KEY POINTS
1. Increasing utilization of intraoperative transesophageal echocardiography (TEE) has greatly expanded the role of the cardiac anesthesiologist in operations for valve repair and replacement.
2. Because atrial contraction contributes up to 40% of left ventricular (LV) filling in patients with aortic stenosis (AS) and with hypertrophinc obstructive cardiomyopathy, it is essential to maintain sinus rhythm and treat arrhythmias aggressively in both of these conditions.
3. In patients with AS, the early use of α-adrenergic agonists such as phenylephrine is indicated to prevent drops in blood pressure that can lead quickly to sudden death.
4. Patients with severe acute aortic regurgitation are not capable of maintaining sufficient forward stroke volume (FSV) and often develop sudden and severe dyspnea, cardiovascular collapse, and deteriorate rapidly. Patients with chronic aortic regurgitation may be asymptomatic for many years.
5. In patients with mitral stenosis, particular attention should be paid to avoiding any increases in pulmonary artery pressure (PAP) due to inadequate anesthesia or inadvertent acidosis, hypercapnia, or hypoxemia.
6. Bradycardia is harmful in patients with mitral regurgitation, leading to an increase in LV volume, reduction in forward cardiac output, and an increase in regurgitant fraction (RF). The heart rate should be kept in the normal to elevated range.
7. In patients with tricuspid regurgitation (TR), high airway pressures during pulmonary ventilation and agents that can increase pulmonary arterial (PA) pressure should be avoided. If inotropic support is necessary, dobutamine, isoproterenol, or milrinone, which dilate the pulmonary vasculature, should be used.
8. Hypertrophy and pressure within the right ventricle with pulmonic stenosis limit right ventricular (RV) subendocardial blood flow to diastole. Coronary perfusion pressure (CPP) must be maintained to provide an adequate RV subendocardial coronary blood supply.
9. The hemodynamic requirements for AS and mitral regurgitation are contradictory. Because AS will most frequently lead these patients into deadly intraoperative situations, it should be given priority when managing the hemodynamic variables.
I. Introduction
Following the “golden age” of cardiac surgery in the 1980s and 1990s, recent years have seen an overall decline in cardiac surgery volume. Surgery is no longer the preferred option for treating many forms of cardiac disease, especially in the case of coronary artery disease (CAD). The number of coronary artery bypass grafting (CABG) operations has undergone a noticeable decline, in large part due to continuing advances in the field of interventional cardiology. However, the same does not hold true for the surgical treatment of valvular heart disease, as the volume of valve surgery remains steady and now represents 10% to 20% of all cardiac surgical procedures in the United States [1]. In the majority of these cases, surgery remains the best, and often only effective, approach to treatment. Valve surgery should continue to thrive with an aging patient population and continued improvements in surgical techniques, available prosthetic valves, and patient outcomes following these procedures. Approximately 66% of valvular surgery is on the aortic valve, most often because of AS [1].
The prevalence of valvular heart disease remains relatively constant at about 2.5% of the population in industrialized countries because an increase in the frequency of degenerative valvular disease has balanced the decrease in rheumatic disease. Further, the widespread use of echocardiography improves the detection and may increase the apparent incidence of disease. In Nkomo’s large echo-based series in Minnesota, the two most common valvular lesions were mitral regurgitation and AS, and the prevalence of disease increased markedly with age, ranging from less than 2% before age 65, to 8.5% in patients age 65 to 75, to 13.2% in patients older than 75 yrs [2]. Further, almost one-third of patients presenting for surgical management have had a prior medical or surgical intervention [3]. The mortality of valve surgery ranges from 0% to 3% for isolated mitral valve repair to 6% to 11% in patients undergoing multiple valve replacement, depending on the severity of the patient’s cardiac disease as well as their age and general health [4].
The anesthetic management of valvular surgical patients is often quite challenging. These lesions may lead to pathophysiologic changes in the heart with profound hemodynamic consequences, particularly in the setting of general anesthesia and surgical stress. A well-planned anesthetic must compensate for these stresses by manipulating several hemodynamic variables. The most important variables to consider include heart rate and rhythm, preload, afterload, and contractility. In addition, it is critical to consider the time course of the disease, as the clinical presentation and management will vary dramatically in the setting of acute versus chronic valvular disorders.
1
Increasing utilization of intraoperative TEE has greatly expanded the role of the cardiac anesthesiologist in operations for valve repair and replacement. In the setting of valve surgery, the anesthesiologist is frequently consulted by the surgeon to provide diagnostic interpretation of the echo findings to help guide the operative approach. The echo exam is also critical to immediately assess the adequacy of a valve repair or replacement and, if necessary, alert the surgeon to any complications that may require further attention and correction. There are few other settings in which an anesthesiologist’s diagnostic skills play such an integral role in surgical decision making as in the cardiac operating room during valve surgery.
This chapter will review the physiologic implications of the most common types of valvular heart disease and the practical approach to the anesthetic management of these patients. All four cardiac valves will be discussed, focusing on the stenotic and regurgitant lesions of each. A section addressing prosthetic valves will conclude the chapter with a review of each of the different types of prostheses most frequently used for valve replacement.
II. Stenotic versus regurgitant lesions
A. Valvular stenosis. Stenotic lesions lead to pathology associated with pressure overload. Narrowing of the orifice of a cardiac valve will ultimately lead to obstruction of blood flow through the valve. This obstruction translates into an increase in blood flow velocity as it approaches the stenotic valve orifice. The pattern of blood flow is distinctly different in the regions proximal and distal to a stenotic valve. The high-velocity flow proximal to the stenosis is laminar and organized, whereas distal to the stenosis, it becomes turbulent and disorganized. In addition, the increased blood flow velocities observed in valvular stenosis translate into an increase in the pressure gradient across the valve. The simplified Bernoulli equation helps explain this relationship. In this equation, the pressure gradient through the stenotic valve can be estimated by multiplying the velocity squared times four:
Pressure gradient (Δ) = 4 * Blood Flow Velocity (v) squared
(ΔP = 4v2)
The simplified Bernoulli equation allows blood flow velocities measured by Doppler echocardiography to be converted into pressure gradients that can be used to quantify the severity of valvular stenosis [5]. It is also important to understand that valvular obstruction can be of two primary types: Fixed versus dynamic. In fixed obstruction (i.e., true valvulvar AS, subaortic membrane), the degree of obstruction to blood flow remains constant throughout the cardiac cycle and is not affected by the loading conditions of the heart. With dynamic obstruction (i.e., HOCM with dynamic subaortic stenosis), obstruction is only present for part of the cardiac cycle, primarily occurring in mid- to late systole. The degree of obstruction is highly dependent on loading conditions, changing in severity as loading conditions change.
B. Valvular regurgitation. Regurgitant lesions lead to pathology associated with volume overload, resulting in chamber dilatation and eccentric hypertrophy. Clinically, although this chamber remodeling will initially allow the left ventricle to compensate for the increased volume load, it will lead to an eventual decline in LV systolic function that can ultimately lead to irreversible LV failure. Effective perioperative management of valvular regurgitation is facilitated by understanding how preload, afterload, and heart rate each affect the contributions of the FSV (flow reaching the peripheral circulation) and regurgitant stroke volume (retrograde flow back across the valve) to the overall total stroke volume (TSV) of the ventricle [5]. Hemodynamic management of these patients should aim to optimize the FSV while minimizing the amount of regurgitant stroke volume.
III. Structural and functional response to valvular heart disease.
The anesthetic management of patients undergoing valvular heart surgery requires a thorough understanding of the hemodynamic changes associated with valvular heart disease, as well as the cardiac remodeling imposed by abnormal valves.
A. Cardiac remodeling includes changes in the size, shape, and function of the heart in response to an acute or chronic cardiac injury. In valvular heart disease, cardiac injury is usually caused by alterations in ventricular loading conditions. Depending on the nature of the valvular pathology, the ventricle will be subject to either pressure or volume overload, or both. This leads to cardiac remodeling in the form of chamber dilation and ventricular hypertrophy. In addition to mechanical stress, cardiac remodeling results from the activation of neurohumoral factors, enzymes such as angiotensin II, ion channels, and oxidative stress [6]. Intended initially as an adaptive response to maintain cardiac performance, remodeling eventually leads to decompensation and deterioration in ventricular function. Ventricular hypertrophy is defined as increased LV mass. Ventricular hypertrophy can be either concentric or eccentric. Pressure overload usually results in concentric ventricular hypertrophy, which means that ventricular mass is increased by myocardial thickening, whereas ventricular volume is not increased. Its adaptive purpose is to reduce the increased wall stress that results from the chronic pressure overload. Recall the law of LaPlace to understand how this compensatory hypertrophy results in reduced wall stress, where:
LV wall stress = (LV pressure * LV radius)/2 * LV wall thickness
The increased afterload results in an elevated LV pressure, which translates into an increase in LV wall stress. The resulting increase in wall thickness, seen in the denominator of the above equation, will have the beneficial effect of reducing LV wall stress and avoiding a significant decline in LV systolic function. The cost of LV hypertrophy is a reduction in LV compliance, which leads to diastolic dysfunction with an increase in LV end-diastolic pressure (LVEDP) and subendocardial ischemia.
Volume overload, on the other hand, leads to eccentric hypertrophy, which means that ventricular mass is increased by an increase in ventricular volume, whereas myocardial thickness remains unchanged [3].
B. Ventricular function. To anticipate the effect of valvular lesions on ventricular function, it is helpful to separate ventricular function into its two distinct components [3].
1. Systolic function represents the ventricle’s ability to contract and eject blood. Normal systolic function means that ventricular contractility is normal.
a. Contractility can be defined as the intrinsic ability of the myocardium to contract and generate force. Contractility itself is independent of preload and afterload. Normal contractility means that a ventricle of normal size and normal preload can generate sufficient stroke volume at rest and during exercise.
b. Preload can be defined as the load placed on myocardium before the contraction. This load results from a combination of diastolic volume and filling pressure and can be expressed as end-diastolic stress.
c. Afterload is the load placed on the myocardium during contraction. This load results from the combination of systolic volume and generated pressure and can be expressed as end-systolic stress.
2. Diastolic function represents the ventricle’s ability to accept inflowing blood. Diastolic function consists of a combination of relaxation and compliance. In general, normal diastolic function means that the ventricle accepts normal diastolic volume at normal filling pressure. When diastolic dysfunction occurs, maintaining normal ventricular diastolic volume requires elevated ventricular filling pressure. Both systolic and diastolic functions require energy and can be compromised by ventricular ischemia.
IV. Pressure–volume loops may be utilized to illustrate LV function and performance. These loops are constructed by plotting ventricular pressure (y-axis) versus ventricular volume (x-axis) over the course of a complete cardiac cycle (Fig. 12.1). The presence of valvular heart disease alters the normal pressure–volume loop tracing, representing changes in ventricular physiology and loading conditions imposed by valvular pathology. The ventricle adapts differently to each valvular lesion and characteristic patterns of the pressure–volume loop help illustrate these changes.
Figure 12.1 Normal pressure–volume loop. The first segment of the ventricular pressure–volume loop (Phase 1) represents diastolic filling of the left ventricle. The next two segments represent the two stages of ventricular systole: Isovolemic contraction (Phase 2) and ventricular ejection (Phase 3). The final segment of the loop corresponds to isovolemic relaxation of the left ventricle, which precedes ventricular filling and the start of the next cardiac cycle. The isovolemic relaxation and ventricular filling phases constitute the two phases of diastole. Both end-systolic volume at the time of aortic valve closure (AC), and end-diastolic volume at the time of mitral valve closure (MC), are represented as distinct points on the loop. MO, mitral valve opening; AO, aortic valve opening. [Modified from Jackson JM, Thomas SJ, Lowenstein E. Anesthetic management of patients with valvular heart disease. Semin Anesth. 1982;1:240.]
V. Aortic stenosis (AS)
A. Natural history
1. Etiology. The normal adult aortic valve has three cusps, with an aortic valve area of 2.6 to 3.5 cm2, representing a normal aortic valve index of 2 cm2/m2. AS may result from congenital or acquired valvular heart disease. Congenital AS is classified as valvular, subvalvular, or supravalvular based on the anatomic location of the stenotic lesion. Subvalvular and supravalvular AS are usually caused by a membrane or muscular band. Congenital valvular AS may occur with a unicuspid, bicuspid, or a tricuspid aortic valve with partial commissural fusion.
A congenitally bicuspid aortic valve occurs in approximately 1% to 2% of the general population, making it the most common congenital valvular malformation. Calcification of a congentially bicuspid valve results in the early onset of AS, and represents the most common cause of AS among patients younger than 70 yrs of age [7]. Currently, bicuspid aortic valve disease accounts for approximately 50% of all valve replacements for AS in the United States and Europe [8]. Commonly associated findings in patients with bicuspid aortic valves include abnormalities of the aorta, including aortic coarctation, aortic root dilatation, and an increased risk of aortic dissection.
Of the acquired aortic stenoses, senile degeneration is the most common cause in the developed world, with 30% patients older than 85 yrs demonstrating significant degenerative aortic valve changes on autopsy. The calcification seen with senile degeneration of the aortic valve also appears to have an inflammatory component as well, similar to that observed in CAD [3]. While rheumatic AS is now rarely seen in the developed world, it remains the most common cause of AS worldwide. Additionally, rheumatic AS is usually associated with some degree of aortic regurgitation and frequently affects the mitral valve as well. Less frequent causes of AS include atherosclerosis, end-stage renal disease, and rheumatoid arthritis.
A characteristic finding of senile valvular degeneration is progression of the calcification from the base of the valve toward the edge, as opposed to rheumatic degeneration, in which calcification spreads from the edge toward the base.
2. Symptoms. Unicuspid AS often presents in infancy. Patients with rheumatic AS may be asymptomatic for 40 yrs or more. Congenital bicuspid aortic valves in the majority of cases must undergo calcific degeneration to become stenotic. The time of onset and speed of progression of calcific degeneration varies from patient to patient. This is why patients with congenitally bicuspid aortic valves may develop symptomatic AS anytime between the ages of 15 and 65, and even later in life. Degenerative stenosis of a tricuspid aortic valve usually develops in the seventh or eighth decade of life. Asymptomatic patients with AS have an excellent prognosis [3]. Patients with even severe AS may stay asymptomatic for many years and carry a small risk of sudden death, which does not exceed the risk of operation. However, the onset of any one of the following triad of symptoms is an ominous sign and indicates a life expectancy of less than 5 yrs:
a. Angina pectoris. Angina is the initial symptom in approximately two-thirds of patients with severe AS. Angina and dyspnea secondary to AS alone initially occur with exertion [1]. Life expectancy when angina develops is about 5 yrs.
b. Syncope. Syncope is the first symptom in 15% to 30% of patients. Once syncope appears, the average life expectancy is 3 to 4 yrs.
c. Congestive heart failure. Once signs of LV failure occur, the average life expectancy is only 1 to 2 yrs.
B. Pathophysiology
1. Heart remodeling. As stenosis progresses, the maintenance of normal stroke volume is associated with an increasing systolic pressure gradient between the LV and the aorta. The LV systolic pressure increases to as much as 300 mm Hg, whereas the aortic systolic pressure and stroke volume remain relatively normal. This pressure gradient results in a compensatory concentric LV hypertrophy. As stenosis progresses, eccentric LV hypertrophy may develop. This is usually associated with low LV ejection fraction, indicating a compromise of LV contractility.
2. Hemodynamic changes
a. Arterial pressure. In severe AS, the arterial pulse pressure usually is reduced to less than 50 mm Hg. The systolic pressure rise is delayed with a late peak and a prominent anacrotic notch. As stenosis increases in severity, the anacrotic notch occurs lower in the ascending arterial pressure trace. The dicrotic notch is relatively small or absent.
b. PA wedge pressure. Because of the elevated LVEDP, which stretches the mitral valve annulus, a prominent V wave can be observed, but with progression of the disease and the development of left atrial hypertrophy, a prominent A wave becomes the dominant feature.
3. Pressure–Volume Loop in AS. (Fig. 12.2)
Figure 12.2 Pressure–volume loop in AS. In comparison to the normal loop, note the elevated peak systolic pressure necessary to generate a normal stroke volume in the face of the elevated pressure gradient through the aortic valve. Also, end-diastolic pressure is elevated with a steeper diastolic slope, reflecting diastolic dysfunction with altered LV compliance. Phase 1, Diastolic filling; Phase 2, Isovolumetric contraction; Phase 3, Ventricular ejection; Phase 4, Isovolumetric relaxation; MO, Mitral valve opening; MC, Mitral valve closure; AO, Aortic valve opening; AC, Aortic valve closure. [Modified from Jackson JM, Thomas SJ, Lowenstein E. Anesthetic management of patients with valvular heart disease. Semin Anesth. 1982;1:241.]
C. Assessment of severity (echocardiographic criteria; see Table 12.1)
Table 12.1
1. Transthoracic echocardiography. Echocardiography is now the standard modality for quantifying the severity of AS. With the exception of rare cases where echocardiography is non-diagnostic or discrepant with clinical data, cardiac catheterization is no longer recommended for this purpose. The most commonly utilized methods for quantifying AS severity with echocardiography include measurement of the peak blood flow velocity of the AS jet, mean gradient across the aortic valve, and determination of aortic valve area. In AS, the valve area can be measured by the direct planimetry and continuity equation methods. The pressure gradient can be measured using a simplified Bernoulli equation. (Fig. 12.3) Cardiac remodeling is assessed by measurement of left atrial size, LV end-systolic and end-diastolic dimensions, and LV myocardial thickness.
Figure 12.3 Severe AS, showing determination of valve gradient using the Bernoulli equation. This image displays a deep transgastric long-axis TEE view with a continuous wave Doppler beam aligned through the left ventricular outflow tract (LVOT) and aortic valve (AV). Tracing 2 on the spectral display (outer envelope) demonstrates blood flow through the severely stenotic aortic valve, with a maximum velocity (AV Vmax) of 4.95 m/s and peak AV pressure gradient (AV max PG) of 97.9 mmHg. Tracing 1 (inner envelope) demonstrates blood flow through the LVOT, with a maximum velocity (Vmax) of 1.19 m/s and peak LVOT pressure gradient (Pmax) of 5.66 mmHg. Additional parameters: AV Vmean, Mean velocity of blood flow across the aortic valve; AV meanPG, Mean pressure gradient across the aortic valve; AV VTI, Aortic valve velocity-time integral; V mean, Mean velocity of blood flow in LVOT; Pmean, Mean pressure gradient in LVOT; VTI, LVOT velocity-time integral in LVOT; HR, Heart Rate. [ECHO Image from Perrino AC, Reeves ST, eds. A Practical Approach to Transesophageal Echocardiography. 2nd ed. Philadelphia, PA: Lippincott Williams & Wilkins; 2008:246, Figure 12.2.]
2. Transesophageal echocardiography (TEE). TEE is useful in patients with a poor transthoracic window or in patients with complex cardiac pathology (e.g., a combination of subaortic and valvular stenosis). It is also useful when precise planimetry of the aortic valve area is necessary or when infective endocarditis is suspected.
3. Dobutamine stress echocardiography is useful in patients with apparently severe AS on the echocardiogram that is combined with severe LV dysfunction and low cardiac output. Low cardiac output will not allow the generation of elevated blood flow velocities and pressure gradients commonly observed in AS. This combination of findings may be explained either by primary severe valvular AS causing LV dysfunction or by severe LV dysfunction independent of some degree of AS. In this case, the aortic valve does not open to its full extent due to low stroke volume and low generated pressure, which imitates severe AS. If with dobutamine infusion the transvalvular pressure gradient increases and the aortic valve area on the echocardiogram does not change, then AS is probably fixed and has caused the LV dysfunction. In this case, the patient will most likely benefit from surgical intervention [9]. If, however, the apparent aortic valve area increases with dobutamine, then ventricular dysfunction is likely the prime factor and valve replacement would have little benefit.
4. If cardiac catheterization data is utilized, the mean pressure gradient may be measured from a direct transaortic measurement and the aortic valve area may be calculated using the Gorlin formula.
D. Timing and type of intervention
1. Due to the high risk of sudden death and limited life expectancy, symptomatic patients should undergo surgery. Asymptomatic patients with severe AS may be monitored closely until symptoms develop. However, the risk of waiting should be carefully weighted against the risk of surgery. For example, prior to elective noncardiac surgery under general or neuraxial anesthesia, asymptomatic patients with severe AS should be considered for aortic valve surgery.
2. Patients with moderate AS should have aortic valve surgery if they happen to require another cardiac operation, such as CABG, because the rate of progression of AS is approximately 0.1 cm2/yr and the risk of having to redo cardiac surgery is substantially higher than the risk of the primary operation. Similarly, if a patient undergoing aortic valve surgery has significant CAD, CABG should be performed simultaneously. In patients over age 80 yrs, the risk of aortic valve replacement (AVR) alone is approximately the same as the risk of combined AVR and CABG [10].
3. A commissural incision or balloon aortic valvuloplasty is often the first procedure performed in young patients with severe noncalcific aortc valve stenosis, even if they are asymptomatic [1]. This operation frequently results in some residual AS and aortic regurgitation. Eventually, most patients require a subsequent prosthetic valve replacement. In older adult patients with calcific AS, valve replacement is the primary operation. In young adults, a viable alternative to AVR is the Ross (switch) procedure. In the Ross procedure, the diseased aortic valve is replaced with a patient’s normal pulmonary valve and the pulmonary valve is replaced with a pulmonary homograft. This more complex procedure avoids the need for systemic anticoagulation and extends the time until reoperation is required by several decades.
4. Surgical intervention should not be denied to patients, almost no matter how severe the symptoms, because irreversible LV failure occurs only very late in the disease process.
5. Balloon aortic valvuloplasty in adults with advanced disease often results in significant aortic regurgitation and early restenosis, and is reserved for patients with severe comorbidity. Percutaneous AVR is a treatment modality with potential applications for high-risk patients deemed not suitable candidates for surgery. Transapical AVR, involving a small thoracotomy incision and insertion of the valve via the LV apex, has shown similar promise in this same high-risk patient population. Both techniques require brief cessation of the patient’s cardiac output via rapid pacing during positioning of the device. Hemodynamic instability is common and necessitates prompt recognition and treatment. These techniques have demonstrated improved rates of procedural success and clinical outcomes, with an overall 94% success rate and an 11.3% 30-day mortality [11].
E. Goals of perioperative management
1. Hemodynamic profile (Table 12.2)
Table 12.2
a. LV preload. Due to the decreased LV compliance as well as the increased LVEDP and LV end-diastolic volume (LVEDV), preload augmentation is necessary to maintain a normal stroke volume.
b. Heart rate. Extremes of heart rate are not tolerated well. A high heart rate can lead to decreased coronary perfusion. A low heart rate can limit cardiac output in these patients with a fixed stroke volume. If a choice must be made, however, low heart rates (50 to 70 beats/min) are preferred to rapid heart rates (greater than 90 beats/min) to allow time for systolic ejection across a stenotic aortic valve. Because atrial contraction contributes up to 40% of LV filling, due to decreased LV compliance and impaired early filling during diastole, it is essential to maintain a sinus rhythm. Supraventricular dysrhythmias should be treated aggressively, if necessary, with synchronized DC shock, because both tachycardia and the loss of effective atrial contraction can lead to rapid reduction of cardiac output.
2
c. Contractility. Stroke volume is maintained through preservation of a heightened contractile state. The β-blockade is not well tolerated and can lead to an increase in LVEDV and a decrease in cardiac output, significant enough to induce clinical deterioration.
d. Systemic vascular resistance. Most of the afterload to LV ejection is caused by the stenotic aortic valve itself and thus is fixed. Systemic blood pressure reduction does little to decrease LV afterload. In addition, patients with hemodynamically significant AS cannot increase cardiac output in response to a drop in systemic vascular resistance. Thus, arterial hypotension may rapidly develop in response to the majority of anesthetics. Finally, when hypotension develops, the hypertrophied myocardium of the patient with AS is at great risk for subendocardial ischemia because coronary perfusion depends on maintenance of an adequate systemic diastolic perfusion pressure. Therefore, the early use of a-adrenergic agonists such as phenylephrine is indicated to prevent drops in blood pressure that can lead quickly to sudden death.
3
e. Pulmonary vascular resistance (PVR). Except for end-stage AS, PAPs remain relatively normal. Special intervention for stabilizing PVR is not necessary.
2. Anesthetic techniques
a. Light premedication is necessary to provide a calm patient without tachycardia. An experienced cardiac surgeon should be present, and perfusionists should be prepared, before induction of anesthesia should rapid cardiovascular deterioration necessitate emergency use of cardiopulmonary bypass.
b. Placement of external defibrillator pads should be considered to allow for rapid defibrillation if cardiovascular collapse occurs on induction or prior to sternotomy.
c. Preinduction arterial line placement is standard practice at most institutions and is generally well tolerated with light premedication and local anesthetic infiltration. Invasive blood pressure monitoring facilitates early recognition and intervention if any hemodynamic instability occurs during induction.
d. During induction of anesthesia, in order to maintain hemodynamic stability, medications should be carefully titrated to a fine line between a reasonable depth of anesthesia and hemodynamic stability.
e. During the maintenance stage of anesthesia, anesthetic agents causing myocardial depression, blood pressure reduction, tachycardia, or dysrhythmias can lead to rapid deterioration. A narcotic-based anesthetic is usually chosen for this reason. Low concentrations of volatile anesthetics are usually safe.
f. If the patient develops signs or symptoms of ischemia, nitroglycerin should be used with caution because its effect on preload or arterial pressure may actually make things worse.
g. Thermodilution cardiac output. Pulmonary artery catheters are helpful in evaluating the cardiac output of patients prior to repair of the aortic valve. The pulmonary capillary wedge pressure (PCWP), however, may overestimate preload of a noncompliant LV. Mixed venous oxygen saturation monitoring via an oximetric PA catheter may be used to provide a continuous index of cardiac output. However, because the postbypass management is not likely to be marked by myocardial failure or low output states, this technique may be best reserved for other patients who may be at higher risk of postbypass hemodynamic complications.
There is also a small risk of life-threatening arrhythmias leading to drug-resistant hypotension during passage of a pulmonary artery catheter through the right atrium and ventricle. In the absence of pre-existing left bundle branch block (LBBB) or tachyarrhythmias, a pulmonary artery catheter may be placed under continuous rhythm monitoring, perhaps after placement of transcutaneous pacing electrodes. In the presence of pre-existing abnormal rhythms or conduction disturbances, however, the most conservative approach dictates leaving the catheter tip in a central venous position until the chest is open, when internal defibrillator pads can be easily applied and cardiopulmonary bypass can be initiated within a few minutes if necessary.
h. Omniplane TEE is useful for intraoperative monitoring of LV function, preload, and afterload. TEE can predict prosthetic aortic valve size based on the LV outflow tract width. It is also very helpful in the detection of air and facilitating deairing prior to weaning from cardiopulmonary bypass. TEE is the method of choice for the postbypass assessment of a prosthetic valve for paravalvular regurgitation and prosthetic valve stenosis. It is important to remember that Doppler-derived blood flow velocities and pressure gradient must be interpreted in light of the altered loading conditions seen in the dynamic operating room setting.
i. In the presence of myocardial hypertrophy, adequate myocardial preservation with cardioplegic solution during bypass is a challenging task. A combination of antegrade cardioplegia administered via coronary ostia and retrograde cardioplegia via the coronary sinus has an important role in preserving myocardial integrity.
j. In the absence of preoperative ventricular dysfunction and associated coronary disease, inotropic support often is not required after cardiopulmonary bypass because valve replacement decreases ventricular afterload.
F. Postoperative care. After a sharp drop in the aortic valve gradient, PCWP and LVEDP immediately decrease and stroke volume rises. Myocardial function improves rapidly, although the hypertrophied ventricle may still require an elevated preload to function normally. Over a period of several months, LV hypertrophy regresses. It must be remembered that a prosthetic aortic valve may cause a mean pressure gradient of 7 to 19 mm Hg.
VI. Hypertrophic cardiomyopathy
A. Natural history
1. Etiology and classification. Hypertrophic cardiomyopathy (HCM) is a relatively uncommon genetic disorder affecting approximately 0.2% of the general population. The inheritance pattern is autosomal dominant with variable penetrance, making it a heterogeneous condition with a highly variable presentation. Historically, several names have been given to this disorder, such as idiopathic hypertrophic subaortic stenosis (IHSS) and asymmetric septal hypertrophy. Since ventricular hypertrophy may occur in multiple patterns, not just confined to the septum, the term HCM is now used to describe this disorder. Additionally, despite the classic association of HCM with obstruction to systolic outflow through the left ventricular outflow tract (LVOT), only 25% of patients with HCM exhibit this subvalvular obstruction. The term HOCM is used to refer to this subset of HCM patients.
4
2. Symptoms. Patients with HCM may present with a wide range of symptoms, with many having no symptoms at all. The most common presenting symptom is dyspnea on exertion with poor exercise tolerance. Patients may also experience syncope, presyncope, chest pains, fatigue, and palpitations. Though LVOT obstruction may cause symptoms, there is no clear relationship between the degree of LVOT obstruction and the occurrence or severity of the symptoms. Other equally important causes of symptoms include diastolic dysfunction, dysrhythmias, mitral regurgitation, and an imbalance of myocardial oxygen supply and demand. Unfortunately, the initial presenting symptom in many patients is sudden cardiac death, usually due to ventricular fibrillation. Though all patients with HCM are at risk for sudden death, the highest-risk groups include those with a family history of HCM and young patients undergoing significant physical exertion. This has led to increased and improved measures to screen for HCM in young athletes and patients with a family history of the disorder.
B. Pathophysiology. By definitition, HCM involves an abnormal thickening of the myocardium without an identifiable cause of hypertrophy. There is an absence of chamber dilation and, in most cases, normal to hyperdynamic LV systolic function. In addition, there are important histological derangements, including abnormal cellular architecture and disarray of the cardiac myocytes, interstitial fibrosis, increased connective tissue, and patchy myocardial scarring. These cellular abnormalities contribute significantly to the common, and potentially catastrophic, dysrhythmias seen in these patients. HCM patients are prone to both atrial and ventricular dysrhythmias, with ventricular fibrillation as the most common cause of sudden death.
In the subset of patients with HOCM, hypertrophy occurs disproportionately in the ventricular septum. As the septum enlarges, it extends into and narrows the LVOT, whose borders are formed by the ventricular septum and the anterior leaflet of the mitral valve. During systole, there is further narrowing of the LVOT due to inward septal movement with ventricular contraction, leading to increased blood flow velocities and pressure gradients through the narrowed outflow tract. Rapid blood flow creates hydraulic forces (Venturi effect) capable of pulling the anterior mitral leaflet into the LVOT, causing further narrowing and obstruction. This abnormal systolic anterior motion (SAM) of the mitral valve leads to dynamic obstruction, in which the degree of obstruction varies based upon cardiac loading conditions and contractility. The obstruction occurs proximal to the aortic valve (subaortic) and occurs only in mid- to late systole. The degree of obstruction is directly proportional to LV contractility and inversely proportional to LV preload and afterload.
While dynamic LVOT obstruction is seen in only a subset of HCM patients, most of them exhibit diastolic dysfunction secondary to ventricular hypertrophy, as well as hypertrophy and disarray of the myocytes. Early diastolic filling is impaired secondary to this poor diastolic compliance, making atrial contraction, and thus maintenance of sinus rhythm, critical for adequate diastolic filling. Mitral regurgitation can be significant, as SAM does not allow for normal coaptation of the mitral valve, creating a regurgitant orifice as the anterior mitral valve leaflet is pulled into the LVOT. Mismatch of oxygen supply and demand is a frequent occurrence in HCM that predisposes to ischemia. The hypertrophied myocardium represents a large muscle mass and there is increased oxygen demand associated with elevated ventricular pressures and wall tension [3].
C. Preoperative evaluation and assessment of severity. Echocardiography allows for assessment of the location and severity of hypertrophy and helps determine the necessity and feasibility of potential surgical intervention. It demonstrates the degree of LVOT narrowing, as well as the presence or absence of SAM. Doppler measurements in the LVOT help determine the presence and severity of subaortic obstruction, with outflow gradients of >30 mm Hg representing significant obstruction. In addition to the mitral coaptation defect associated with SAM, the mitral apparatus itself may be abnormal in HCM patients and should be thoroughly examined. LV systolic function is typically normal or hyperdynamic and diastolic function is almost always abnormal.
D. Timing and type of intervention. The mainstay of medical therapy for HCM involves treatment with β-blockers, which help reduce LVOT obstruction due to their negative inotropic effects and reduction in heart rate. Calcium channel blockers are also frequently utilized for their favorable effect on diastolic compliance. The most critical intervention in patients identified as high risk for malignant dysrthythmias is placement of an automated implantable cardioverter-defibrillator. Other non-surgical approaches to decrease outflow obstruction include dual-chamber pacing and ethanol ablation of the ventricular septum. Surgical treatment involves removal of septal muscle tissue to widen the LVOT via septal myomectomy and may occasionally involve modification of the mitral valve apparatus or mitral valve repair/replacement.
E. Goals of perioperative management
1. Hemodynamic profile (Table 12.3)
Table 12.3
a. LV preload. Any condition that leads to a decrease in LV cavity size can potentially exacerbate dynamic LVOT obstruction, as this places the septum and anterior mitral leaflet in closer proximity, narrowing the outflow tract and increasing the potential for SAM and obstruction. In this regard, preload augmentation is essential to help maintain ventricular volume. Additionally, like in AS, diastolic dysfunction will lead to decreased LV compliance with increased LVEDP, which will necessitate adequate preload to maintain a normal stroke volume. Treatment with nitroglycerin, or other vasodilators, should be avoided as it may dangerously reduce cardiac output.
b. Heart rate. It is essential to avoid tachycardia in patients with HCM because it leads to a reduction in ventricular volume, exacerbation of dynamic LVOT obstruction, and increased oxygen demand. Decreased heart rates are beneficial as this prolongs diastole and allows more time for ventricular filling. Maintenance of sinus rhythm is essential, as the atrial contraction component of ventricular filling is critical due to reduced early diastolic filling because of reduced LV compliance.
c. Contractility. Decreases in myocardial contractility help reduce outflow obstruction. The β-blockade, volatile anesthetics, and avoidance of sympathetic stimulation are all beneficial. The use of intraoperative inotropic agents can increase contractility, worsen LVOT, lead to severe hemodynamic instability, and must be avoided.
d. Systemic vascular resistance. Decreases in afterload must be promptly and aggressively treated with vasopressors such as phenylephrine or vasopressin. Hypotension can be especially detrimental in this population because diastolic dysfunction leads to increased LVEDP, requiring an increased blood pressure to provide adequate CPP:
CPP = Diastolic blood pressure (aorta) − LVEDP
e. PVR. PAPs remain relatively normal in this patient population. Special intervention for stabilizing PVR is not necessary.
2. Anesthetic technique
a. Premedication. Many of these patients are on maintenance therapy with β-blockers or calcium channel blockers, which should be given on the day of surgery and continued throughout the perioperative period.
b. Induction and maintenance of anesthesia. During induction and laryngoscopy, careful attention is required to avoid decreases in afterload, as well as sympathetic stimulation leading to increases in heart rate and contractility. Adequate preload must be maintained and all blood or fluid losses must be aggressively replaced. The direct myocardial depression of volatile anesthetics is advantageous.
c. Patients with HCM are at risk for atrial and ventricular tachyarrythmias during surgery. Preparation must be in place for immediate cardioversion or defibrillation.
d. Intraoperative TEE, like preoperative echocardiography, allows visualization of the location and extent of hypertrophy in the septum, the degree SAM, the degree of obstruction, and quantification of the degree of mitral regurgitation. Since CVP and PCWP measurements will overestimate true volume status, TEE is the most reliable means of accurately assessing volume. The ability to monitor LV systolic function and wall motion is useful, as the oxygen supply–demand relationship is tenuous in these patients, making them prone to ischemia. The adequacy of surgical repair and any post-repair complications can be immediately assessed.
e. Postoperative care. Potential complications in the immediate postoperative period following septal myomectomy include residual LVOT obstruction, residual SAM, residual mitral regurgitation, complete heart block, and the creation of a ventricular septal defect.
VII. Aortic regurgitation
A. Natural history
1. Etiology. Aortic insufficiency can be caused by aortic valve disease, aortic root dilation, or a combination of both [1]. Examples of causes of chronic aortic valve insufficiency include rheumatic fever, infective endocarditis, congenital bicuspid valve, and rheumatoid arthritis. Aortic root dilation can be caused by degenerative aortic dilation, syphilitic aortitis, Marfan’s syndrome, and aortic dissection. Acute aortic insufficiency is usually caused by aortic dissection, trauma, or aortic valve endocarditis.
2. Symptoms. Patients with severe acute aortic regurgitation are not capable of maintaining sufficient FSV and often develop sudden and severe dyspnea, cardiovascular collapse, and deteriorate rapidly. Patients with chronic aortic regurgitation may be asymptomatic for many years. Symptoms such as shortness of breath, palpitations, fatigue, and angina usually develop after significant dilatation and dysfunction of the LV myocardium. The 10-yr mortality for asymptomatic aortic regurgitation varies between 5% and 15%. However, once symptoms develop, patients progressively deteriorate and have an expected survival of only 5 to 10 yrs.
B. Pathophysiology
1. Pathophysiology and natural progression
a. Acute aortic regurgitation. The sudden occurrence of acute aortic regurgitation places a major volume load on the LV. The immediate compensatory mechanism for the maintenance of adequate forward flow is increased sympathetic tone, producing tachycardia and an increased contractile state. Fluid retention increases preload. However, the combination of increased LVEDV and increased stroke volume and heart rate may not be sufficient to maintain a normal cardiac output. Rapid deterioration of LV function can occur, necessitating emergency surgical intervention.
b. Chronic aortic regurgitation. The onset of aortic regurgitation leads to LV systolic and diastolic volume overload. The increased volume load causes an increase in the size of the ventricular cavity, or eccentric ventricular hypertrophy. Because the LVEDV increases slowly, the LVEDP remains relatively normal. Forward flow is aided by the presence of chronic peripheral vasodilation, which occurs along with a large stroke volume in patients with mild aortic regurgitation. As the LV dilation and hypertrophy progresses, coronary perfusion finally decreases leading to irreversible LV myocardial tissue damage and dysfunction. The onset of LV dysfunction is followed by an increase in PAP with symptoms of dyspnea and congestive heart failure. As a compensatory mechanism for the poor cardiac output and poor coronary perfusion, sympathetic constriction of the periphery occurs to maintain blood pressure, which in turn leads to further decreases in cardiac output.
2. Pressure wave disturbances
a. Arterial pressure. Incompetence of the aortic valve leads to regurgitant blood flow from the aorta back into the LV during diastole. This causes a pronounced decline in aortic diastolic blood pressure, translating into a wide pulse pressure. Patients with aortic regurgitation, therefore, show a wide pulse pressure with a rapid rate of rise, a high systolic peak, and a low diastolic pressure. The pulse pressure may be as great as 80 to 100 mm Hg. The rapid upstroke is due to the large stroke volume, and the rapid downstroke is due to the rapid flow of blood from the aorta back into the ventricle and into the dilated peripheral vessels. The occurrence of a double-peaked or bisferiens pulse trace is not unusual due to the occurrence of a “tidal” or backwave.
b. Pulmonary capillary wedge trace. Stretching of the mitral valve annulus may lead to functional mitral regurgitation, a prominent V wave, and a rapid Y descent. In patients with acute aortic regurgitation associated with poor ventricular compliance, LV pressure may increase fast enough to close the mitral valve before end diastole. In this situation, the continued regurgitation of blood raises the LVEDP above left atrial pressure, and the PCWP can significantly underestimate the true LVEDP.
3. Pressure–volume loop in aortic regurgitation (Fig. 12.4)
Figure 12.4 Pressure–volume loop in acute and chronic aortic regurgitation. Note the rightward shift of the loop in chronic aortic regurgitation (C), reflecting elevated LV volume without a dramatic elevation in filling pressure. In acute aortic regurgitation (A), LV volumes are also increased; however, the ventricle has not adapted to accommodate the increased volumes without elevation of filling pressures. [Modified from Jackson JM, Thomas SJ, Lowenstein E. Anesthetic management of patients with valvular heart disease. Semin Anesth. 1982;1:247.]

Full access? Get Clinical Tree
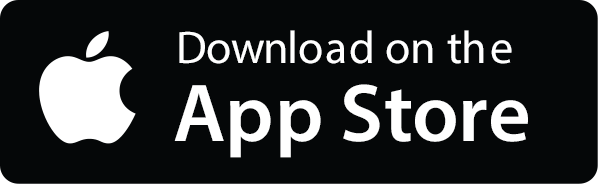
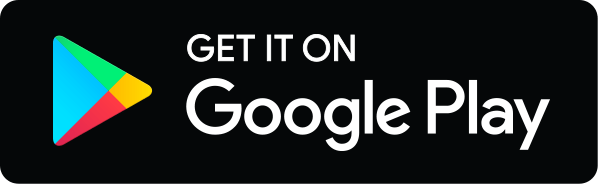