Chapter 2
Approach to Mechanical Ventilation
The “Generic” Positive Pressure Ventilator
Although modern microprocessor-controlled ventilators have a large array of settings, controls, and displays on their consoles, all positive pressure ventilators operate by the same basic elements and parameters (Figure 2.1 and Table 2.1). The mode of ventilation selected and the patient’s specific clinical circumstances should determine the exact settings.
TABLE 2.1
Basic Ventilator Parameters and Typical Initial Settings
∗Initial settings for 70-kg PBW adult with normal lungs (see Appendix E for tidal volumes based on PBW for men and women). Set initial rate higher than 8 to 12 BPM if lung disease is present—for example, asthma and COPD, depending on the disease (see Appendix B for more considerations of dead space).
Principles and Practice of Positive Pressure Ventilation
Ventilating the Respiratory System
The respiratory system resembles a balloon in that it inflates during inspiration, followed by passive deflation during expiration (Figure 2.2). This model has two mechanical elements: compliance and resistance. Compliance relates the gas volume in the balloon to the pressure inside the balloon under static conditions. It determines the balloon’s internal pressure (static recoil pressure) after inflation to a certain volume. Resistance determines the inspiratory pressure needed to achieve a certain inspiratory airflow as well as how quickly the lungs empty during expiration.
Figure 2.2 The respiratory system (lungs plus chest wall) is a closed system during ventilation with a cuffed endotracheal tube. See Equation 1 for relationships among pressures. Pprox, pressure at proximal end of endotracheal tube; Paw, pressure drop across airways during inspiration as a result of resistance of artificial and natural airways; Palv, alveolar pressure (equal to the static recoil pressure of the respiratory system); Pb, barometric pressure. (Modified from Lanken PN: Mechanical ventilation. In Fishman AP [ed]: Pulmonary Diseases and Disorders, 2nd ed. New York: McGraw-Hill, 1988.)
As a rule, when adults are being mechanically ventilated via an endotracheal tube or tracheostomy tube, the respiratory system is a “closed” system and has no leaks (see Figure 2.2). Under these circumstances, the following relationship holds during inspiration:
where Pprox is the pressure at the proximal end of the artificial airway during inspiration; Paw, the pressure drop across the airways during inspiration; R(airways), airway resistance; flow, the inspiratory flow rate; and Palv, the alveolar pressure during inspiration.
Equation 1 indicates that the proximal airway pressure during inspiration is the sum of two components: (1) a dynamic component, Paw, representing the pressure drop that occurs as air flows into the lungs (as a result of the resistance of artificial and natural airways), and (2) a static component, Palv, the mean pressure in alveoli, which represents the static recoil pressure of the lungs and chest wall. This pressure is determined by the increase in lung volume above functional residual capacity (FRC), the volume in the lungs at end expiration.
Pressure-Volume Curves of the Respiratory System
Static Pressure-Volume Curve
As noted earlier, changes in lung volume above FRC during inspiration relate to the recoil pressure of the respiratory system (Figure 2.3A). The compliance of the respiratory system incorporates both lung compliance and chest wall compliance and is expressed as follows:
Figure 2.3 Schematic Pressure-Volume (P-V) Curves of the Respiratory System, with the Static Recoil Pressure of the Respiratory System (Palv in Figure 2.2) as Abscissa. A, Static P-V curve. A tidal volume of 1000 mL results in recoil pressure of 10 cm H2O. Because compliance of the respiratory system (Cstat) is equal to the change in volume (V) above functional residual capacity (FRC) divided by the change in pressure (
P), Cstat = (1000 mL/10 cm H2O) = 100 mL/cm H2O (a normal value). Note that the curve flattens at higher pressures as total lung capacity is approached. B, Dynamic P-V curve of 1000 mL tidal volume superimposed on the static P-V curve in A. The difference between the two curves (
Paw) represents the pressure drop across the airways resulting from airway resistance. Note that peak pressure (Ppeak) exceeds the end-inspiratory static pressure (plateau pressure or Pplat) by ∼2 cm H2O, reflecting normal airway resistance. (Modified from Lanken PN: Mechanical ventilation. In Fishman AP [ed]: Pulmonary Diseases and Disorders, 2nd ed. New York: McGraw-Hill, 1988.)
where Cstat is the static compliance of the respiratory system, V is a change in volume, and
P is the corresponding change in pressure.
Dynamic Pressure-Volume Curve
The static pressure-volume (P-V) curve of the respiratory system, discussed earlier, applies only when there is no airflow—that is, at end inspiration or end expiration. When air is flowing into or out of the lung, resistance to airflow comes into play as can be seen by the patient’s dynamic P-V curves (see Equation 1 and Figure 2.3B).
Basic Traditional Modes of Ventilation
Assist/Control Mode
Settings
The control mode is straightforward because the operator sets only two primary parameters: respiratory rate and tidal volume (Figure 2.4A). Most ventilators function as volume-cycled ventilators, in which inspiration ceases after the preset tidal volume is delivered to the patient. Unless there is a leak in the system, such as around the cuff of a tracheal tube or through a chest tube, or unless peak inspiratory pressure exceeds the “pop-off” pressure (the threshold set for the peak pressure alarm [see Chapter 47]), the patient should receive all of the preset tidal volume. The combination of rate and tidal volume, therefore, defines the minute ventilation that the patient receives (Table 1.1).
The assist mode adds two features of operation to the control mode (Figure 2.4B). First, the ventilator can detect a patient’s inspiratory effort (by detecting the negative pressure deflection from baseline in the inspiratory circuit or by detecting the beginning of patient-initiated airflow through the ventilator’s demand valve). When that inspiratory effort exceeds a certain threshold, the patient “triggers” the ventilator to begin delivery of the preset inspiratory tidal volume. The timing of the start of the next inspiration is defined by the set respiratory rate or by the patient’s spontaneous rate, whichever is higher. For example, if the preset rate is 10 breaths/min, the ventilator provides a tidal volume every 6 seconds unless it detects the patient’s spontaneous inspiratory effort earlier. If it does, it resets the start of the next ventilator-initiated breath to be 6 seconds from the start of the patient-initiated breath.
The second additional feature of the assist mode allows the patient to inspire from the ventilator’s demand valve (which is similar to the demand valve of SCUBA [self-contained underwater breathing apparatus] gear). This occurs during inspiration if the patient’s inspiratory flows exceed those generated by the ventilator or if the patient continues to inspire after the preset tidal volume has been delivered. In this manner, the patient’s actual tidal volumes can be consistently larger than the ventilator’s set tidal volume (see Figure 2.4B). On occasion, patients breathing vigorously can also set off the ventilator’s low inspiratory pressure alarm by continually inspiring during machine inspiration (see Chapter 47 for more information about alarms).
Mechanical Considerations
In volume-cycled ventilation, such as in A/C mode, the delivered tidal volume is less than the preset tidal volume if the peak inspiratory pressure (PIP) exceeds the threshold for the peak pressure alarm. For any given tidal volume and inspiratory flow rate, PIP is determined by two factors relating to the patient’s mechanics (as expressed in Equations 1 and 2): airway resistance and static compliance of the respiratory system. If the PIP exceeds the threshold for the ventilator’s peak pressure alarm, the remaining tidal volume is “dumped” (allowed to escape from the system) and is not delivered to the patient. This may occur if the patient actively resists the machine-delivered inspiration (termed bucking or fighting the ventilator) or coughs (both of which decrease compliance of the respiratory system). Another common cause of high PIPs is if the patient’s airway resistance increases, such as in an airway that is partially blocked by respiratory secretions or by the patient biting the endotracheal tube.
Clinical Considerations
In the case of patients who have high respiratory rates while on A/C mode, one must first check that the ventilator’s triggering sensitivity is not excessive (which could lead to ventilator self-cycling and high respiratory rates). One can address the problem of ventilated patients who continue to make strong inspiratory efforts on A/C mode by increasing inspiratory flow rates and checking for auto-PEEP (auto–positive end-expiratory pressure, described later). If auto-PEEP is present, it should be treated as discussed later. If the tachypnea persists, one may need to suppress the patient’s high respiratory drive pharmacologically, such as with more sedation (see Chapter 5), in order to provide a respite for the patient’s respiratory muscles. Changing to the PS mode of ventilation is another alternative.

Full access? Get Clinical Tree
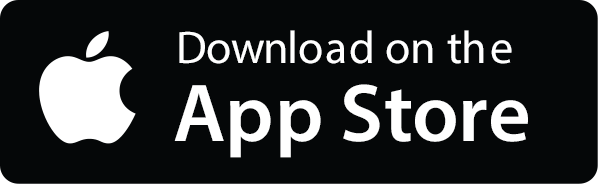
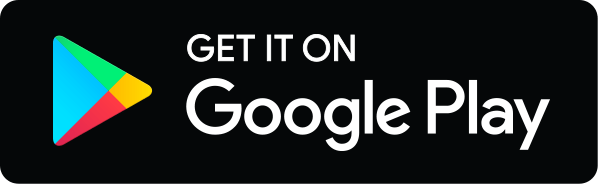