Anticoagulants are widely used in the perioperative period. The anesthesia consultant must have an understanding of their pharmacology and the implications of their use in a myriad of clinical scenarios. Intricate and complex processes that influence coagulation and anticoagulation are always in a dynamic state of equilibrium. The balance is shifted one way or the other by various disease states, medications, or perioperative events (ie, hemodilution with aggressive resuscitation). Anesthesiologists are often called upon to find a reasonable balance between anticoagulation and hemostasis in a variety of circumstances, such as during procedures associated with blood loss in patients at risk for arterial thrombotic events or when managing postoperative neuraxial analgesia in patients at high risk for deep vein thrombosis. The focus of this chapter is on elements of anticoagulant clinical pharmacology that are important for anesthesiologists to understand. Anticoagulation drugs represent a rapidly expanding field, with many novel agents in various stages of testing and approval. However, this chapter will be confined to classes of anticoagulants and antiplatelets in clinical use. A summary of these drugs is presented in Table 16–1.
Route | Indications | |
---|---|---|
ANTICOAGULANTS | ||
Antithrombin III Binding Agents | ||
Heparins | ||
Heparin. | IV, SC | DVT/PE prophylaxis Treatment for ACS, DVT, PE Anticoagulation for AF, CPB, ECHO Patency of indwelling venous catheters |
Low-molecular-weight heparin | ||
Enoxaparin (Lovenox, Clexane, Xaprin) | SC | DVT/PE prophylaxis Treatment of DVT, ACS |
Tinzaparin (Innohep) | IV, SC | DVT/PE prophylaxis (safe in pregnancy) |
Fondaparinux (Arixtra) | SC | DVT/PE prophylaxis |
Factor Xa Inhibitors | ||
Rivaroxaban (Xarelto) | Oral | DVT/PE prophylaxis Anticoagulation for nonvalvular AF Treatment for DVT/PE |
Direct Thrombin Inhibitors | ||
Lepirudin (Refludan) | IV | Heparin alternative for HIT |
Argatroban (Argatroban) | IV | DVT prophylaxis Treatment for DVT Heparin alternative for HIT |
Bivalirudin (Angiomax, Angiox) | IV | Anticoagulation for PCA Heparin alternative for HIT |
Dabigatran etexilate (Pradaxas, Pradax, Prazaxa) | Oral | CVA prevention in patients with AF |
Clotting Factor Synthesis Inhibitor | ||
Warfarin (Coumadin, Jantoven, Marevan, Warfant) | Oral | DVT/PE prophylaxis Anticoagulation for AF, antiphospholipid syndrome, and mechanical heart valves |
ANTIPLATELET AGENTS | ||
Cyclooxygenase Inhibitors | ||
Aspirin | Oral | MI and CVA prophylaxis |
Nonsteroidal anti-inflammatory drugs | IV, Oral | |
Thienopyridines (Adenosine Diphosphate Inhibitors) | ||
Clopidogrel (Plavix) Oral | Oral | Clot prevention for coronary artery, peripheral artery, and cerebral artery vascular disease Treatment for ACS, PCI |
Ticlopidine (Ticlid) | Oral | Similar to clopidogrel Associated with thrombotic thrombocytopenic purpura; supplanted by clopidogrel |
Prasugrel (Effient) | Oral | Treatment for ACS, PCI |
GpIIb/IIIa Inhibitors | ||
Abciximab (ReoPro) | IV, IC | Treatment for PCI |
Eptifibatide (Integrilin) | IV | Treatment for PCI Treatment of acute myocardial ischemic events or unstable angina |
Tirofiban (Aggrastat) | IV | Treatment for PCI Treatment of acute myocardial ischemic events or unstable angina |
Both unfractionated heparin (UH) and low-molecular-weight heparin (LMWH) produce anticoagulation indirectly by causing a conformational change in antithrombin III (ATIII), increasing its catalytic activity by 1000 fold.1,2 ATIII primarily inhibits the actions of factor IIa, thrombin, and Xa, as well as factors IXa, XIa, and XIIa to a lesser extent.3 There are 2 major factors that determine the activity of heparin: (1) polysaccharide chain length and (2) a specific 5-sugar (pentasaccharide) sequence within the heparin molecule.4 In general, inhibition by ATIII of both factors IIa and Xa occurs in response to UH. Low-molecular-weight heparins will selectively inhibit factor Xa via ATIII.1 The interaction with ATIII is mediated by a unique pentasaccharide sequence that is randomly distributed along the heparin molecule.5 This pentasaccharide sequence is present in 30% of the chains of UH and 15% to 22% of the chains of LMWH.1,6 Binding of the pentasaccharide sequence causes a conformational change in ATIII that accelerates its interaction with thrombin and activated factor X.
Heparin chain length partially determines the factors that ATIII inhibits. UH has chain lengths of 45 to 50 sugars, whereas LMWH has lengths of 13 to 22 sugars.1,4 Any pentasaccharide-containing chain can catalyze the inhibition of factor Xa simply by binding to ATIII and causing a conformational change. In contrast, to inactivate thrombin, heparin must bind to both antithrombin and thrombin, forming a ternary complex (3 proteins bound together). This complex can be formed only by pentasaccharide-containing chains composed of at least 18 saccharide units.6 Less than 50% of LMWH chains are of sufficient length to bind to both thrombin and ATIII. As a result, UH usually has an anti Xa-to-IIa ratio of 1:1, whereas LMWHs typically have a ratio of 2:1 to 4:1.1,3 Both LMWH and UH are inactive against fibrin-bound thrombin, and neither agent inhibits factor Xa bound to platelets within the prothrombinase complex.7
UH is parenterally administered either intravenously or subcutaneously. There are a variety of clinical scenarios requiring different dosing regimens of UH.8 Dosing is dependent upon the therapeutic goal. Primary uses of heparin include thrombosis prevention (lower doses) and anticoagulation (higher doses). Common dosing for thrombosis prophylaxis includes subcutaneous injection of 5000 to 7500 units every 8 to 12 hours. Higher doses are warranted (5000 units subcutaneously every 8 hours) in high-risk patients (eg, history of cancer, known hypercoagulopathy, anticipated prolonged postoperative course in a sedentary state). This may present a clinical conundrum in managing postoperative pain with indwelling epidural catheters (see Clinical Implications: Anticoagulants and Neuraxial Anesthesia).
Anesthesiologists are almost always called upon to administer heparin for cardiopulmonary bypass and frequently called upon to administer heparin for vascular and endovascular procedures. For cardiopulmonary bypass, a large bolus dose is administered (300 units/kg) to achieve an activated clotting time (ACT) greater than 400 seconds. Additional doses may be required to maintain the ACT at that level. For vascular procedures, even following consultation with surgical colleagues, a review of recent laboratory values for activated partial thromboplastin time (aPTT), international normalized ratio (INR), hemoglobin concentration, platelet count, consideration of the surgical procedure, and a careful review of the risk–benefit in this setting (eg, excessive blood loss versus vascular graft patency), intraoperative dosing is often variable.
Aspects of heparin use are perhaps beyond the scope of practice for anesthesiologists. Heparin is used to treat acute coronary syndromes, deep vein thromboses, pulmonary emboli, cerebrovascular accidents, and transient ischemic attacks. Initial dosing for acute coronary syndromes is a 60-unit/kg bolus followed immediately by a continuous infusion of 15 units/kg/min. Initial dosing for deep vein thromboses, pulmonary emboli, cerebrovascular accidents, and transient ischemic attacks is a 80-units/kg bolus followed immediately by an infusion of 18 units/kg/min.
UH is available in many concentrations ranging from 1 unit/mL to 10,000 units/mL. The wide variety in preparations makes it a high-risk medication, since significant harm may occur from either overdosing or underdosing.
Traditionally, a unit is defined as the amount needed to maintain 1 mL of citrated sheep’s plasma liquid for 1 hour after recalcification. It is now defined based on a reference antifactor Xa activity. In 2009, the United States Pharmacopeia (USP) changed the reference standard and potency assay for UH. The USP unit is harmonized with the International Unit (IU). The result is that a unit of UH is 10% less potent than the traditional definition. With large boluses, a larger heparin dose may be required. End points in monitoring should remain the same. The new preparation has an “N” after the lot number. This change was made to deal with the contamination crisis in 2007-2008.8
The kinetic profile of UH is unpredictable and dependent on multiple factors. Even with precise weight-based bolus and continuous infusion dosing, the extent of anticoagulation is variable. Some patients will be underdosed or overdosed, while others will be dosed appropriately.9 There is a high degree of protein binding as well as binding to endothelium and macrophages. Polysaccharide chain length plays a key role in the function of heparin and LMWH. The smaller the chain length, the less affinity a molecule has for plasma proteins. Lack of protein binding translates into a more predictable anticoagulant response and reduces or completely eliminates the need for laboratory monitoring.1
The high degree of protein binding has been a major drawback of UH. Many of these proteins are acute-phase reactants and vary markedly between patients. Other proteins, such as platelet factor 4 (PF4) and von Willebrand factor, are released from platelets when they are activated by thrombin. High concentrations of PF4 and von Willebrand factor at sites of vascular injury can neutralize UH and lower its effectiveness in the vicinity of thrombus.10,11 The high degree of protein binding of UH means that a loading dose is required to occupy these protein binding sites before effective anticoagulation through binding to ATIII can be achieved.2 The anticoagulant effect of UH is dependent both on dose and molecular size. It is not linear and increases disproportionately with increasing doses. With its smaller chain size, LMWH has much less binding to plasma proteins and a more predictable anticoagulant response, largely eliminating the need for laboratory monitoring.12
Heparin resistance, in which much higher than normal doses are required to achieve the desired effect, is relatively common. This can be due to the effects of protein binding as described above, variations in potency, increased clearance, or acquired or congenital antithrombin deficiency. Because heparin is dependent on ATIII for its effect, antithrombin deficiency can produce heparin resistance. For instance, this occurs when a patient has had a prolonged exposure to heparin as when the patient is awaiting a coronary artery bypass graft or after having an acute coronary syndrome. Antithrombin deficiency can be treated by giving fresh-frozen plasma or, with less exposure to the risks of blood products, ATIII concentrates.3
Because the relationship between a given dose and the degree of anticoagulation is unpredictable, and the harm from inadequate or excessive anticoagulation potentially severe, therapy is tailored by monitoring anticoagulant effect. The effect of UH can be monitored using the aPTT or ACT. The aPTT is a very sensitive test of heparin effect suited to lower plasma concentrations of heparin as is used in treatment of venous thromboembolism or acute coronary syndrome. The target aPTTs when managing a venous thromboembolism or an acute coronary syndrome are 60 to 80 seconds and 50 to 70 seconds, respectively.3 For much higher plasma concentrations used in cardiopulmonary bypass, the time required to measure a aPTT is excessive, so the ACT is used. An ACT of greater than 400 seconds is required prior to initiation of cardiopulmonary bypass.
In most instances, laboratory monitoring of LMWH is unnecessary because of a predictable antithrombotic response. In certain patients, however, monitoring of LMWH activity may be useful. For example, patients with renal failure experience an enhanced response to LMWHs because of dependence on renal clearance.1 LMWHs do not affect the traditional measures of clotting such as detected by the aPTT or prothrombin time (PT). The only available test to monitor LMWH activity is an anti-Xa level. Although anti-Xa is a reliable measure of antithrombotic effect, it is not a reliable measure of antihemostatic effect and is not predictive of bleeding risk.13 At the present time, there is no reliable and practical laboratory assay that accurately measures bleeding risk.
Clearance of UH is characterized as slower first-order kinetics, where mechanisms to break down heparin are quickly saturated. As the dose increases, the amount of available heparin to exert an effect increases in a nonlinear fashion. For example, for a dose of 25 U/kg the half-life is 25 minutes whereas for a dose of 400 U/kg the half-life is 150 minutes. The half-life of UH is reported as 60-90 minutes, but as the example suggests, the actual half-life is dose dependent. For smaller bolus doses, such as those often used in the operating room or interventional suite, the half-life is shorter. Bolus dosing may need to be repeated more frequently to achieve the desired effect.3
UH has the benefit of a rapid, reliable reversible agent in protamine sulfate. Protamine is a strong cationic substance derived from salmon sperm that interacts with the strong anionic substance, heparin, to form a stable salt. Each 100 units of heparin is neutralized by 1 mg of protamine. The dose required for reversing anticoagulation can be derived from the time course and dose of heparin but is more accurately accomplished with automated titration of the blood sample against different concentrations of protamine.
Protamine has a variety of potentially serious adverse effects, including systemic hypotension, elevations in pulmonary vascular resistance, and anaphylactoid reactions. In order to minimize the adverse effects as well as to match the slow return of protein-bound heparin to the circulation, it is recommended that protamine be given by slow intravenous infusion.
Protamine neutralizes the antithrombin activity of LMWH but only partially reverses (40%–70%) the antifactor-Xa activity. A dose of 1-mg protamine per 100 anti-Xa units reverses 90% of anti-IIa and 60% of anti-Xa activity. The clinical significance of the residual anti-Xa effect is unknown. Both anti-IIa and anti-Xa activity may return up to 3 hours after protamine reversal, which is thought to be due to release of additional LMWH from a tissue depot if administered subcutaneously.4
Heparin-induced thrombocytopenia (HIT) is an antibody-mediated hypercoagulable state with formation of thromboses in the venous and arterial systems; HIT is associated with a high degree of morbidity and mortality. Heparin can cause a mild early non–immune-mediated drop in platelet count that is clinically unimportant (formerly called type I HIT). In some patients treated with heparin for more than 4 days, antibodies form to the complex of heparin and PF4 on the surface of platelets. In a subset of patients, the antibodies are functionally active, causing the degranulation of procoagulant substances, strong platelet activation and thrombin formation. HIT can occur with any type of heparin at any dose but is more commonly associated with unfractionated heparin, longer duration of exposure, surgical rather than medical patients and female gender.14,15
Diagnosis of HIT is based on clinical and laboratory criteria. Clinical criteria include thrombocytopenia, timing, thrombosis, and other causes of thrombocytopenia (known as the 4 Ts). HIT is suspected with (1) a drop in platelet count by 50% or more than 20 × 109 cells/L from baseline for heparin therapy that exceeds 5 to 10 days or (2) an immediate drop in platelets with a recent exposure to heparin. It is also suspected in the presence of venous or arterial thrombosis or skin necrosis or in the absence of a clear reason for a fall in platelets. Diagnostic testing is done with enzyme-linked immunosorbent assay (ELISA), for presence of the heparin–PF4 antibody, and platelet activation tests, which are highly sensitive and specific, respectively. The presence of an antibody can often be present without HIT, so a confirmatory functional test such as the serotonin release assay or platelet aggregation assay should be performed. Heparin/PF4 antibodies typically drop to minimal or undetectable levels after 100 days and require several days of heparin reexposure to become clinically significant.16 When the diagnosis of HIT is made, all forms of heparin and LMWH at any dose should be discontinued. The patient has a coagulation risk in excess of the initial indication for heparin and requires another form of immediate-acting anticoagulation. This is usually accomplished with a direct thrombin inhibitor or anti-Xa medication. These agents will be discussed in a subsequent section of this chapter.
Safe cardiopulmonary bypass is dependent on a reliable, effective, and testable method of anticoagulation that can be returned to a normal state of coagulation within a reasonable time. UH is ideally suited to this application. Using UH may put the patient at risk, and this represents a unique anticoagulation challenge. There are circumstances in which a discreet reexposure to heparin has a favorable risk–benefit profile and other circumstances in which alternative strategies are preferred. The American College of Chest Physicians has published practice guidelines that provide an evidence-based approach to this difficult situation.

Full access? Get Clinical Tree
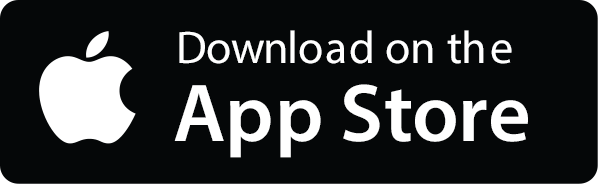
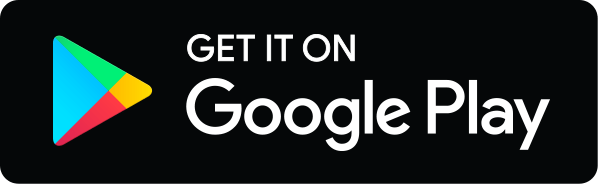