A major concern within the specialty of anesthesiology for many years has been the impact of general anesthesia and sedative drugs on neurodevelopment and cognition across the life span. Although definitive conclusions cannot be made, anesthesia providers should follow the progress of our knowledge regarding longer-term effects of anesthesia on the brain. For sure, neuronal cell death and neurocognitive impairments after general anesthesia have been unequivocally demonstrated in laboratory animal models. This public health concern has prompted the Food and Drug Administration to issue a Drug Safety Communication “warning that repeated or lengthy use of general anesthetic and sedation drugs during surgeries or procedures in children younger than 3 years of age or in pregnant women during their third trimester may affect the development of children’s brains” (also see Chapter 34 ). However, this is not a new concern. In 1953, Eckenhoff warned about an abnormal incidence of postoperative personality changes in children. Since then, preclinical reports on juvenile animal models unequivocally demonstrate a causal effect of general anesthesia on subsequent neurotoxic and neurocognitive dysfunction. In addition, in 1955 Bedford wrote about behavioral changes in the elderly after general anesthesia. Laboratory reports from several groups have found that anesthetic drugs induce histologic, biochemical, and neurocognitive deficits in mature rodents.
Anesthetic drugs are potent modulators of the central nervous system (CNS) and reversibly render patients insensate to painful procedures and surgery. Although the exact molecular mechanisms that produce immobility, analgesia, and amnesia are unknown, most anesthetic and sedative drugs are either γ-aminobutyric acid (GABA) receptor agonists, N -methyl- d -aspartate (NMDA) glutamate receptor antagonists, or a combination of the two. General anesthesia and sedation can be achieved by inhaled or intravenous administration of specific drugs. Both GABA agonists and NMDA antagonists have been implicated in causing anesthetic-induced developmental neurotoxicity (AIDN). Both the short-term and the long-term neurocognitive effects of general anesthesia should be considered.
Anesthetic Drugs as a Cause for Neurodegeneration and Long-Term Neurocognitive Deficits
Basic Science of Anesthetic-Induced Developmental Neurotoxicity
Determining the root cause of the neurotoxic effect of CNS depressant drugs on the developing brain is complicated by the myriad of molecular targets and the still unknown mechanistic pathway to achieve general anesthesia. AIDN has been demonstrated in laboratory models, both in vivo and in vitro, by exposure to most anesthetic and sedative drugs commonly administered to pediatric patients (also see Chapter 34 ). A comparable pattern of neurodegeneration and impaired neurocognitive development has been described with the perinatal administration of alcohol and anticonvulsant drugs. AIDN was first described more than 40 years ago in fetal and postnatal rats exposed to halothane, but its impact was not fully publicized to both the scientific and lay community until a 1999 report that emphasized that ketamine increased neurodegeneration in neonatal rat pups. Subsequently, it was found that the combination of commonly used anesthetic drugs—isoflurane, nitrous oxide, and midazolam—not only induced neuroapoptosis but resulted in deficits in hippocampal synaptic function and learning behavior. Although the anesthetic mechanisms of NMDA antagonists (ketamine and nitrous oxide) and GABA agonists (isoflurane, sevoflurane, desflurane, propofol, and midazolam) are divergent, preclinical reports clearly demonstrate neurodegenerative and neurocognitive changes in animal models.
Anesthesia removes sensory input and suppresses normal neural traffic, which in turn diminishes the trophic support required for neurogenesis and context-dependent modulation of neuroplasticity. However, several reports have described neuronal cell death mechanisms such as excitotoxicity, mitochondrial dysfunction, aberrant cell cycle reentry, trophic factor dysregulation, and disruption of cytoskeletal assembly. Although GABA acts as an inhibitory drug in the mature brain, it is an excitatory agent during early stages of brain development, owing to the preponderance of the immature Na/K/2Cl transporter protein NKCC1, which produces a chloride influx leading to neuron depolarization. Therefore, GABA remains excitatory until the GABA receptors are switched to the normal inhibitory mode when the mature chloride transporter, KCC2, is expressed, which actively transports chloride out of the neural cell.
Age-Dependent Vulnerability of Anesthetic
Neural development progresses through several steps that include neurogenesis, neuronal morphogenesis, and synaptogenesis. Neurogenesis starts with the creation of progenitor cells, which proliferate and differentiate into neurons or glial cells. Then oligodendrocytes and astrocytes appear, which serve as supporting cells for neurons. This proliferative (cell cycle) stage produces an overabundance of progenitor cells that develop into neural and glial cells. These cells can exit the cell cycle at different times to assume specific functions. A subpopulation remains undifferentiated and remains in the cell cycle. As neurons undergo terminal differentiation into a postmitotic state, they can no longer replicate. Dendrites and axons extend from the cell body to form functional synapses with other neurons. Most neurons (up to 70%) are eliminated during normal development by early elimination and programmed cell death (apoptosis), both normal components of neurodevelopment. CNS neural development is regulated by early elimination during the embryonal stage and programmed cell death after birth. Redundant neural progenitor cells and neurons that do not migrate properly or make synapses are physiologically pruned by apoptosis.
Critical periods of plasticity during brain development are modulated by environmental cues and have been implicated in perceptual development in vision and speech. Likewise, the perioperative environment can influence brain development. Anesthetic drugs are powerful modulators of neuronal circuits and have an impact on the constant flux of CNS development and remodeling in both health and disease states. Because neurogenesis is ongoing throughout life, from the fetus to the elderly, these neural progenitor cells are vulnerable to the toxic effects of anesthetic drugs. Exposure to isoflurane produces neuronal cell death in brain regions where neural progenitor cells reside. Therefore, susceptibility to AIDN extends from the fetal period to late adulthood.
Brain growth spurt in most species is likely the time of maximal susceptibility to AIDN. This time corresponds with the time of maximal synaptogenesis. The growth spurt of human brains occurs in the last trimester of gestation until about 3 to 4 years of age, which is the time that children are most vulnerable to the negative effects of general anesthesia (also see Chapter 34 ). There is controversy about this though. Yet, neuroinformatic mapping of the development of corticospinal tracts across species demonstrates that 7-day-old rat pups are neurodevelopmentally closer to 20- to 22-week-old human fetuses. This is also the time of maximal susceptibility to fetal alcohol syndrome, which involves fetal exposure to alcohol, which is both a GABA agonist as well as an NMDA antagonist.
The timing of maximal brain growth during development is species dependent. Rodents are altricial species and much of their neurodevelopment occurs postnatally. This time period occurs from about postnatal day 6 through postnatal day 21. Simian species including humans are usually considered precocial and typically have a longer gestation because offspring are born at a relatively advanced stage of development. Rhesus monkeys are susceptible to anesthetic-induced neuroapoptosis when exposed as fetuses or up to day 6 of life. However, AIDN has not been demonstrated when the exposure occurs on day 35 postnatally. Five-day-old rhesus monkeys given equivalent human concentrations (0.5%-1.5%) of isoflurane for 4 to 6 hours, either with or without nitrous oxide, developed extensive neuroapoptosis found in the temporal, frontal, and hippocampal areas of the brain. However, ketamine given in very large doses for a prolonged duration causes both an increased level of neuroapoptosis, and later, learning deficits in exposed rhesus monkeys.
The time of exposure during development results in contrasting patterns of neural fate. Exposure of anesthetics to pregnant rats results in increased apoptotic cells in the brains of the fetuses. Administration of anesthetics to neonatal rodents leads to increased apoptosis and stunted axonal growth and dendritic arborization. In contrast, anesthetic exposure in juvenile rat models does not increase apoptosis but leads to enhanced dendritic formation and synaptic density. It is of concern that similar altered dendritic morphologic appearance has been observed in psychiatric and neurologic disorders.
Characterization of AIDN
Pathologic Apoptosis
Accelerated apoptosis is the hallmark of AIDN ( Table 12.1 ). Although an essential process in controlling neural development, the apoptotic pathway is also activated by cellular stress. Such stresses include glucocorticoids, heat, radiation, starvation, infection, hypoxia, pain, and anesthetics. Apoptosis is almost always executed by caspase enzymes, which are cysteine dependent aspartate proteases that either initiate the apoptotic process (caspases 2, 8, 9, and 10) or affect the process (caspases 3, and 7). The two main pathways are the extrinsic and intrinsic pathways. The extrinsic pathway is mediated by death receptors on the cell membrane wall whereas the intrinsic pathway is dependent on mitochondrial activation.
Feature | Comment (see text for details) |
---|---|
Pathologic apoptosis | The hallmark of AIDN Can be induced by extrinsic or intrinsic pathways. |
Impeded neurogenesis | Effect of anesthetics on neurogenesis is age-dependent |
Altered dendritic development | Anesthetics affect dendritic morphogenesis in age-dependent manner |
Aberrant glial development | Isoflurane can interfere with release of trophic factors by astrocytes |
The extrinsic pathway involves the Fas ligand to the Fas cell wall receptor, which then becomes the Fas-associated death domain, or FADD. This joins with either procaspase 8 or 10 to become a death-inducing signaling complex (DISC). This activates the effector caspase 3 to induce cell death. The extrinsic pathway can also be induced by the tumor necrosis factor–related apoptosis-inducing ligand (TRAIL), which also causes activation of FADD, DISC formation, and apoptosis.
The intrinsic pathway involves the mitochondria, which under stress release proapoptotic proteins such as cytochrome c , procaspases, Smac/Diablo, endonuclease G, adenylate kinase-2, and apoptosis-inducing factor (AIF). AIF can induce apoptosis without caspase activation, which differs from other proteins. These proapoptotic proteins are released from the space between the inner and outer mitochondrial layers by increased permeability of the outer mitochondrial wall. The Bcl-2 cytosolic proteins are made up of proapoptotic and prosurvival components. The mitochondrial outer wall permeability is induced by the Bax proteins (Bcl-2 proapoptotic) and leads to the release of cytochrome c as well as other proteins that activate caspase 9. Exposure to volatile anesthetics impairs mitochondrial function, which in turn activitates the intrinsic apoptotic pathway. Reactive oxygen species (ROS) scavengers and restoration of mitochondrial integrity mitigate this response.
Impeded Neurogenesis
Anesthetics affect neurogenesis in animals in an age-dependent manner. Isoflurane causes loss of neural stem cells and reduced neurogenesis in neonatal but not adult rats, where it causes a brief increase in neurogenesis. Likewise propofol decreases hippocampal cell proliferation in young rats but not in adults. Exposure to isoflurane impairs growth and delayed maturation of astrocytes in young animals. Inflammation caused by general anesthetics may also cause a decrease in neurogenesis in animals. Based on both in vivo and in vitro evidence, general anesthetics may decrease both the pool of neural stem cells and their capacity for self-renewal, especially in juveniles and adults.
Altered Dendritic Development
Dendritic spines are small protrusions of the neurons that typically receive input from a single synapse of an axon and are essential components of synaptogenesis. Exposure to ketamine and isoflurane decreases synapse and spine density in very young infant rats. However, in slightly older rats (age postnatal days 15, 16, and 20), exposure to propofol, midazolam, isoflurane, sevoflurane, desflurane, and ketamine leads to an increase in dendritic spine formation. The implications of a decrease in dendritic spine formation at a very young age and an increase in slightly older animals are unclear. However, the impact of the vulnerability of specific developmental stages on AIDN is clear. Motor skill learning by subjecting rats to running on a rotarod enhances dendritic development. Exposure to ketamine and xylazine at postnatal day 14 resulted in a reduction of dendritic spine formation. Taken together, the impact of anesthetics on dendritic morphogenesis clearly differs with the age at which exposure occurs.
Aberrant Glial Development
The glial cells within the CNS form the scaffolding, which guides the migration and synaptogenesis of the neurons during development. Astrocytes are impaired during neural development by exposure to isoflurane. This anesthetic interferes with the release of brain-derived neurotrophic factor (BDNF) by astrocytes, which in turn deprive the developing neurons of trophic support for axonal outgrowth. Isoflurane also induces apoptosis of oligodendrocytes in fetuses and neonate rhesus monkeys.
Anesthetic Effects on Spinal Cord
General anesthetic exposure (isoflurane, nitrous oxide) in very young rat pups causes an increase in apoptosis in the spinal cord with a preponderance of injury in the ventral horns. However, no motor functional disabilities were detected in exposed rats that were allowed to mature. Postnatal day 3 rat pups that received an intrathecal injection of ketamine had increased apoptosis and microglial activation on histologic examination of their spinal cords and deranged spinal function at adulthood. Intrathecal morphine produced analgesia, but no histologic or functional changes on the spinal cord. Exposure to a local anesthetic (bupivacaine) in the same population did not cause an increased level of apoptosis.
Neuroinflammation
Activation of neuroinflammatory cascades may influence the development of postoperative cognitive dysfunction (also see Chapter 35 ). Surgical trauma clearly activates neuroinflammation. Therefore, the administration of anesthetic and analgesic drugs during surgery and painful procedures should minimize this response. However, sevoflurane increases markers of neuroinflammation in young but not adult mice. It is unclear if the impact of surgical trauma and anesthetic exposure are additive in inducing neuroinflammation.
Alzheimer-Related Neuropathology
Preclinical reports demonstrate expression of biologic precursors of Alzheimer disease. Experimental surgery on mice increased β-amyloid accumulation in the hippocampus. Furthermore, exposure to isoflurane leads to increased β-amyloid levels in cell culture and rodent brains. Neuroinflammation and Alzheimer disease neuropathology is a potent combination that could diminish neurocognitive function.
Neurocognitive Function
Decrements in neurocognitive function clearly occur after fetal and neonatal exposure to anesthetic drugs in rodents. Standard behavioral measures in rodents include the Morris water navigation test, radial arm maze, startle, prepulse inhibition of the startle reflex, and odor recognition testing. Behavioral tests were also described in rhesus monkeys exposed to ketamine or sevoflurane with operant test battery or human intruder paradigm, respectively. The operant test battery is a measure of motivation and recognition memory, whereas the human intruder paradigm is a test for emotional reactivity. Both reports demonstrated a diminution of performance at an older age after neonatal exposure to these drugs.
Exposure to anesthetic drugs also adversely impacts neurobehavioral assessments of elderly rats. Six- and 20-month-old rats anesthetized with isoflurane and nitrous oxide equally developed persistent deficits in the radial arm maze test. However, propofol did not result in impaired radial arm maze test results in a similar experimental paradigm. These reports clearly demonstrate that exposure to anesthetic drugs can lead to neurobehavioral functional consequence at a later age (also see Chapter 35 ).
Relevant Anesthetic Durations and Concentrations
The duration of exposure may be more relevant than the concentration of exposure, although both are important. Almost all the animal studies involved an anesthetic exposure of at least 4 hours with some trials exposing primates to 24 hours of continuous anesthesia. Exposures of less than 1 hour regardless of the animal studied did not cause increased neuroapoptosis. Exposure to volatile anesthetics of 0.25% to 0.5% minimum alveolar concentration (MAC) for 6 hours increased caspase 3 marker levels, indicating an increase in cell death or apoptosis in rat pups. There is inconsistency about the relative neurotoxic potential of individual volatile anesthetics. Whether desflurane induces more neuroapoptosis than sevoflurane or halothane is not clear. Also, whether anesthetics given in combinations are more neurotoxic than solitary anesthetics is not known. Although nitrous oxide in combination with isoflurane is more neurotoxic than isoflurane alone, it may be because the total MAC exposure is higher when given in combination rather than a synergistic effect.
Anesthetic and Sedative Drugs
GABAergic general anesthetics act on the GABA A receptor. Although GABA is inhibitory in the mature brain, it is an excitatory agent during early stages of brain development. The immature Na/K/2Cl transporter protein NKCC1 produces a chloride influx leading to neuron depolarization. As a consequence GABA remains excitatory until the GABA neurons switch to the normal inhibitory mode when the mature chloride transporter, KCC2, is expressed, which actively transports chloride out of the neural cell. This switch begins around the 15th postnatal week in term human infants but is not complete until about 1 year of age.
The N -methyl- d -aspartate glutamate receptor (NMDAR) is found in neurons and is activated when glutamate, glycine, or d -serine binds to it. It is critical for synaptic plasticity, which is needed for learning and memory. Structurally the NMDAR is a protein composed of four subunits—two GluN1 (formerly called NR1) and two GluN2 (formerlly called NR2). The GluN1 subunit binds the coagonist glycine, and the GluN2 subunit binds glutamate. Ketamine, which is a noncompetitive NMDAR antagonist, has been associated with AIDN in animals and causes an upregulation of the GluN1 subunit.
In general, opioids do not increase neuroapoptosis but under some experimental conditions, repeated morphine administration over 7 days is associated with increased apoptosis in the sensory cortex and amygdala of neonatal rats. However, a single dose of morphine given to postnatal day 7 rat pups did not increase neuroapoptosis. Furthermore, daily administration of morphine for 9 consecutive days did not alter dendritic morphologic appearance. These areas of the brain are not the areas of the brain that are affected by volatile and intravenous anesthetics, which preferentially affect the learning and memory areas (hippocampus) of developing brains.
Alleviation of AIDN
Several molecular mechanisms for anesthetic-induced apoptosis have been elucidated. This finding has led to studies designed to determine whether there are clinically available neuroprotective strategies that can ameliorate the negative effects of general anesthetics on developing young children. Several nonspecific drugs, which have neuroprotective properties (lithium, melatonin, estrogen, erythropoietin, estradiol, and dexmedetomidine), alleviate AIDN. Dexmedetomidine mitigates isoflurane-induced neuroapoptosis and behavioral impairment. However, large doses of dexmedetomidine can induce neuroapoptosis. The neuroprotective effect of dexmedetomidine probably induces cell survival signaling pathways at clinical doses. Finally, an enhanced and stimulating environment mitigates neurobehavioral deficits after neonatal exposure to sevoflurane.
Clinical Evidence for Neurotoxicity
Taken together, three factors appear to induce AIDN in laboratory models: (1) susceptibility during a critical period of development, (2) large dose of the anesthetic, and (3) prolonged duration of exposure. Extrapolation of these laboratory data to the human neonate is problematic. A rat brain develops over a matter of weeks, whereas a human brain develops over years. Six hours of anesthesia in a neonatal rat pup may equate to weeks in a human neonate. With the exception of sedation in intensive care patients, this extreme condition is not common in clinical practice. Therefore, uncovering the effect of an equivalent exposure on the neurologic outcome in a human neonate is difficult. Prolonged and repetitive exposure and exposure at a young age to general anesthesia causes the most neuroapoptosis and later developmental delays in animals. Children who need frequent examinations under anesthesia or radiation treatments for cancer theoretically are at increased risk for the neurotoxic effects of general anesthesia.
The implication that general anesthesia may be harmful to children is limited to retrospective epidemiologic analyses (also see Chapter 34 ). This evidence may be confounded by the effects of surgery and the effects of the underlying comorbid conditions. Although control for obvious confounders has been attempted, the retrospective nature of these investigations makes it impossible to control for all the known and unknown confounders. There have been several epidemiologic studies originating from the Mayo Clinic. The population in Olmsted County, Minnesota, is stable, and researchers there have access to both their medical records as well as the school records of this population. A retrospective cohort study of over 5000 children born from 1976 through 1982 found more reading, written language, and math learning disabilities in the 593 patients who were exposed to anesthesia before the age of 4 years. Risk factors included more than one anesthetic exposure and general anesthesia lasting longer than 2 hours. A similar study was done using a matched cohort of 8530 children from Olmsted County, which found that the 64 children less than 2 years of age who had more than one anesthetic exposure were almost twice as likely to have speech and language disabilities as the children who had a single or no anesthetic exposure. An analysis of these retrospective studies disclosed that halothane (no longer a commonly used inhaled anesthetic) was the chief anesthetic used, most of the cases were performed before pulse oximetry was commonly used, and the records used were handwritten so that there may have been some informational bias.
A database of over 200,000 children was developed using the New York State Medicaid billing codes. Initial studies from this database revealed that children undergoing inguinal hernia repair at less than 1 year of age had almost a threefold increase in diagnoses relating to developmental and behavioral issues. When this group was controlled for gender and birth weight there was still a nearly twofold increase in these issues. However, a follow-up study matching exposed twins with nonanesthetic exposed twin siblings found that there was no association between general anesthesia receipt and later neurologic and developmental problems. A small retrospective cohort paper was published of children who received anesthesia before the age of 4 years. Fifty-three exposed children were matched with the same number of control children. All the children underwent cranial magnetic resonance imaging studies as well as neurocognitive examinations. They found that the previously exposed children scored significantly lower in listening comprehension and performance intelligence quotient (IQ). Exposure did not lead to gross elimination of gray matter in regions previously identified as vulnerable in animals. Decreased performance IQ and language comprehension, however, were associated with lower gray matter density in the occipital cortex and cerebellum. The general conclusion is that there is a more frequent risk to children who have had two or more anesthetic exposures. However, a 2012 prospectively followed cohort study from Australia of 2608 children exposed to a wide variety of general anesthetics and surgical procedures before the age of 3 years found that even a single exposure to general anesthesia was related to decreased performance on receptive and expressive language and cognitive testing performed at age 10 years. Another prospective evaluation compared a smaller group of children exposed to anesthesia before 1 year of age to a similar number of age- and gender-matched children who had not received anesthesia. The study revealed that the anesthetized children had deficits in measures of long-term recognition memory, but no differences in familiarity, IQ, and Child Behavior Checklist scores.
Large database clinical investigations from Canada and Sweden reveal that exposure to surgery and anesthesia at an age older than 2 to 4 years increased the odds ratio of cognitive deficits, though not to the extent of previously published retrospective reports from smaller populations. Scrutiny of these large datasets reveals a lower percentage in academic achievement scores for toddlers undergoing ear, nose, and throat surgery. This finding suggests that early derangements in hearing and speech may have an impact on subsequent cognitive domains as assessed by school performance.
Other studies have cast doubt on the association between exposure to general anesthesia at a young age and later school problems. A study from the Netherlands evaluating the educational achievements of 1143 identical twin pairs found that twin pairs in which any member of the twin pair was exposed to general anesthesia had lesser educational achievements than unexposed twin pairs. However, the educational achievements of discordant twin pairs (one twin exposed, one twin nonexposed) were similar to each other, meaning that the receipt of general anesthesia did not appear to be a relevant factor. Similarly, a large cohort study of 2689 children born in Denmark between 1986 and 1990 who underwent inguinal herniorrhaphy as infants were matched to control subjects who were randomly selected from an age-matched sample representing 5% of the population. This study found no statistically significant differences between the exposed and nonexposed children after adjusting for known confounders.
Two published large prospective cohort studies support the contention that there is no impact of anesthetic exposure on subsequent neurocognitive domains in children. The GAS study is the only prospective randomized controlled trial to date comparing the effects of general anesthesia with regional anesthesia for inguinal hernia surgery in early infancy. This interim analysis found no evidence that 1 hour of sevoflurane anesthesia in infancy increases the risk of adverse neurodevelopmental outcome at 2 years of age compared with awake-regional anesthesia. The primary outcome, which is a 5-year assessment, is under way. The PANDA study prospectively examined the impact of inguinal hernia surgery in infants younger than 36 months of age. An extensive battery of neurocognitive tests was used to compare each anesthetized infant to a sibling who had no anesthesia exposure. When compared to a sibling cohort naïve to surgery and general anesthesia, there was no significant difference in the tested neurocognitive domains. Both negative studies only examined the impact of short exposures to general anesthesia and surgery. These findings are consistent with the lack of AIDN after exposures of short duration in laboratory animals. Furthermore, the neurocognitive assessments in all the clinical reports were performed in childhood and adolescence, not at adulthood. Therefore, the relationship between prolonged exposure to anesthetic drugs and neurocognitive performance in later stages of the life span needs to be addressed in future investigations.
On the other end of the age spectrum, elderly patients are at increased risk of developing postoperative cognitive dysfunction after surgery and anesthesia (also see Chapter 35 ).

Full access? Get Clinical Tree
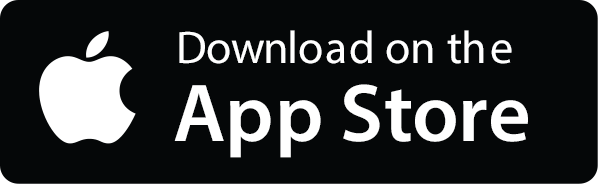
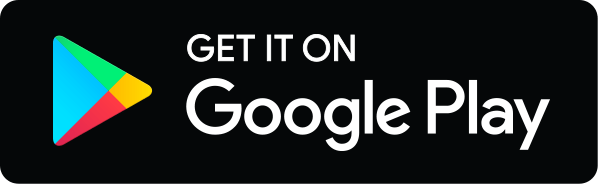