stimulation of α-adrenergic receptors in precapillary resistance vessels of the skin, mucosa, and kidneys outweighs β2-mediated vasodilation in skeletal muscle, causing increased mean and systolic blood pressure [1].
Stimulation of myocardial β1 and β2 receptors increases contractility and heart rate, resulting in a rise in cardiac output (Fig. 32.2). Cardiac output is further augmented by an increase in venous return as a result of α1-mediated venoconstriction. Blood flow to skeletal muscles is increased owing to β2-mediated vasodilation. With very low-dose infusions of epinephrine (0.01 to 0.05 μg per kg per minute), β-adrenergic–mediated positive chronotropic and inotropic effects predominate. Diastolic blood pressure and overall peripheral vascular resistance may actually decrease owing to vasodilation in skeletal muscle. With higher doses of epinephrine, stimulation of α-adrenergic receptors in precapillary resistance vessels of the skin, mucosa, and kidneys outweighs β2-mediated vasodilation in skeletal muscle, causing increased mean and systolic blood pressure [1].
Epinephrine plays a central role in cardiovascular resuscitation (see Chapter 23) and the management of anaphylaxis (see Chapter 194). Epinephrine is also used to reverse hypotension with or without bradycardia after cardiopulmonary bypass or cardiac transplantation [12]. Because of its adverse effects on splanchnic and renal blood flow and potential for inducing myocardial ischemia and tachyarrhythmias, epinephrine has generally been regarded as a second-line agent in the management of septic shock [2,13]. However, a recent randomized trial showed no difference in efficacy or safety between epinephrine alone versus norepinephrine plus dobutamine in patients with septic shock [14]. For patients with symptomatic bradycardia and hypotension who have failed atropine or external pacing, epinephrine may be used to stabilize the patient while awaiting more definitive therapy (e.g., transvenous placement of a temporary or permanent pacemaker) [15]. When used to treat hypotension, epinephrine is given as a continuous infusion starting at a low dose (0.5 to 1.0 μg per minute) and titrating up to 10 μg per minute as needed. Continuous infusions of epinephrine may cause restlessness, tremor, headache, and palpitations. Epinephrine should be avoided in patients taking β-adrenergic antagonists, as unopposed α-adrenergic vasoconstriction may cause severe hypertension and cerebral hemorrhage.
Norepinephrine
Norepinephrine is an endogenous catecholamine that is a potent β1– and α1-adrenergic agonist, with little β2 activity. The main cardiovascular effect of norepinephrine is dose-dependent arterial and venous vasoconstriction owing to α-adrenergic stimulation (Fig. 32.2). The positive inotropic and chronotropic effects of β1 stimulation are generally counterbalanced by the increased afterload and reflex vagal activity induced by the elevated systemic vascular resistance. Thus, heart rate and cardiac output usually do not change significantly, although cardiac output may increase or decrease depending on vascular resistance, left ventricular function, and reflex responses [5].
Norepinephrine, when infused at doses ranging from 0.5 to 30.0 μg per minute, is a potent vasopressor. Although generally reserved as a second-line agent or used in addition to other vasopressors in cases of severe distributive shock, norepinephrine is emerging as an agent of choice for the management of hypotension in hyperdynamic septic shock [14,16]. In a small, prospective double-blind trial, Martin et al. [17] randomized patients with hyperdynamic septic shock to dopamine or norepinephrine titrated to a mean arterial pressure greater than or equal to 80 mm Hg or systemic vascular resistance greater than 1,100 dynes per second per cm– 5, or both. Although only 5 of 16 patients randomized to dopamine were able to achieve these endpoints, 15 of 16 patients randomized to norepinephrine were successfully treated with a mean dose of 1.5 μg per kg per minute. Moreover, 10 of the 11 patients who remained hypotensive on high-dose dopamine improved with the addition of norepinephrine. A subsequent prospective, nonrandomized, observational study suggested that in adults with septic shock treated initially with high-dose dopamine or norepinephrine, the use of norepinephrine was associated with improved survival [18]. In the setting of sepsis, norepinephrine improves renal blood flow and urine output [19], although large doses may be required to achieve these effects due to α-receptor downregulation [2].
Adverse effects of norepinephrine include increased myocardial oxygen consumption causing ischemia and renal and mesenteric vasoconstriction. Renal ischemia, may be of particular concern in patients with hemorrhagic shock. Norepinephrine can also cause necrosis and sloughing at the site of intravenous injection owing to drug extravasation. Norepinephrine is relatively contraindicated in patients with hypovolemia. As previously discussed, the overall effect of norepinephrine on gut mucosal oxygenation in septic patients compares favorably with that of high-dose dopamine.
Phenylephrine
Phenylephrine is a synthetic sympathomimetic amine that selectively stimulates α1-adrenergic receptors. When administered intravenously, phenylephrine causes dose-dependent arterial vasoconstriction and increases peripheral vascular resistance. As blood pressure rises, activation of vagal reflexes causes slowing of the heart rate.
Phenylephrine, infused at 40 to 180 μg per minute, is commonly used in the management of anesthesia-induced hypotension [20,21] and hyperdynamic septic shock. Its rapid onset of action, short duration, and primary vascular effects make it an ideal agent for treating hemodynamically unstable patients in the intensive care setting. However, there are few data regarding its relative efficacy compared with older vasopressors such as norepinephrine and dopamine. In one small study of fluid-resuscitated patients with septic shock, the addition of phenylephrine to dobutamine or dopamine increased mean arterial pressure and systemic vascular resistance without a change in heart rate [22]. In addition, urine output improved while serum creatinine remained stable. The absence of β-adrenergic agonist activity at usual doses (phenylephrine activates β receptors only at much higher doses) makes phenylephrine an attractive agent for the management of hypotension in clinical situations where tachycardia or tachyarrhythmias, or both, limit the use of other agents [2]. As with other vasopressors, high-dose phenylephrine may cause excessive vasoconstriction. In addition, patients with poor ventricular function may not tolerate the increased afterload induced by α1-stimulation [22]. Compared to epinephrine and norepinephrine, phenylephrine is less likely to decrease microcirculatory blood flow in the gastrointestinal tract [23].
Ephedrine
Ephedrine is a naturally occurring sympathomimetic amine derived from plants. Its pharmacologic action results from direct nonselective activation of adrenergic receptors, as well as stimulation of norepinephrine release from storage sites. Although ephedrine is less potent and longer acting (half-life, 3 to 6 hours) than epinephrine, its hemodynamic profile is similar and includes cardiac stimulation and peripheral vasoconstriction.
Ephedrine is rarely used in the critical care setting except in the temporary treatment of hypotension induced by spinal anesthesia [20]. Ephedrine does not appear to compromise uterine blood flow and is considered by some to be the vasopressor of choice in the treatment of anesthesia-induced hypotension in the obstetric patient [24]. However, prophylactic use in pregnant woman undergoing Caesarian section is not recommended as it may cause hypertension and tachycardia [25]. Ephedrine can be administered in doses of 10 to 25 mg, given as an intravenous bolus every 5 to 10 minutes, with the total dose not to exceed 150 mg in 24 hours. In healthy women undergoing elective cesarean delivery that develop hypotension, pharmacogenomic data suggests that β2-adrenoceptor genotype may affect dose requirements [26]. Adverse effects of ephedrine include myocardial ischemia and excessive vasoconstriction.

Full access? Get Clinical Tree
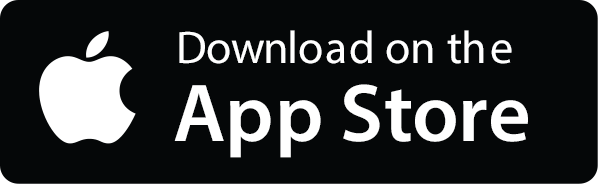
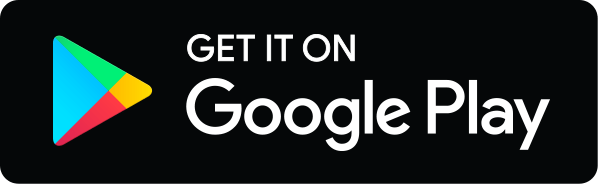