The goal of pharmacologic treatment of epilepsy is to control seizures with minimal medication-related adverse effects. Approximately 70% of patients with epilepsy will become seizure-free using a single antiepileptic drug. For the remaining 30% of patients, further treatment options may include transitioning to another drug; combining the primary drug with an additional drug; or upon failure of medical therapy, progression to invasive procedures such as vagal nerve stimulator insertion or neurosurgical resection.2 The antiepileptic drug selected to treat epilepsy is highly individualized and tailored to the individual patient, explaining the high interpatient variability in drug regimens. Criteria that must be considered in choosing an antiepileptic drug include efficacy for the characteristic seizures experienced by the patient, tolerability, safety, ease of use and frequency of administration, and pharmacokinetics (Table 13-2).2 Over the last two decades, there has been a dramatic increase in drug choices, which offer markedly fewer side effects with often comparable efficacy to older drugs.3 However, dose-related side effects can limit the use of any of the antiepileptic drugs (Table 13-3). Although side effects are normally associated with higher plasma levels of the drug, the specific concentration at which a patient develops toxicity varies considerably (Table 13-4).4




Pharmacokinetics
All antiepileptic drugs are administered once daily or more frequently. Sustained-release preparations are becoming increasingly available and preferred by patients. Absorption of these drugs from the gastrointestinal tract occurs slowly over a period of hours and may be incomplete, especially for gabapentin. Protein binding varies greatly (0% for gabapentin to 90% or greater for phenytoin). Hepatic and renal disease may necessitate dose adjustment. Medications that rely on renal excretion include gabapentin, pregabalin, levetiracetam, vigabatrin, and zonisamide and should be dosed according to renal function. The remaining drugs should be dosed according to the patient’s degree of liver dysfunction.
Antiepileptic drug clearance and elimination half-time range from hours (carbamazepine, valproate, primidone, gabapentin) to days (phenytoin, lamotrigine, phenobarbital, zonisamide) (see Table 13-4). Because of their ability to induce or inhibit drug metabolism, all antiepileptic drugs, except gabapentin, levetiracetam, and vigabatrin, may be associated with pharmacokinetic drug interactions in which plasma drug concentrations and resulting pharmacologic effects of concomitantly administered drugs may be altered. Such drug interactions should be anticipated in all patients receiving antiepileptic drugs and subsequently receiving drugs for other purposes.
Drug Interactions Related to Protein Binding
Medications that compete for protein-binding sites of highly bound antiepileptic drugs (phenytoin, valproate, carbamazepine) can displace the bound drug and lead to increases in the plasma concentration of pharmacologically active antiepileptic drug. Commonly used medications that are highly protein bound include phenylbutazone, thyroxine, and salicylates. Albumin is the principal binding protein for antiepileptic drugs. Hypoalbuminemia, as may accompany renal or hepatic disease or malnutrition, can result in increased plasma concentrations of unbound antiepileptic drug, resulting in toxicity despite therapeutic plasma concentrations. In pregnancy, hypoalbuminemia is due to a progressive increase in central volume which offsets the effect of hypoalbuminemia.
Drug Interactions Related to Accelerated Metabolism
Enzyme-inducing antiepileptic drugs that accelerate metabolism (carbamazepine, lamotrigine, oxcarbazepine, phenobarbital, phenytoin, topiramate, and primidone) may accelerate the metabolism of estrogen and progesterone and thus render oral contraceptives ineffective at usual doses. Patients being treated with antiepileptic drugs have increased dose requirements for thiopental, propofol, midazolam, opioids, and nondepolarizing neuromuscular blocking drug. Possible explanations for altered dose requirements for drugs administered during anesthesia include increased hepatic P450 enzyme activity as a result of enzyme-inducing effects of antiepileptic drugs, alterations in the number and/or responsiveness of receptors, and interactions with endogenous neurotransmitters.
Principles of Dosing
The initial dose is that which is high enough to expect clinical effect but low enough to avoid significant side effects (see Table 13-3). Gradual dose titration is recommended in all but emergency situations. The clinical response guides dose adjustment over time as there is significant variability in clinical response over a wide range of dosages. A common cause of medication ineffectiveness is failure to achieve a sufficiently high plasma concentration. Noncompliance is a particular concern in specific patient populations including adolescents and the elderly.1,2,4
To maintain plasma drug concentrations in a therapeutic range, equal doses of the antiepileptic drug are often administered at intervals equivalent to less than one elimination half-time of the drug (see Table 13-4). Dosing at one-half the drug’s elimination half-time ensures that a single missed dose will not result in the plasma concentration decreasing below a therapeutic level.
Plasma Concentrations and Laboratory Testing
Phenytoin is the only agent for which monitoring is routinely recommended due to its nonlinear saturation dose kinetics. Routine laboratory monitoring of plasma concentrations for all other agents is not recommended.2 For this reason, titration to clinical efficacy is recommended for guiding the dosages of antiepileptic drugs. Some patients may respond at low plasma concentrations, and some patients will not respond until high plasma concentrations are obtained. If a patient does not respond to a particular drug as expected, investigating the plasma drug concentration may aid in determining compliance and identifying potential pharmacokinetic interactions.2,4
Mechanism of Seizure Activity
Seizure activity in most patients with epilepsy has a localized or focal origin. The reason for the high frequency and synchronous firing in a seizure focus is usually unknown. Possible explanations include local biochemical changes, ischemia, loss of cellular inhibitory systems, infections, and head trauma.
Neurons in a chronic seizure focus exhibit a type of denervation hypersensitivity with regard to excitatory stimuli. The spread of seizure activity to neighboring normal cells is presumably restrained by normal inhibitory mechanisms. Factors such as changes in blood glucose concentrations, PaO2, PaCO2, pH, electrolyte balance, endocrine function, stress, and fatigue may facilitate the spread of a seizure focus into areas of the normal brain. If the spread is sufficiently extensive, the entire brain is activated and a tonic-clonic seizure with unconsciousness ensues. Conversely, if the spread is localized, the seizure produces signs and symptoms characteristic of the anatomic focus. Once initiated, a seizure is most likely maintained by reentry of excitatory impulses in a closed feedback pathway that may not even include the original seizure focus.
Mechanism of Drug Action
The mechanism of action of antiepileptic drugs is incompletely understood. It is commonly presumed that antiepileptic drugs control seizures by decreasing neuronal excitability or enhancing inhibition of neurotransmission. This is achieved by altering intrinsic membrane ion currents (sodium, potassium, and calcium conductance) or by affecting activity of inhibitory neurotransmitters. Ion currents affected by antiepileptic drugs are primarily those involving the voltage-gated sodium and calcium ion channels. Drugs that delay reactivation of sodium channels (phenytoin, carbamazepine, primidone, valproate, and lamotrigine) during high frequency neuronal firing produce an inhibitory effect on creation of action potentials until neuronal discharge is blocked. Some drugs (phenytoin, carbamazepine, valproate, lamotrigine, and zonisamide) act at both sodium and calcium ion channels. Other drugs (ethosuximide and phenobarbital) act selectively at calcium ion channels. Ethosuximide selectively blocks the T-type calcium ion current, which is thought to act as a pacemaker for thalamic neurons and may be important in absence seizures. Drugs (phenobarbital and benzodiazepines) that alter synaptic function act primarily by enhancing γ-aminobutyric acid (GABA)–mediated neuronal inhibition. Benzodiazepines increase the frequency of GABA-mediated ion channel openings, whereas barbiturates increase the duration of ion channel openings. Tiagabine delays the reuptake of GABA from synaptic clefts, effectively enhancing GABA-mediated neuronal inhibition after synaptic release of the neurotransmitter.
Major Antiepileptic Drugs
The principal antiepileptic drugs used to treat patients with epilepsy are carbamazepine, ethosuximide, pregabalin, gabapentin, clobazam, lamotrigine, levetiracetam, oxcarbazepine, phenobarbital, phenytoin, primidone, tiagabine, topiramate, valproate, and zonisamide (see Table 13-2). Since 2005, the number of agents has more than doubled, thereby offering broader therapeutic effectiveness with fewer drug interactions, broader spectrums of activity, and unique mechanisms of action (see Table 13-2) (Fig. 13-1).5

Benzodiazepines such as diazepam, lorazepam, and midazolam are used for short-term treatment of acute seizures or status epilepticus and are usually administered parenterally. Clonazepam can be used to treat epilepsy but most patients develop tolerance to its antiepileptic effects and sedation is a common side effect. Felbamate is reserved for use in selected patients with uncontrolled seizures due to its side effect profile. Clobazam, a benzodiazepine derivative, is a more recent addition to this class and is unique among other members of the class in that it does not induce significant levels of sedation and can be used for long-term therapy because tolerance is relatively uncommon.6
Drugs used in the treatment of partial seizures are carbamazepine, lamotrigine, oxcarbazepine, topiramate, zonisamide and phenytoin, which are highly effective and associated with an acceptable side effect profile (see Table 13-2). Valproate, lamotrigine, and topiramate are the antiepileptic drugs useful for treatment of patients with generalized seizures. Ethosuximide, lamotrigine, or valproate is effective in treatment of patients with generalized nonconvulsive seizures, especially absence seizures.
Adverse Side Effects
Antiepileptic drugs may potentially produce numerous and varied adverse side effects. Newer agents have a significantly more favorable side effect profile. Some adverse side effects are dose-related (sedation, lethargy, neurotoxicity), whereas others are idiosyncratic (hypersensitivity, hepatotoxicity, aplastic anemia) (see Table 13-3).
Maternal Epilepsy
As previously mentioned, pregnancy can result from enzyme inducing antiepileptic drugs that render oral contraceptive pills less effective. Seizures during pregnancy can result in significant morbidity and mortality to both mother and fetus, making seizure control during this period imperative.2 Monotherapy with the lowest dose possible is the guiding principle. Fetal organogenesis is largely complete by 8 weeks. Significant teratogenicity may occur during this period if pregnancy is not detected early enough to permit discontinuation of potentially teratogenic medications. Drug regimens in women of childbearing age should therefore be given special attention. In particular, parturients who take valproate and carbamazepine have more than double the risk of giving birth to a fetus with congenital malformations including neural tube defects such as spina bifida. Patients on lamotrigine have rates of congenital malformation comparable to the general population. Clobazam may be added as needed especially during labor. Conclusive data regarding other antiepileptic drugs during pregnancy is lacking, in part due to the ethical and regulatory difficulty of conducting randomized trials during pregnancy.1
Carbamazepine
Carbamazepine is an iminostilbenes derivative that is effective for suppression of nonconvulsive and convulsive partial seizures. In addition, this drug is useful in the management of patients with trigeminal neuralgia and glossopharyngeal neuralgia. Structurally, carbamazepine is related to the tricyclic antidepressant imipramine. Like phenytoin, carbamazepine alters ionic conductance and thus has a membrane-stabilizing effect.
Pharmacokinetics
This drug is available only as an oral preparation (see Table 13-4). Oral absorption is rapid, with peak plasma concentrations occurring 2 to 6 hours after ingestion. Plasma protein binding is 70% to 80%. The plasma elimination half-time is 8 to 24 hours. The principal metabolite of carbamazepine is an epoxide derivative that has antiseizure effects that may also be responsible for many of the dose-limiting side effects of this drug. Because this drug induces its own metabolism, many patients require a dosage increase in 2 to 4 weeks after initiation of therapy. The usual therapeutic plasma concentration of carbamazepine is 6 to 12 µg/mL.
Side Effects
The toxicity of carbamazepine is similar to that produced by phenytoin (see Table 13-3). Sedation, vertigo, diplopia, nausea, and vomiting are the most frequent side effects of this drug. Chronic diarrhea develops in some patients, whereas others experience the syndrome of inappropriate antidiuretic hormone secretion. Aplastic anemia, thrombocytopenia, hepatocellular and cholestatic jaundice, oliguria, hypertension, and cardiac dysrhythmias are rare but potentially life-threatening complications. Chronic suppression of white blood cell counts can occur. For these reasons, it may be prudent to monitor bone marrow, cardiac, hepatic, and renal function in patients being treated with carbamazepine. At high plasma concentrations, carbamazepine has an arginine vasopressin hormone-like action that may result in hyponatremia. Skin rash, often with other manifestations of drug allergy, occurs in approximately 10% of chronically treated patients.
In addition to inducing its own metabolism, carbamazepine can accelerate the hepatic oxidation and conjugation of other lipid-soluble drugs. The most common interaction is with oral contraceptive pills, and most women require an increase in the daily dose of estrogen. Carbamazepine also accelerates the metabolism of valproic acid, ethosuximide, corticosteroids, anticoagulants, and antipsychotic drugs. Drugs that inhibit the metabolism of carbamazepine sufficiently to cause toxic effects include cimetidine, propoxyphene, diltiazem, verapamil, isoniazid, and erythromycin.
Ethosuximide
Ethosuximide is the drug of choice for suppression of absence (petit mal) epilepsy in patients who do not also have tonic-clonic seizures. This drug acts by decreasing voltage-dependent calcium conductance in thalamic neurons. This is consistent with the speculated importance of the thalamocortical system in the etiology of absence seizures.
Pharmacokinetics
This drug is available only as an oral preparation (see Table 13-4). Peak plasma concentrations occur in 1 to 7 hours after oral administration. Ethosuximide is not significantly bound to albumin. Approximately 25% of the drug is excreted unchanged in urine, and the remainder is metabolized to inactive metabolites by hepatic microsomal enzymes. The elimination half-time is 20 to 60 hours. The usual maintenance dose of ethosuximide is 20 to 30 mg/kg. A plasma concentration of 40 to 100 µg/mL is required for satisfactory suppression of absence epilepsy.
Side Effects
Toxicity of ethosuximide is low, manifesting most often as gastrointestinal intolerance (nausea, vomiting) and CNS effects (lethargy, dizziness, ataxia, photophobia). There have been rare reports of bone marrow suppression.
Felbamate
Because of its potential to produce life-threatening side effects, felbamate is not used as a first-line drug for treatment of seizures but rather is reserved for patients with intractable epilepsy. Felbamate is used principally for poorly controlled partial and secondarily generalized seizures. It also decreases the frequency of seizures associated with the Lennox-Gastaut syndrome and myotonic and atonic forms of epilepsy.7 The mechanism of action of felbamate is unknown but may involve action at voltage-gated sodium channels, NMDA and non-NMDA glutamate receptors, voltage-gated calcium currents, and GABA receptor modulation.6
Pharmacokinetics
Oral absorption is prompt and the elimination half-time is prolonged (see Table 13-4). Felbamate undergoes minimal metabolism with most of the drug being excreted unchanged by the kidneys.
Side Effects
Serious side effects include aplastic anemia and hepatotoxicity (see Table 13-3). Monitoring of treated patients with complete blood counts and liver function tests is indicated. Because felbamate is metabolized by hepatic cytochrome P450 enzymes, its metabolism is affected by concurrent administration of other drugs that are also metabolized by this system. In particular, concomitant administration of carbamazepine or phenytoin may decrease plasma concentrations of felbamate. Likewise, since felbamate is a potent inhibitor of P450 enzymes, it can slow the metabolism of phenytoin, phenobarbital, and valproic acid. In this regard, if a patient is receiving phenytoin, carbamazepine, or valproic acid and receives felbamate, the dose of these drugs should be decreased by 20% to 30% to prevent toxic effects.
Gabapentin
The pharmacokinetic considerations for gabapentin are discussed in detail in Chapter 8. Gabapentin is an analog of GABA that increases synaptic GABA considerations. Gabapentin induces dose-related sedation and it has efficacy in the treatment of anxiety, panic, and major depression.8 Despite its multiple other uses, gabapentin has limited efficacy in the treatment of epilepsy.
Lamotrigine
Lamotrigine is a chemically novel anticonvulsant drug of the phenyltriazine class that most likely acts by stabilizing voltage-sensitive sodium ion channels, thus preventing release of aspartate and glutamate. This drug has a broad spectrum of activity and is effective when used alone or in combination in adults who have partial seizures or generalized seizures and in children with Lennox-Gastaut syndrome. When administered orally, lamotrigine is well absorbed and its plasma elimination half-time is about 25 hours (see Table 13-4). Drugs that induce hepatic microsomal enzymes (phenobarbital, phenytoin, and carbamazepine) decrease the elimination half-time of lamotrigine by about 50%, necessitating a higher dose. Conversely, valproic acid slows the metabolism of lamotrigine and extends its elimination half-time to about 60 hours. The most common side effects of lamotrigine are headache, nausea, vomiting, dizziness, diplopia, and ataxia (see Table 13-3). Tremor can be troublesome at higher doses. In approximately 5% of adults, a rash develops, which subsequently disappears in some patients, despite continued therapy. In a few patients, however, the rash is more serious, and fever, arthralgias, and eosinophilia occur. In rare cases, Stevens-Johnson syndrome has been reported.
Levetiracetam
Levetiracetam is effective in the management of partial-onset seizures in adults. Its mechanism of action is not fully known; however, it binds to certain presynaptic calcium channels, acting to reduce synaptic neurotransmitter release.9 Side effects are considered minor and include sedation, asthenia, anxiety, and headache. The pharmacokinetic profile of levetiracetam is favorable, with the absence of hepatic metabolism and minimal protein binding. No significant drug interactions have been described with coadministration of other antiepileptic drugs.
Oxcarbazepine
Oxcarbazepine is a keto analogue of carbamazepine that provides equivalent seizure control but with fewer adverse side effects. After administration, oxcarbazepine acts as a prodrug that is converted to an active metabolite, 10-hydroxycarbazepine. Oxcarbazepine and its active metabolite do not induce hepatic microsomal enzymes nor does it displace other drugs from plasma protein-binding sites. As such, they are safer drugs to be used in combination therapy. Oxcarbazepine causes dose-dependent hyponatremia in up to half of patients, mandating monitoring of serum sodium levels at those receiving higher doses of this agent.
Phenobarbital
Phenobarbital is a long-acting barbiturate that is effective against all seizure types except nonconvulsive primary generalized seizures. Cognitive and behavioral side effects limit this drug’s usefulness in the treatment of epilepsy. Because of these side effects, phenobarbital is considered a second-line drug in the treatment of epilepsy.
Phenobarbital appears to exert its antiepileptic properties partly through potentiation of the postsynaptic actions of the inhibitory neurotransmitter GABA and inhibition of the excitatory postsynaptic actions of glutamate. These drug-induced effects prolong the duration of chloride channel opening and thus limit the spread of seizure activity and increase the seizure threshold.
Pharmacokinetics
Oral absorption of phenobarbital is slow but nearly complete, with peak concentrations occurring 12 to 18 hours after a single dose (see Table 13-4). Plasma protein binding is 48% to 54%. Approximately 25% of phenobarbital is eliminated by pH-dependent renal excretion, with the remainder inactivated by hepatic microsomal enzymes. The principal metabolite is an inactive parahydroxyphenyl derivative that is excreted in urine as a sulfate conjugate. The elimination half-time of phenobarbital is prolonged.
The usual daily oral dose of phenobarbital is 60 mg in adults or 4 mg/kg in children. Plasma phenobarbital concentrations of 10 to 40 µg/mL are usually necessary for control of seizures. The value of measuring plasma phenobarbital concentrations is limited because the concentration associated with optimal control is highly variable among patients. In addition, the development of tolerance to the drug’s CNS effects makes the toxic threshold imprecise.
Side Effects
Sedation in adults and children and irritability and hyperactivity in children are the most troublesome side effects when this drug is used to treat epilepsy (see Table 13-3). Tolerance to the sedative effects of phenobarbital may develop with chronic therapy. Depression develops in many adults taking phenobarbital, and confusion may occur in elderly patients. Cognitive effects include slowing of task processing. Scarlatiniform or morbilliform rash occurs in up to 2% of patients. Megaloblastic anemia that responds to folic acid administration and osteomalacia that responds to vitamin D therapy may occur during chronic phenobarbital therapy as well as during treatment with phenytoin. Nystagmus and ataxia are likely if the plasma phenobarbital concentration is >40 µg/mL. Abnormal collagen deposition manifesting as Dupuytren contracture may occur. Congenital malformations may occur when phenobarbital is administered chronically during pregnancy. Coagulation defects and hemorrhage in the neonate must be considered in the setting of fetal exposure. Interactions between phenobarbital and other drugs usually involve induction of hepatic microsomal enzymes. In this regard, phenobarbital is the classic example of a hepatic microsomal enzyme inducer that can accelerate the metabolism of many lipid-soluble drugs.
Phenytoin
Phenytoin is the prototype of the hydantoins and is effective for the treatment of partial and generalized seizures. Available in oral and intravenous (IV) preparations, phenytoin may be administered acutely to achieve effective plasma concentrations within 20 minutes. This drug has a high therapeutic index, and its administration is not accompanied by excessive sedation.
Mechanism of Action
Phenytoin regulates neuronal excitability and thus the spread of seizure activity from a seizure focus by regulating sodium and possibly calcium ion transport across neuronal membranes. This stabilizing effect on cell membranes is relatively selective for the cerebral cortex, although the effect also extends to peripheral nerves. In addition to the effect on ion fluxes, phenytoin acts on second messengers such as calmodulin and the cyclic nucleotides.
Pharmacokinetics
Phenytoin is a weak acid (pK 8.3) that is maintained in aqueous solutions as a sodium salt (see Table 13-4). The drug precipitates in solutions with a pH of <7.8. Its poor water solubility may result in slow and sometimes variable absorption from the gastrointestinal tract (30% to 97%). The initial daily adult oral dosage is 3 to 4 mg/kg. Doses of >500 mg daily are rarely tolerated. The long duration of action of phenytoin allows a single daily dosage, but gastric intolerance may necessitate divided dosage. After intramuscular (IM) injection, the drug precipitates at the injection site and is slowly absorbed. For this reason, IM administration is not recommended. The rate of IV administration of phenytoin should not exceed 50 mg per minute in adults and 1 to 3 mg/kg per minute (or 50 mg per minute, whichever is slower) in pediatric patients because of the risk of severe hypotension and cardiac arrhythmias.
Plasma Concentrations
Control of seizures is usually obtained when plasma concentrations of phenytoin are 10 to 20 µg/mL. In the control of digitalis-induced cardiac dysrhythmias, phenytoin, 0.5 to 1.0 mg/kg IV, is administered every 15 to 30 minutes until a satisfactory response is achieved or a maximum dose of 15 mg/kg is administered. A plasma phenytoin concentration of 8 to 16 µg/mL is usually sufficient to suppress cardiac dysrhythmias. Adverse side effects of phenytoin such as nystagmus and ataxia are likely when the plasma concentration of drug is >20 µg/mL. Nevertheless, the diagnosis of phenytoin toxicity should be made on the basis of clinical symptoms.
Protein Binding
Phenytoin is bound approximately 90% to plasma albumin. A greater fraction of phenytoin remains unbound in neonates, in patients with hypoalbuminemia, and in uremic patients.
Metabolism
Metabolism of phenytoin to inactive metabolites is by hepatic microsomal enzymes that are susceptible to stimulation or inhibition by other drugs. An estimated 98% of phenytoin is metabolized to the inactive derivative parahydroxyphenyl, which appears in urine as a glucuronide. Approximately 2% of phenytoin is recovered unchanged in urine.
When the plasma concentration of phenytoin is <10 µg/mL, metabolism follows first-order kinetics, and the elimination half-time averages 24 hours. At plasma concentrations of >10 µg/mL, the enzymes necessary for metabolism of phenytoin become saturated, and the elimination half-time becomes dose-dependent (zero-order kinetics). At this stage, relatively small increases in dose may result in dramatic increases in the plasma concentration of phenytoin. Zero-order kinetics in phenytoin metabolism resembles the metabolism of alcohol.

Full access? Get Clinical Tree
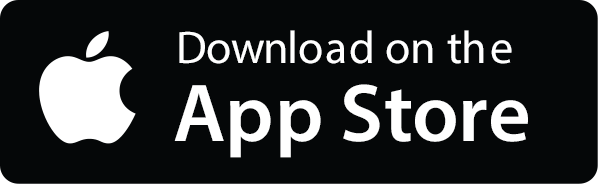
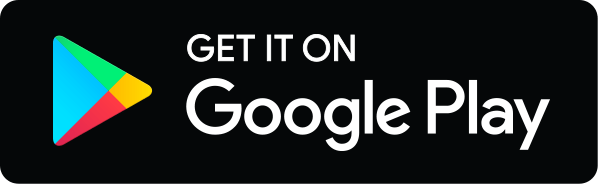