Anesthesia for Thoracic Surgery
James B. Eisenkraft
Edmond Cohen
Steven M. Neustein
Key Points











Related Matter
Position Double-lumen Tube
Bronchial Blocker
Univent
CPAP
Bronchoscopy
Jet Ventilation
Fiberoptic Intubation
Mediastinal Mass
Mediastinoscopy
Lung cancer has long been the most common cause of cancer mortality in the United States in men, and surpassed breast cancer as the leading cause of cancer deaths in women in 1987.1 The most recent statistics from the American Cancer Society indicated that approximately 221,130 new cases of lung cancer would be diagnosed in 2011 (115,060 among men and 106,070 among women). The Society also estimated that there would be 156,940 deaths from lung cancer, which represents 27% of all cancer deaths (Fig. 37-1).2 The increased incidence of lung cancer has led to an increase in the amount of noncardiac thoracic surgery performed in the United States.
In this chapter, the physiologic, pharmacologic, and clinical considerations for the patient undergoing pulmonary surgery are reviewed, followed by sections on anesthesia for diagnostic and therapeutic procedures, high-frequency ventilation, and special situations, including bronchopleural fistula (BPF) and tracheal reconstruction. A discussion of myasthenia gravis (MG) is included because of its relationship to the thymus gland and because thymectomy is one of the most commonly performed surgical procedures in these patients. The chapter concludes with a review of the postoperative management of the patient who has undergone noncardiac thoracic surgery.
Preoperative Evaluation

It is more difficult to predict postoperative pulmonary complications following elective cardiothoracic, compared with noncardiothoracic surgery.3 Thoracic surgery is known to be associated with high risk, and patient factors that have been associated with increased risk include advanced age, poor general health status, and chronic obstructive pulmonary disease (COPD).4
History
Dyspnea
Dyspnea occurs when the requirement for ventilation is greater than the patient’s ability to respond appropriately (see Chapter 11). Dyspnea is quantified by the degree of physical activity required to produce it, the level of activity possible (e.g., ability to walk on level ground or climb stairs), and management of daily activities. Severe exertional dyspnea usually implies a significantly diminished ventilatory reserve and a forced expiratory volume in 1 second (FEV1) of <1,500 mL, with possible need for postoperative ventilatory support.
Cough
Recurrent productive cough for 3 months of the year for two consecutive years is necessary to make the diagnosis of chronic bronchitis. Cough indirectly increases airway irritability. If the cough is productive, the volume, consistency, and color of the sputum should be assessed. Sputum should be cultured to rule out infection and to establish whether there is a need for preoperative antibiotic therapy. Blood-stained sputum or episodes of gross hemoptysis should alert the anesthesiologist to the possibility of a tumor invading the respiratory tract (e.g., the main stem bronchus), which might interfere with endobronchial intubation.
Cigarette Smoking
Cigarette smoking increases the risk of chronic lung disease and malignancy, as well as the incidence of postoperative pulmonary complications. The number of pack-years (packs smoked per day multiplied by the number of years) is directly related to measurable changes in respiratory gas flow and closing capacity,
making these patients prone to postoperative atelectasis and arterial hypoxemia.
making these patients prone to postoperative atelectasis and arterial hypoxemia.
Exercise Tolerance
Patients who can walk up three or more flights of stairs are at reduced risk, and those unable to climb two flights are generally at increased risk.5 The best evaluation is actually the history of the patient’s quality of life.6 An otherwise healthy patient, with good exercise tolerance, generally does not require additional screening tests.
Risk Factors for Acute Lung Injury
In some cases, thoracic surgery may lead to acute lung injury (ALI) postoperatively. Perioperative risk factors that have been identified include preoperative alcohol abuse and patients undergoing pneumonectomy. Intraoperative risk factors include high ventilatory pressures and excessive amounts of fluid administration.7
Physical Examination
The physical examination of the patient should address the following aspects.
Respiratory Pattern
The presence of cyanosis and clubbing, the breathing pattern, and the type of breath sounds should be noted.
Cyanosis. The presence of peripheral cyanosis (in the fingers, toes, or ears) should be distinguished from causes of poor circulation (acrocyanosis). The presence of central cyanosis (in the buccal mucosa) is usually secondary to arterial hypoxemia. If cyanosis is present, the arterial hemoglobin saturation with oxygen is 80% or less (PaO2 <50 to 52 mm Hg), which indicates a limited margin of respiratory reserve.
Clubbing. Clubbing of fingers and toes is often seen in patients with chronic lung disease, malignancies, or congenital heart disease associated with right-to-left shunt.
Respiratory Rate and Pattern. A patient’s inability to complete a normal sentence without pausing for breath is an indication of severe dyspnea. Inspiratory paradox, the abdomen moving in while the chest moves out, suggests diaphragmatic fatigue and respiratory dysfunction. The patient should be assessed for paroxysmal retraction (Hoover’s sign), limited diaphragmatic movement because of hyperinflation, asymmetry of chest movement secondary to phrenic nerve involvement, hemothorax, pleural effusion, and pneumothorax. The pattern and rate of breathing have important roles in distinguishing between obstructive and restrictive lung diseases. For constant minute ventilation, the work done against airflow resistance decreases when breathing is slow and deep. Work done against elastic resistance decreases when breathing is rapid and shallow (e.g., as in pulmonary infarct or pulmonary fibrosis).
Breath Sounds. Wet sounds (crackles) are usually caused by excessive fluid in the airways and indicate sputum retention or edema. Dry sounds (wheezes) are produced by high-velocity gas flow through bronchi and are a sign of airways obstruction. Distant sounds are an indication of emphysema and possibly bullae. The trachea should be in the midline. Displacement of the trachea may be secondary to a number of causes, including mediastinal mass, and should alert the anesthesiologist to a potentially difficult intubation of the trachea or airway obstruction on induction of anesthesia.
Evaluation of the Cardiovascular System
One of the most important factors in the evaluation of a patient scheduled for thoracic surgery is the presence of an increase in pulmonary vascular resistance secondary to a fixed reduction in the cross-sectional area of the pulmonary vascular bed. The pulmonary circulation is normally a low-pressure, high-compliance system capable of handling an increase in blood flow by recruitment of normally underperfused vessels. This acts as a compensatory mechanism that normally prevents an increase in pulmonary arterial pressure. In COPD, there is distention of the pulmonary capillary bed with decreased ability to tolerate an increase in blood flow (decreased compliance). Such patients demonstrate an increase in pulmonary vascular resistance when cardiac output increases because of a decreased ability to compensate for an increase in pulmonary blood flow. This results in pulmonary hypertension, signs of which include a narrowly split second heart sound, increased intensity of the pulmonary component of the second heart sound, and right ventricular and atrial hypertrophy. An increase in pulmonary vascular resistance is of significance in the management of the patient during anesthesia because several factors, such as acidosis, sepsis, hypoxia, and application of positive end-expiratory pressure (PEEP), all further increase the pulmonary vascular resistance and increase the likelihood of right ventricular failure.
In patients with ischemic or valvular heart disease, the function of the left side of the heart should also be carefully evaluated.
Electrocardiogram
A patient with COPD may present with electrocardiographic features of right atrial and ventricular hypertrophy and strain. These include a low-voltage QRS complex due to lung hyperinflation and poor R-wave progression across the precordial leads. An enlarged P wave (“P pulmonale”) in standard lead II is diagnostic of right atrial hypertrophy. The electrocardiographic changes of right ventricular hypertrophy are an R/S ratio of greater than 1 in lead V1 (i.e., R-wave voltage exceeds S-wave voltage).
Chest Radiography
Hyperinflation and increased vascular markings are usually present with COPD. Prominent lung markings often occur in bronchitis they are decreased in emphysema, particularly at the bases, where actual bullae may be present in severe cases. Hyperinflation, with an increased anteroposterior chest diameter, may be present, together with an enlarged retrosternal air space of >2 cm in diameter seen in a lateral chest radiograph.
The location of the lung lesion should be assessed by posteroanterior and lateral projections on chest radiography. In addition to tracheal or carinal shift, a mediastinal mass may indicate difficulty with ventilation, a difficult and bloody dissection, difficulty in placing a double-lumen tube (DLT; because of deviation of the main stem bronchus), or a collapsed lobe owing to bronchial obstruction with possible sepsis. Review of a computed tomography (CT) study is also useful, and often provides more information about tumor size and location than the chest radiograph.
Arterial Blood Gas Analysis
A common finding in arterial blood gas analysis of patients with COPD is hypoventilation and CO2 retention. The “blue bloaters” (chronic bronchitis) are cyanotic, hypercarbic, hypoxemic, and usually overweight. They are in a state of chronic respiratory failure and have a decreased ventilatory response to CO2. In these
patients, the high PaCO2 increases cerebrospinal fluid bicarbonate concentration, the medullary chemoreceptors become reset to a higher level of CO2, and sensitivity to CO2 is decreased. Such patients hypoventilate when given high oxygen concentrations to breathe because of a decreased hypoxic drive.
patients, the high PaCO2 increases cerebrospinal fluid bicarbonate concentration, the medullary chemoreceptors become reset to a higher level of CO2, and sensitivity to CO2 is decreased. Such patients hypoventilate when given high oxygen concentrations to breathe because of a decreased hypoxic drive.
The “pink puffers” (patients with emphysema) are typically thin, dyspneic, and pink, with essentially normal arterial blood gas values. They present with an increase in minute ventilation to maintain their normal PaCO2, which explains the increase in work of breathing and dyspnea. The preoperative PaO2 correlates with the intraoperative PaO2 during one-lung ventilation (OLV), but the intraoperative PaO2 during two-lung ventilation correlates more closely.8
Pulmonary Function Testing and Evaluation for Lung Resectability
There are three goals in performing pulmonary function tests in a patient scheduled for lung resection. The first goal is to identify the patient at risk of increased postoperative morbidity and mortality. In thoracic surgery for lung cancer, the specific question is: How much lung tissue may be safely removed without making the patient a pulmonary cripple? This should be weighed against the 1-year mean survival rate of the patient with surgically untreated lung carcinoma. The second goal is to identify the patient who will need short-term or long-term postoperative ventilatory support. The third goal is to evaluate the beneficial effect and reversibility of airway obstruction with the use of bronchodilators.
Effects of Anesthesia and Surgery on Lung Volumes
Anesthesia and postoperative medications can cause changes in lung volumes and ventilatory pattern. Total lung capacity (TLC) decreases after abdominal surgery but not after surgery on an extremity. Vital capacity is decreased by 25% to 50% within 1 to 2 days after surgery and generally returns to normal after 1 to 2 weeks. Residual volume (RV) increases by 13%, whereas expiratory reserve volume decreases by 25% after lower abdominal surgery and 60% after upper abdominal and thoracic surgery. Tidal volume (VT) decreases by 20% within 24 hours after surgery and gradually returns to normal after 2 weeks. Pulmonary compliance decreases by 33% with similar reductions in functional residual capacity (FRC) secondary to small airway closure. Most of the patients who undergo lung resection are smokers with a certain degree of COPD they are prone to postoperative complications in direct relation to the amount of lung to be resected (lobectomy or pneumonectomy) and to the severity of the preoperative lung disease.
Spirometry

FEV1 is a more direct indication of airway obstruction. In the past, an FEV1 of <800 mL in a 70-kg man had been considered an absolute contraindication to lung resection. However, with the advent of thoracoscopic surgery and improved postoperative pain management, patients with smaller lung volumes are now successfully undergoing surgery. It is preferable to indicate the percentage of predicted value, rather than just using the actual results in liters. The percentage of predicted value takes into account the age and size of the patient, and the same number may have a different implication in another patient. The ratio FEV1/FVC is useful in differentiating between restrictive and obstructive pulmonary diseases. It is normal in restrictive disease because both FEV1 and FVC decrease, whereas in obstructive disease the ratio is usually low because the FEV1 is markedly decreased. MVV is a nonspecific test and is an indicator of both restriction and obstruction. Although MVV has not been systematically evaluated as a predictor of morbidity, it is generally accepted that an MVV <50% of predicted value is an indication of high risk. A ratio of RV to TLC (RV/TLC) of >50% is generally indicative of a high-risk patient for pulmonary resection. By multiplying the preoperative FEV1 by the percentage of lung tissue expected to remain following resection, a predicted postoperative FEV1 can be calculated. Patients with a predicted postoperative FEV1 value >40% are at reduced risk and those with predicted postoperative FEV1 <30% are at increased risk.10 Those patients who fall into the latter category are more likely to need postoperative ventilation.
Flow–Volume Loops
The flow–volume loop displays essentially the same information as a spirometer but is more convenient for measurement of specific flow rates (Fig. 37-2). The shape and peak airflow rates during expiration at high lung volumes are effort dependent, but indicate the patency of the larger airways. Effort-independent expiration occurs at low lung volumes and usually reflects small airway resistance, best measured by forced expiratory flow (FEF) during the middle half of the FVC (FEF25–75%).
In general, patients with obstructive airway disease (Fig. 37-3), such as asthma, bronchitis, and emphysema, have grossly decreased FEV1/FVC ratios because of increased airway resistance
and a decrease in FEV1. Peak expiratory flow rate and MVV are usually decreased, whereas TLC increases secondary to increases in RV. In these patients, the effort-independent portion of the flow–volume curve is markedly depressed inward, with reduction of the flow rate at 25% to 75% of FVC.
and a decrease in FEV1. Peak expiratory flow rate and MVV are usually decreased, whereas TLC increases secondary to increases in RV. In these patients, the effort-independent portion of the flow–volume curve is markedly depressed inward, with reduction of the flow rate at 25% to 75% of FVC.
In patients with restrictive disease (Fig. 37-3), such as pulmonary fibrosis and scoliosis, there is a decrease in FVC with a relatively normal FEV1. Because the airway resistance is normal, FEV1/FVC is also normal. TLC is markedly decreased, whereas MVV and FEF25–75% are usually normal. The flow–volume curves of these patients are normal in shape, but the lung volumes and peak flow rates are decreased.
Significance of Bronchodilator Therapy. Pulmonary function tests are usually performed before and after bronchodilator therapy to assess the reversibility of the airway obstruction. This is useful in the assessment of the degree of airway obstruction and the patient’s effort ability. After treatment with bronchodilators, increases in peak expiratory flow compared with a baseline indicate reversibility of airway obstruction (often seen in asthmatic patients). A 15% improvement in pulmonary function tests may be considered a positive response to bronchodilator therapy and indicates that this therapy should be initiated before surgery. The overall prognosis of COPD is better related to the level of spirometric function after bronchodilator therapy than to a baseline function.
Split-lung Function Tests
Regional lung function studies serve to predict the function of the lung tissue that would remain after lung resection. A whole (two)-lung test may fail to estimate whether the amount of postresection lung tissue will allow the patient to function at a reasonable level of activity without disabling dyspnea or cor pulmonale.
Regional Perfusion Test. This involves the intravenous injection of insoluble radioactive xenon (133Xe). The peak radioactivity of each lung is proportional to the degree of perfusion of each lung.
Regional Ventilation Test. Using an inhaled, insoluble radioactive gas, the peak radioactivity over each lung is proportional to the degree of ventilation. Combining radiospirometry with whole-lung testing (FEV1, FVC, maximal breathing capacity) has resulted in a fair degree of correlation between predicted volumes and pulmonary function tests measured after pneumonectomy.
Computed Tomography and Positron Emission Tomography Scans. Patients normally undergo CT scanning. The CT scan provides anatomic sections through the chest and can delineate the size of the tumor. It can also reveal if there is airway or cardiovascular compression.
Positron emission tomography (PET) scans use a glucose analog that is labeled with a radionuclide positron emitter. This scan can detect tumor based on the metabolic activity. Because malignant tumors are growing at such a fast rate compared with healthy tissue, the tumor cells will use up more of the sugar that has the radionuclide attached to it. There is greater uptake by malignant mediastinal lymph nodes than benign nodes. PET may be more accurate than CT for mediastinal staging.11 Currently, PET scans can be used to further evaluate lesions that are seen on a CT scan. The PET scan can also be used to follow the results of lung cancer treatments.12
The CT and PET scans can be done at the same time to produce a PET–CT scan. A mass that is seen on the CT scan is more likely to be malignant if it also demonstrates enhanced glucose uptake on the PET scan.
Diffusing Capacity for Carbon Monoxide
The ability of the lung to perform gas exchange is reflected by the diffusing capacity for carbon monoxide. It is impaired in such disorders as interstitial lung disease, which affects the alveolar-capillary site. A predicted postoperative diffusing capacity for carbon monoxide <40% is associated with increased risk. Predicted postoperative diffusing capacity percent is the strongest single predictor of risk of complications and mortality after lung resection. There is little interrelationship of predicted postoperative diffusing capacity percent and predicted postoperative FEV1, indicating that these values should be assessed independently when estimating operative risk.13 In a recent study of 956 patients, a lower DLCO ppo (diffusing capacity for carbon monoxide, predicted postoperative) and the preoperative administration of chemotherapy, were found to be predictive of postoperative complications. In that study, FEV1 was not found to be predictive of complications.14 In another study, the DLCO ppo was the most predictive factor for postoperative morbidity and mortality.15
Maximal Oxygen Consumption. The maximal oxygen consumption (VO2 max) is a predictor of postoperative complications. Patients with a VO2 max >15 to 20 mL/kg/min are at reduced risk.16 A VO2 max <10 mL/kg/min indicates very high risk for lung resection.15,17 A simpler test that can be performed is exercise oximetry—a decrease of 4% during exercise is associated with increased risk.18 A 6-minute walk test <2,000 feet has been correlated both with a VO2 max <15 mL/kg/min and with a decrease in oximetry reading during exercise. It has been suggested that the percentage of predicted VO2 max may be a better indicator for risk, and a threshold of 50% to 60% could be established without an increase in surgical mortality.19 Brunelli and Fianchini20 had patients climb the maximum number of stairs possible. On the basis of the results of this study, these authors recommended that patients who were able to climb >14 m can safely undergo surgery, and those who were able to climb <12 m, with predicted postoperative function FEV1 <35% not be considered for major lung resection. The inability to do a maximal stair climbing has been correlated with an increased mortality following major lung resection.21 The preoperative evaluation of the patient for lung resection is summarized in Figure 37-4.
Preoperative Preparation
The wide spectrum of physiologic changes that occur during thoracic surgery puts patients at great risk of developing postoperative complications. Morbidity and mortality increase when these changes are superimposed on an acutely or chronically compromised patient. Several conditions, including infection, dehydration, electrolyte imbalance, wheezing, obesity, cigarette smoking, cor pulmonale, and malnutrition, show particular correlations with postoperative complications. Proper, vigorous preoperative preparation can improve the patient’s ability to face the surgery with a decreased risk of morbidity and mortality. It is important that conditions predisposing to postoperative complications be rigorously treated before surgery.
Smoking

Infection
Acute or chronic infection should be vigorously treated before surgery. Broad-spectrum antibiotics are commonly used. Treatment of the acutely ill patient depends on the results of the Gram stain of the sputum and blood cultures. Unless there are other modifying circumstances such as allergic history or patients are already receiving antibiotics, cefazolin is routinely administered perioperatively. To be most effective, it needs to be given prior to skin incision.25 In one prospective study, the incidence of mortality was lower in the group treated with prophylactic antibiotics compared with the untreated group (9% vs. 17%), and a lower incidence of postoperative pulmonary infection was also found.26 Although not all surgeons routinely administer antibiotics prophylactically to their patients, any infection present before surgery should be vigorously treated.
Hydration and Removal of Bronchial Secretions
Correction of hypovolemia and electrolyte imbalance should be accomplished before surgery because adequate hydration decreases
the viscosity of bronchial secretions and facilitates their removal from the bronchial tree. Humidification of inspired gas is extremely useful. The use of mucolytic drugs, such as acetylcysteine (Mucomyst), or oral expectorants (potassium iodide) can be beneficial to patients with viscous secretions. Commonly used methods for removing secretions from the bronchial tree include postural drainage, vigorous coughing, chest percussion, deep breathing, and the use of an incentive spirometer. These modalities often require patient cooperation and frequent verbal encouragement to maximize the benefit.
the viscosity of bronchial secretions and facilitates their removal from the bronchial tree. Humidification of inspired gas is extremely useful. The use of mucolytic drugs, such as acetylcysteine (Mucomyst), or oral expectorants (potassium iodide) can be beneficial to patients with viscous secretions. Commonly used methods for removing secretions from the bronchial tree include postural drainage, vigorous coughing, chest percussion, deep breathing, and the use of an incentive spirometer. These modalities often require patient cooperation and frequent verbal encouragement to maximize the benefit.
Wheezing and Bronchodilation
The presence of acute wheezing represents a medical emergency, and elective surgery should be postponed until effective treatment has been instituted. Chronic wheezing is often seen in patients with COPD and is attributable to the presence of gas flow obstruction secondary to smooth muscle contraction, accumulation of secretions, and mucosal edema. Smooth muscle contraction may occur in small airways only (detectable by changes in FEF25–75%) or may be widespread, with a large reduction of FEV1 and FVC. The efficacy of bronchodilators in reversing the bronchospastic component is extremely important. A trial of bronchodilators and measurement of their effects on pulmonary function should be performed in any patient who shows evidence of air flow obstruction. Several classes of bronchodilators are available.
Sympathomimetic Drugs
Sympathomimetic drugs increase the formation of 3′,5′-cyclic adenosine monophosphate (cAMP). The balance between cAMP, which produces bronchodilation, and cyclic guanosine monophosphate, which produces bronchoconstriction, determines the state of contraction of the bronchial smooth muscle. Increasing cAMP production therefore causes relaxation of the bronchial tree. Sympathomimetic drugs, such as epinephrine, isoproterenol, isoetharine, and ephedrine, all have mixed β1 and β2 sympathetic agonist effects. The β1 (cardiac effects) of these drugs are often undesirable in patients with COPD. Selective β2 sympathomimetic drugs, such as albuterol, terbutaline, and metaproterenol, given as inhaled aerosols, are the preferred drugs for the treatment of bronchospasm, particularly in patients with cardiac disease.
Phosphodiesterase Inhibitors
Phosphodiesterase inhibitors inhibit the breakdown of cAMP by cytoplasmic phosphodiesterase. The methylxanthines, such as aminophylline, increase the level of cAMP, resulting in bronchodilation. In addition, aminophylline improves diaphragmatic contractility and increases the patient’s resistance to fatigue. Therapeutic blood levels of aminophylline are 5 to 20 μg/mL and can be achieved by infusing a loading dose of 5 to 7 mg/kg over 20 minutes, followed by a continuous intravenous infusion of 0.5 to 0.7 mg/kg/hr. Aminophylline may cause ventricular dysrhythmias, and this side effect should be borne in mind when treating patients who have myocardial ischemia. Because newer medications have fewer side effects; aminophylline is now rarely used.
Steroids
Although not true bronchodilators, steroids are traditionally considered to decrease mucosal edema and may prevent the release of bronchoconstricting substances. They are of questionable benefit in acute bronchospasm. Steroids may be administered orally, parenterally, or in aerosol form, such as beclomethasone by inhaler.
Cromolyn Sodium
Cromolyn sodium stabilizes mast cells and inhibits degranulation and histamine release. It is useful in the prevention of bronchospastic attacks but is of little value in the treatment of the acute situation (see Chapter 12).
Parasympatholytic Drugs
Parasympatholytics include atropine and ipratropium (see Chapter 15). In the past, atropine has been avoided in patients with COPD and bronchitis because of the concern regarding increases in the viscosity of mucus produced by this agent. However, atropine blocks the formation of cyclic guanosine monophosphate and therefore has a bronchodilator effect.
Pulmonary Rehabilitation
Sekine et al.26 reported that pulmonary rehabilitation led to reduced hospital stay and improved postoperative FEV1, compared with a historical control group. The pulmonary rehabilitation included education in a variety of areas such as breathing, exercise, and nutrition.
Intraoperative Monitoring
All patients undergoing anesthesia for thoracic surgical procedures require adherence to the Standards of Basic Anesthetic Monitoring American Society of Anesthesiologists (ASA) (see Chapter 25). In particular, these include an electrocardiogram (lead II and, if possible, V5), chest or esophageal stethoscopes for heart and breath sound auscultation, and a temperature probe. A chest stethoscope may be placed over the dependent hemithorax to assess dependent lung ventilation. Pulse oximetry, which is a standard of care, is especially valuable during thoracic surgery because hypoxemia may occur during OLV.
Dysrhythmias occur commonly both during and after thoracic surgery, making the usual need for continuous electrocardiographic monitoring even more important. Intraoperative supraventricular tachyarrhythmias may be caused by cardiac manipulation. Dysrhythmias that occur during OLV may be a sign of inadequate oxygenation or ventilation. Postoperative dysrhythmias may be related to sympathetic nervous system stimulation from pain or to a decreased pulmonary vascular bed following lung resection. Patients who present for lung resection often have COPD due to cigarette smoking, have right-sided heart strain, and are prone to multifocal atrial tachyarrhythmias.
The axis of electrocardiogram lead II parallels that of the P wave, making this lead useful for dysrhythmia detection. The simultaneous monitoring of lead V5 also allows for monitoring of anterolateral wall myocardial ischemia. The use of multiple leads increases the sensitivity for ischemia detection.27 The following invasive monitors are also indicated and have led to marked improvements in patient care.
Direct Arterial Catheterization
Peripheral arterial catheterization has become an essential tool for the anesthesiologist in the management of patients undergoing major thoracic surgical procedures (see Chapter 25). It allows for continuous beat-to-beat measurement of blood pressure and frequent sampling for the determination of arterial blood gases.
Continuous blood pressure readings are critical during thoracic surgery because surgical manipulations may result in cardiac compression and there may be sudden bleeding. Immediate recognition of these changes allows time for proper identification of the etiology and the institution of appropriate treatment.
Continuous blood pressure readings are critical during thoracic surgery because surgical manipulations may result in cardiac compression and there may be sudden bleeding. Immediate recognition of these changes allows time for proper identification of the etiology and the institution of appropriate treatment.
Serial arterial blood gas analyses are performed as needed in the management of patients undergoing one-lung anesthesia or during cases in which a part of the lung may be “packed away” for a period. Arterial hypoxemia may occur because of shunting of mixed venous through the collapsed lung and an inadequate hypoxic pulmonary vasoconstriction (HPV) response. Significant changes in acid–base status and hyperventilation or hypoventilation can also be identified.
A radial artery catheter (see Chapter 25) can be placed in either extremity during thoracic surgery. For a mediastinoscopic examination, one approach is to place the catheter in the right arm and to use it to monitor for possible compression of the innominate artery by the mediastinoscope. This can help avoid central nervous system complications that might result from inadequate cerebral blood flow via the right carotid artery (see “Mediastinoscopy”). The other approach would be to place the arterial catheter in the left radial artery, allowing for continuous blood pressure measurements, uninterrupted by innominate artery compression. If this is done, a pulse oximeter probe should be placed on the right upper extremity to monitor for innominate artery compression. During thoracotomy, placement of the arterial catheter in the dependent arm can be used to monitor for possible axillary artery compression, which may occur if the patient is not properly positioned. For a brief thoracoscopy case in a relatively healthy patient, it would be acceptable to proceed without an arterial catheter, as long as the pulse oximeter is functioning reliably. Such an example might be a healthy patient presenting for bilateral VAT sympathectomy for hyperhidrosis.
The patient undergoing a pulmonary resection, and especially a right pneumonectomy, is at risk for postoperative pulmonary edema. It is especially important to not fluid overload such a patient, as the likelihood of postoperative edema is greater with increased intraoperative fluid administration. Prior to its administration it would be preferable to be able to identify which patients would be likely to respond favorably to a fluid bolus. The central venous pressure (CVP) may not accurately reflect intravascular volume status, and is no longer recommended as a guide for fluid responsiveness.28 Systolic pressure variation (SPV) and pulse pressure variation (PPV) have been reported as being able to predict fluid responsiveness.29 In a recent paper, a PPV greater than 13% predicted fluid responsiveness, less than 9% predicted that the patient would not be responsive, and 9% to 13% reflected a gray zone.30 Stroke volume variation has been reported to predict fluid responsiveness, specifically in patients undergoing thoracic surgery, during OLV.31
Central Venous Pressure Monitoring
The CVP may reflect the patient’s blood volume, venous tone, and right ventricular performance; however, it is also affected by central venous obstructions and alterations of intrathoracic pressure such as PEEP (see Chapter 25). The CVP reflects right-sided heart function, not left ventricular performance. Catheters for measuring CVP may be placed for thoracotomies, and in particular, patients undergoing pneumonectomy. Uses of CVP catheters or large-bore introducers include (1) insertion of a transvenous pacemaker where necessary, (2) infusion of vasoactive drugs, and (3) insertion of a pulmonary artery (PA) catheter, which may subsequently be required during surgery or in the postoperative period. A recent study in healthy subjects indicated that, contrary to common belief, the CVP did not reflect intravascular volume status.32
The CVP catheter can be placed centrally from either the external or the internal jugular vein, from the subclavian veins, or from one of the arm veins. The success rate is highest using the right internal jugular vein, and a pacemaker or PA catheter can be inserted most easily from this vein. The major disadvantage of using the external jugular vein during thoracotomy is that the catheter often kinks when the patient is turned to the lateral decubitus position. The subclavian technique leads to a higher incidence of pneumothorax, which can be disastrous if it occurs in the dependent lung during OLV. If necessary and if possible, a subclavian catheter should be placed ipsilateral to the surgery. As discussed above, the CVP is no longer considered an accurate guide for fluid responsiveness. However, it is a common practice among thoracic anesthesiologists to place a CVP catheter for certain thoracic cases such as esophagectomy and pneumonectomy.
Pulmonary Artery Catheterization
The PA catheter is most reliably inserted through the right internal jugular vein using a modified Seldinger technique (see Chapter 25). Insertion of the PA catheter through either the external jugular vein or the subclavian vein often leads to obstruction of the catheter when the patient is placed in the lateral decubitus position. Misinterpretation of data from a PA catheter is a real risk in a patient with cardiac and pulmonary diseases undergoing thoracic surgery with OLV. These errors can be produced by altered ventilatory modes, the location of the PA catheter tip, ventricular compliance changes, or ventricular interdependence.33 A major limitation of the PA catheter is the assumption that the pulmonary capillary wedge pressure (PCWP) provides a good approximation of left ventricular end-diastolic volume. The use of PCWP directly to assess preload assumes a linear relationship between ventricular end-diastolic volume and ventricular end-diastolic pressure. However, alterations in ventricular compliance affect this pressure–volume relationship during surgery. Decreases in ventricular compliance can occur with myocardial ischemia, shock, right ventricular overload, or pericardial effusion. Numerous investigators have demonstrated a poor correlation between PCWP and left ventricular end-diastolic volume in acutely ill patients.34 This correlation is further worsened by the application of PEEP. In addition, ventricular interdependence can cause misdiagnosis when the interventricular septum encroaches on the left ventricular cavity, leading to increased values of PCWP. A PCWP associated with a decreased cardiac output can be interpreted as left ventricular failure, when in fact, left ventricular end-diastolic volume may not be increased but decreased because of compression of the left ventricle by a distended right ventricle. This situation can occur with acute respiratory failure and high levels of PEEP. Techniques such as echocardiography, which directly measure ventricular dimensions, may facilitate resolution of this complex situation.
Because most of the pulmonary blood flow is to the right lower lobe, the tip of a flow-directed PA catheter is usually located in the right lower lobe. During a left thoracotomy with OLV, the catheter tip would then be in the dependent lung and should provide accurate hemodynamic measurements. However, during a right thoracotomy with OLV, the catheter tip would most likely be in the nondependent lung, and may not be accurate. The use of intraoperative mean pulmonary artery pressure has been reported to be an indicator of safety for lung resection under thoracotomy.35 The authors concluded that following occlusion of the main PA, upper safety limits of 33 mm Hg for right, and 35 mm Hg for left thoracotomy could be used. The authors noted that the difference
between sides was minimal, and less than expected. The monitoring of Sv-o2 has been evaluated in patients undergoing one-lung anesthesia.36 Changes in Sv-o2 were mainly dependent on changes in SaO2. Currently, the use of the PA catheter for monitoring during thoracic surgery is generally unnecessary, and may be reserved for patients with pulmonary hypertension.
between sides was minimal, and less than expected. The monitoring of Sv-o2 has been evaluated in patients undergoing one-lung anesthesia.36 Changes in Sv-o2 were mainly dependent on changes in SaO2. Currently, the use of the PA catheter for monitoring during thoracic surgery is generally unnecessary, and may be reserved for patients with pulmonary hypertension.
Transesophageal Echocardiography
Transesophageal echocardiography (TEE) is a useful intraoperative monitor for ventricular function, valvular function, and wall motion changes that might reflect ischemia (see Chapter 26). Its use in thoracic surgical patients has been limited, but it is widely used in patients undergoing lung transplant. The use of TEE requires special training, and may not be available at all centers. A recent review concluded that although the intraoperative use of TEE is not routinely indicated, it may be useful for diagnosing right ventricular dysfunction, in the setting of hypotension or arrhythmias following lung resection.37 Right ventricular dysfunction may occur during OLV, clamping of the pulmonary artery for either pneumonectomy, or during lung transplantation. TEE may be used to help determine if it is necessary to utilize cardiopulmonary bypass during lung transplantation.38
TEE may be useful in visualizing hilar lung tumors, and evaluating possible extension into the heart. In one study, central lung tumors were seen with TEE in nine of the nine patients, peripheral lung tumors in one of the three patients, and an anterior mediastinal mass in one of one patient.39 In this study, TEE revealed PA compression in five patients and PA infiltration in two patients. In another study investigating echocardiographic recognition of mediastinal tumors, TEE revealed that the tumors were often adjacent to the heart and identified those patients in whom there was compression of the innominate vein or PA, or infiltration of the heart.40
Intraoperative TEE has also revealed tumor invasion of the heart, indicating that a resection by thoracotomy without cardiopulmonary bypass was not feasible.41 In one case report, TEE monitoring during an attempted resection of a tumor invading the left atrium showed embolization of the tumor.42 Fragments of the tumor were seen to pass through the aortic valve. This patient subsequently died of disseminated metastases. In an exploratory thoracotomy for hemothorax, intraoperative TEE revealed the presence of a subacute aortic dissection, which was believed to be the cause of the hemothorax.43 TEE was used intraoperatively to evaluate a large anterior mediastinal mass, providing data on right ventricular outflow compression, and ventricular contractility and filling status.44 In another recent report, a mediastinal mass was diagnosed intraoperatively using TEE; in that case, the mass had been misdiagnosed preoperatively with transthoracic echocardiography as a pericardial effusion.45
Additional Noninvasive Monitoring
Although data are presently limited, it has been reported that decreased cerebral oximetry values by absolute cerebral oximetry during OLV have been correlated with postoperative complications.46 In a subsequent study, the larger decreases in cerebral oxygen saturation occurred in patients with better preoperative lung function.47 At this time, the data are still too limited to recommend cerebral oximetry as a routine monitor during thoracic surgery.
A recent meta-analysis of the use of noninvasive cardiac output measurements during surgery revealed poor agreement with thermodilution. Noninvasive cardiac output measurements are not commonly utilized during thoracic surgery.48 The use of noninvasive cardiac output monitoring for thoracic surgery is not recommended at this time.
Monitoring of Oxygenation and Ventilation
Oxygenation
During the administration of all thoracic surgical anesthetics, the concentration of inspired oxygen in the breathing system must be measured using an oxygen analyzer with a low oxygen concentration limit alarm (see Chapter 24). Such analyzers vary in sophistication from fuel cells to rapidly responding paramagnetic analyzers that monitor oxygen breath-by-breath and display an oxygram (analogous to, and a mirror image of, the capnogram). Adequacy of blood oxygenation must also be ensured, and adequate illumination and exposure of the patient are helpful to assess the color of shed blood or the presence of cyanosis of the lips, nail beds, or mucous membranes. Most patients undergoing thoracic surgical or diagnostic procedures have an arterial catheter in place for continuous monitoring of blood pressure and sampling of arterial blood for blood gas analyses.
Pulse oximetry is now a standard of care for noninvasive assessment of blood oxygenation. The use of pulse oximetry is especially important during OLV, when rapid assessment of oxygenation is critical. A low SpO2 reading provides the clinician with an indication for blood gas sampling and laboratory analysis of arterial blood. The traditional two-wavelength pulse oximeter may display spurious readings of SpO2 in the presence of dyshemoglobins, methemoglobin and carboxyhemoglobin. Multiwavelength (8 or 12 wavelengths) pulse oximeters are now commercially available that are capable of measuring carboxyhemoglobin, methemoglobin, deoxygenated hemoglobin, and oxygenated hemoglobin (HbO2%).49,50 In addition, continuous monitoring of total hemoglobin concentration is now available.51
Ventilation
All patients must be continually monitored to ensure adequacy of ventilation. Monitoring includes qualitative signs such as chest excursion (visual observation of the lungs when the chest is open) and auscultation of breath sounds. In addition, during OLV, a stethoscope can be placed on the chest wall under the ventilated dependent lung. During controlled ventilation, circuit low-pressure and high-pressure alarms with an audible signal must be used. The respiratory rate, VT, minute volume, and inflation pressures should be observed.
Adequacy of ventilation should be confirmed by monitoring arterial blood gas analyses and PaCO2, in particular. This may be estimated continuously and noninvasively by using a capnograph (see Chapter 25). The end-tidal CO2 concentration represents alveolar CO2 (PACO2), which approximates PaCO2. There is normally a small arterial-to-alveolar CO2 difference (4 to 6 mm Hg), depending on alveolar dead space. The capnogram waveform is also helpful in diagnosing airway obstruction, incomplete relaxation,52 and even malposition of the DLT.53 During OLV, systemic hypoxemia is usually a greater problem than hypercarbia.54 This is because CO2 is approximately 20 times more diffusible than oxygen and PaCO2 is more dependent on ventilation, compared with PaO2, which is more dependent on perfusion.
Physiology of One-lung Ventilation
Physiology of the Lateral Decubitus Position. Ventilation and blood flow in the upright position are discussed in Chapters 11 and 28.
These variables will now be considered as they pertain to the lateral decubitus position under six circumstances that are encountered during thoracic surgery.
These variables will now be considered as they pertain to the lateral decubitus position under six circumstances that are encountered during thoracic surgery.
Lateral position, awake, breathing spontaneously, chest closed. In the lateral decubitus position, the distribution of blood flow and ventilation is similar to that in the upright position, but turned by 90 degrees (Fig. 37-5). Blood flow and ventilation to the dependent lung are significantly greater than that to the nondependent lung. Good [V with dot above]/[Q with dot above] matching at the level of the dependent lung results in adequate oxygenation in the awake patient who is breathing spontaneously. There are two important concepts in this situation. First, because perfusion is gravity-dependent, the vertical hydrostatic pressure gradient is smaller in the lateral than in the upright position; therefore, zone 1 is usually less extended. Second, in regard to ventilation, the dependent hemidiaphragm is pushed higher into the chest by the abdominal contents compared with the nondependent lung hemidiaphragm. During spontaneous ventilation, the conserved ability of the dependent diaphragm to contract results in an adequate distribution of VT to the dependent lung. Because most of the perfusion is to the dependent lung, the [V with dot above]/[Q with dot above] matching in this position is maintained similar to that in the upright position.
Lateral Position, Awake, Breathing Spontaneously, Chest Open. Controlled positive-pressure ventilation is the most common way to provide adequate ventilation and ensure gas exchange in an open-chest situation. Frequently, thoracoscopy is performed using intercostal blocks with the patient breathing spontaneously to allow proper lung examination. The thoracoscope provides an adequate seal of the open chest to prevent a “free” open-chest situation. Two complications can arise from the patient breathing spontaneously with an open chest. The first is mediastinal shift, usually occurring during inspiration (Fig. 37-6). The negative pressure in the intact hemithorax, compared with the less negative pressure of the open hemithorax, can cause the mediastinum to move vertically downward and push into the dependent hemithorax. The mediastinal shift can create circulatory and reflex changes that may result in a clinical picture similar to that of shock and respiratory distress. Sometimes, depending on the severity of the distress, the patient needs to be tracheally intubated immediately, with initiation of positive-pressure ventilation, and the anesthesiologist must be prepared to intubate in this position without disturbing the surgical field.
The second phenomenon is paradoxical breathing (Fig. 37-7). During inspiration, the relatively negative pressure in the intact hemithorax compared with atmospheric pressure in the open hemithorax can cause movement of air from the nondependent lung into the dependent lung. The opposite occurs during expiration. This gas movement reversal from one lung to the other represents wasted ventilation and can compromise the adequacy of gas exchange. Paradoxical breathing is increased by a large thoracotomy or by an increase in airway resistance in the dependent lung. Positive-pressure ventilation or adequate sealing of the open chest eliminates paradoxical breathing.
Lateral Position, Anesthetized, Breathing Spontaneously, Chest Closed. The induction of general anesthesia does not cause significant change in the distribution of blood flow, but it has an important impact on the distribution of ventilation. Most of the VT enters the nondependent lung, and this results in a significant [V with dot above]/[Q with dot above] mismatch. Induction of general anesthesia causes a reduction in the volumes of both lungs secondary to a reduction in FRC. Any reduction in volume in the dependent lung is of a greater magnitude
than that in the nondependent lung for several reasons. First, the cephalad displacement of the dependent diaphragm by the abdominal contents is more pronounced and is increased by paralysis. Second, the mediastinal structures pressing on the dependent lung or poor positioning of the dependent side on the operating table prevents the lung from expanding properly. The aforementioned factors will move lungs to a lower volume on the S-shaped volume–pressure curve (Fig. 37-8). The nondependent lung moves to a steeper position on the compliance curve and receives most of the VT, whereas the dependent lung is on the flat (noncompliant) part of the curve.
than that in the nondependent lung for several reasons. First, the cephalad displacement of the dependent diaphragm by the abdominal contents is more pronounced and is increased by paralysis. Second, the mediastinal structures pressing on the dependent lung or poor positioning of the dependent side on the operating table prevents the lung from expanding properly. The aforementioned factors will move lungs to a lower volume on the S-shaped volume–pressure curve (Fig. 37-8). The nondependent lung moves to a steeper position on the compliance curve and receives most of the VT, whereas the dependent lung is on the flat (noncompliant) part of the curve.
Lateral Position, Anesthetized, Breathing Spontaneously, Chest Open. Opening the chest has little impact on the distribution of perfusion. However, the upper lung is now no longer restricted by the chest wall and is free to expand, resulting in a further increase in [V with dot above]/[Q with dot above] mismatch as the nondependent lung is preferentially ventilated, owing to a now increased compliance.
Lateral position, anesthetized, paralyzed, chest open. During paralysis and positive-pressure ventilation, diaphragmatic displacement is maximal over the nondependent lung, where there is the least amount of resistance to diaphragmatic movement caused by the abdominal contents (Fig. 37-9). This further compromises the ventilation to the dependent lung and increases the [V with dot above]/[Q with dot above] mismatch.
OLV, anesthetized, paralyzed, chest open. During two-lung ventilation in the lateral position, the mean blood flow to the nondependent lung is assumed to be 40% of cardiac output, whereas 60% of cardiac output goes to the dependent lung (Fig. 37-10). Normally, venous admixture (shunt) in the lateral position is 10% of cardiac output and is equally divided as 5% in each lung. Therefore, the average percentage of cardiac output participating in gas exchange is 35% in the nondependent lung and 55% in the dependent lung.
OLV creates an obligatory right-to-left transpulmonary shunt through the nonventilated, nondependent lung because the [V with dot above]/[Q with dot above] ratio of that lung is zero. In theory, an additional 35% should be
added to the total shunt during OLV. However, assuming active HPV, blood flow to the nondependent hypoxic lung will be decreased by 50% and therefore is (35/2) = 17.5%. To this, 5% must be added, which is the obligatory shunt through the nondependent lung. The shunt through the nondependent lung is therefore 22.5% (Fig. 37-10). Together with the 5% shunt in the dependent lung, total shunt during OLV is 22.5% + 5% = 27.5%. This results in a PaO2 of approximately 150 mm Hg (FIO2 = 1).55
added to the total shunt during OLV. However, assuming active HPV, blood flow to the nondependent hypoxic lung will be decreased by 50% and therefore is (35/2) = 17.5%. To this, 5% must be added, which is the obligatory shunt through the nondependent lung. The shunt through the nondependent lung is therefore 22.5% (Fig. 37-10). Together with the 5% shunt in the dependent lung, total shunt during OLV is 22.5% + 5% = 27.5%. This results in a PaO2 of approximately 150 mm Hg (FIO2 = 1).55
Because 72.5% of the perfusion is directed to the dependent lung during OLV, the matching of ventilation in this lung is important for adequate gas exchange. The dependent lung is no longer on the steep (compliant) portion of the volume–pressure curve because of reduced lung volume and FRC. There are several reasons for this reduction in FRC, including general anesthesia, paralysis, pressure from abdominal contents, compression by the weight of mediastinal structures, and suboptimal positioning on the operating table. Other considerations that impair optimal ventilation to the dependent lung include absorption atelectasis, accumulation of secretions, and the formation of a transudate in the dependent lung. All these create a low [V with dot above]/[Q with dot above] ratio and a large P(A–a)O2 gradient.
One-Lung Ventilation
Absolute Indications for One-lung Ventilation

During the last several years video-assisted thoracoscopy (VAT) was introduced to clinical practice. Unlike conventional thoracoscopy, VAT allows for an extensive variety of diagnostic and therapeutic procedures. Improvements in video-endoscopic
surgical equipment and a growing enthusiasm for minimally invasive surgical approaches have contributed to its use. In most cases general anesthesia with OLV is required. The lung should be well collapsed to provide the surgeon with an optimal view of the surgical field, and to facilitate palpation of the lesion in the lung parenchyma. In addition, it is difficult to place the stapler on a lung that is not completely collapsed, and there is an increase in incidence of postoperative air leak in these circumstances. The increased use of VAT has significantly increased the number of procedures that require lung separation. In some institutions, 80% to 90% of the procedures are performed using the thoracoscopic approach. In modern anesthesia practice, VAT is an absolute indication for lung separation.
surgical equipment and a growing enthusiasm for minimally invasive surgical approaches have contributed to its use. In most cases general anesthesia with OLV is required. The lung should be well collapsed to provide the surgeon with an optimal view of the surgical field, and to facilitate palpation of the lesion in the lung parenchyma. In addition, it is difficult to place the stapler on a lung that is not completely collapsed, and there is an increase in incidence of postoperative air leak in these circumstances. The increased use of VAT has significantly increased the number of procedures that require lung separation. In some institutions, 80% to 90% of the procedures are performed using the thoracoscopic approach. In modern anesthesia practice, VAT is an absolute indication for lung separation.
Table 37-1. Indications for One-Lung Ventilation | |||
---|---|---|---|
|
Relative Indications for One-lung Ventilation
In clinical practice, a DLT is commonly used for a lobectomy or pneumonectomy; these represent relative indications for lung separation when performed through an open thoracotomy. Upper lobectomy, pneumonectomy, and thoracic aortic aneurysm repair are high-priority indications. These procedures are technically difficult, and optimal surgical exposure and a quiet operative field are highly desirable. Lower or middle lobectomy and esophageal resection are of lower priority. There are a number of additional procedures that have not been traditionally included as indications for OLV. Nevertheless, many surgeons are accustomed to operating with the lung collapsed for these cases. OLV minimizes lung trauma from retractors and manipulation, improves visualization of lung anatomy, and facilitates identification and separation of anatomic structures and lung fissures. These procedures include minimally invasive cardiac surgery, lung volume reduction, thoracic aneurysm repair, thoracic spinal procedures, mediastinal mass resection, thymectomies, and mediastinal lymph node dissection.
It is important to distinguish between the need for lung isolation versus lung separation.
Lung Isolation. Whenever the nondiseased lung is threatened with contamination by blood or pus from the diseased lung, the lungs must be isolated to prevent potentially life-threatening complications. Other indications are bronchopleural and bronchocutaneous fistulas because they offer a low-resistance pathway for the delivered VT during positive-pressure ventilation. Finally, during bronchopulmonary lavage for alveolar proteinosis or cystic fibrosis, protection of the contralateral lung from drowning is necessary. These situations, however, are relatively uncommon and in modern anesthesia practice constitute less than 10% of all thoracic procedures.
Lung Separation. All other indications for OLV can be considered as lung separations, in which there is no risk of contamination of the dependent lung. This includes all the relative indications that are primarily for surgical exposure. VAT for diagnostic and therapeutic procedures, which requires a well-collapsed lung, should also be included in this category.4,5,6 The majority of procedures where OLV is used are for lung separation; only a minority require lung isolation.56,57
Methods of Lung Separation
Double-lumen Endobronchial Tubes
Double-lumen endobronchial tubes are currently the most widely used means of achieving lung separation and OLV. There are several different types of DLT, but all are essentially similar in design in that two endotracheal tubes are “bonded” together. One lumen is long enough to reach a main stem bronchus, and the second lumen ends with an opening in the distal trachea. Lung separation is achieved by inflation of two cuffs: A proximal tracheal cuff and a distal bronchial cuff located in the main stem bronchus (see “Positioning Double-lumen Tubes”). The endobronchial cuff of a right-sided tube is slotted or otherwise designed to allow ventilation of the right upper lobe because the right main stem bronchus is too short to accommodate both the right lumen tip and a right bronchial cuff.
Robertshaw Tube. The Carlens tube (which had a carinal hook) was the first clinically available DLT and was used by pulmonologists for split function spirometry testing (Fig. 37-11A). Subsequently, the Robertshaw-design DLT (which lacked a carinal hook) was developed to facilitate thoracic surgery (Fig. 37-11B). This DLT is available in left-sided and right-sided forms. The absence of a carinal hook facilitates insertion. This tube design has the advantages of having D-shaped, large-diameter lumens that allow easy passage of a suction catheter, offer low resistance to gas flow, and have a fixed curvature to facilitate proper positioning and reduce the possibility of kinking. The original red rubber Robertshaw tubes were available in three sizes: Small, medium, and large. Red rubber tubes are rarely used now and have been replaced by clear, polyvinyl chloride (PVC) disposable Robertshaw-design DLTs. These are available in both right-sided and left-sided versions and in 35 French (Fr), 37 Fr, 39 Fr, and 41 Fr sizes. A 32-Fr left-sided DLT is available for small adults, and a 28 Fr for use in pediatric cases. The advantages of the disposable tubes include the relative ease of insertion and proper positioning as well as easy recognition of the blue color of the endobronchial cuff when fiberoptic bronchoscopy is used. Other advantages are the confirmation of the position on a chest radiograph using the radiopaque lines in the wall of the tube and the continuous observation of tidal gas exchange and respiratory moisture through the clear plastic. The right-sided endobronchial tube is designed to minimize occlusion of the opening of the right upper lobe bronchus. The right endobronchial cuff is doughnut-shaped and allows the right upper lobe ventilation slot to ride over the opening of the right upper lobe bronchus. The tube is also suitable for use in long-term ventilation in the intensive care unit (ICU) because it has a high-volume, low-pressure cuff. These disposable PVC tubes are generally considered the tubes of choice for achieving lung separation and OLV.58
A new rubber–silicone left-sided DLT, Silbronco (Silbronco DLT, Fuji Systems, Tokyo, Japan), was recently introduced into clinical practice. It has a D-shaped wire-reinforced lumen to maintain the tip at a 45-degree angle. The reinforced wall tends to prevent obstruction or kinking of the bronchial lumen, yet at the same time maintains flexibility. It is especially useful if the left main stem bronchus is angled at 90 degrees from the trachea, making it almost impossible to position a PVC DLT. This clinical scenario can be seen in patients who have previously undergone a left upper lobectomy and the expansion of the left lower lobe displaces the left main bronchus upward.42,59
As the left main bronchus is considerably longer than the right bronchus, there is a narrow margin of safety on the right main bronchus, with potentially a greater risk of upper lobe obstruction whenever a right-sided DLT is used. A left-sided DLT is preferred for both right- and left-sided procedures. A left-sided DLT was selected for 1,166 of the 1,170 patients in one report, and was used successfully in over 98% of those patients.43 The authors recommended selecting the largest DLT that will safely fit the bronchus. This will provide less resistance to ventilation and is less likely to dislocate.
Some authors have suggested using the patient height as a basis for selecting a DLT. However, the correlation between airway size and height is extremely poor.60 Tracheal and bronchial dimensions can be also directly measured from the chest radiograph
or chest CT scan. It is possible to measure the diameter of the left bronchus from the chest radiograph in almost 75% of patients. In patients in whom the left main bronchus cannot be directly measured, the left bronchial diameter can be accurately estimated by measuring tracheal width. The width of the left bronchus is directly proportional to tracheal width. The left bronchial width is estimated by multiplying the tracheal width by 0.68.61 Typically, most women will need a 37-Fr DLT and most men will be adequately managed with a 39-Fr DLT. In the past it was a more common practice to use the largest size DLT possible to avoid distal migration of the tube, and so that the pressure in the bronchial cuff could be minimized by needing less air for a seal. The common practice of fiberoptic bronchoscopy has lessened the risk of undetected distal placement or migration of the bronchial tip. A recent study demonstrated that the routine use of a 35-Fr DLT in adults regardless of height was not associated with an increase in hypoxemia or any other adverse clinical outcomes.62
or chest CT scan. It is possible to measure the diameter of the left bronchus from the chest radiograph in almost 75% of patients. In patients in whom the left main bronchus cannot be directly measured, the left bronchial diameter can be accurately estimated by measuring tracheal width. The width of the left bronchus is directly proportional to tracheal width. The left bronchial width is estimated by multiplying the tracheal width by 0.68.61 Typically, most women will need a 37-Fr DLT and most men will be adequately managed with a 39-Fr DLT. In the past it was a more common practice to use the largest size DLT possible to avoid distal migration of the tube, and so that the pressure in the bronchial cuff could be minimized by needing less air for a seal. The common practice of fiberoptic bronchoscopy has lessened the risk of undetected distal placement or migration of the bronchial tip. A recent study demonstrated that the routine use of a 35-Fr DLT in adults regardless of height was not associated with an increase in hypoxemia or any other adverse clinical outcomes.62
The depth required for insertion of the DLT correlates with the height of the patient. For any adult 170- to 180-cm tall, the average depth for a left-sided DLT is 29 cm. For every 10-cm increase or decrease in height, the DLT is advanced or withdrawn 1 cm.63

First the tracheal cuff should be inflated, and equal ventilation of both lungs established. If breath sounds are not equal, the tube is probably too far down, and the tracheal lumen opening is in a main stem bronchus or is lying at the carina. Withdrawal of the tube by 2 to 3 cm usually restores equal breath sounds. The second step is to clamp the right side (in the case of the left-sided tube) and remove the right cap from the connector. Then the bronchial cuff is slowly inflated to prevent an air leak from the bronchial lumen around the bronchial cuff into the tracheal lumen. This ensures that excessive pressure is not applied to the bronchus and helps avoid laceration. Inflation of the bronchial cuff rarely requires >2 mL of air. The third step is to remove the clamp and check that both lungs are ventilated with both cuffs inflated. This ensures that the bronchial cuff is not obstructing the contralateral hemithorax, either totally or partially. The final step is to clamp each side selectively and watch for absence of movement and breath sounds on the ipsilateral (clamped) side; the ventilated side should have clear breath sounds, chest movement that feels compliant, respiratory gas moisture with each tidal ventilation, and no gas leak. If peak airway pressure during two-lung ventilation is 20 cm H2O, it should not exceed 40 cm H2O for the same VT during OLV.
Other methods that have been used for ensuring the correct placement of a DLT include fluoroscopy, chest radiography, selective capnography, and use of an underwater seal. Determination of the presence of gas leaks when positive pressure is applied to one lumen of a DLT is easily done in the operating room (OR). If the bronchial cuff is not inflated and positive pressure is applied to the bronchial lumen of the DLT, gas leaks past the bronchial cuff and returns through the tracheal lumen. If the tracheal lumen is connected to an underwater seal system, gas will be seen to bubble up through the water. The bronchial cuff can then be gradually inflated until no gas bubbles are seen and the desired cuff seal pressure can be attained. This test is of extreme importance when absolute lung separation is needed, such as during bronchopulmonary lavage.

bronchial orifice should be identified. When a right-sided DLT is used, the carina should be visualized through the tracheal lumen but, more importantly, the orifice of the right upper lobe bronchus should be identified when the bronchoscope is passed through the right upper lobe ventilating slot of the DLT. Pediatric fiberoptic bronchoscopes are available in several sizes: 5.6, 4.9, and 3.6 mm in external diameter. The 4.9-mm diameter bronchoscope can be passed through DLTs of 37 Fr and larger. The 3.6-mm diameter bronchoscope is easily passed through all sizes of DLT. In general, it is recommended that the largest size that can pass through the lumen of a DLT be used because it provides better visualization and facilitates identification of the bronchial anatomy. Excellent fiberoptic images of the tracheobronchial tree can be seen by accessing the website thoracicanesthesia.com.
![]() Figure 37.12. Fiberoptic bronchoscopic view of the main carina (A), the “left bronchial carina” (B), and the right bronchus (C). Note the right upper lobe orifice (arrow). |
Problems of Malposition of the Double-lumen Tube. The use of a DLT is associated with a number of potential problems, the most important of which is malposition. There are several possibilities for tube malposition. The DLT may be accidentally directed to the side opposite the desired main stem bronchus. In this case, the lung opposite the side of the connector clamp will collapse. Inadequate separation, increased airway pressures, and instability of the DLT usually occur. In addition, because of the morphology of the DLT curvatures, tracheal or bronchial lacerations may result. If a left-sided DLT is inserted into the right main stem bronchus, it obstructs ventilation to the right upper lobe. It is therefore essential to recognize and correct such a malposition as soon as possible.
Second, the DLT may be passed too far down into either the right or the left main stem bronchus (Fig. 37-13). In this case, breath sounds are very diminished or not audible over the contralateral side. This situation is corrected when the tube is withdrawn and the opening of the tracheal lumen is above the carina.
Third, the DLT may not be inserted far enough, leaving the bronchial lumen opening above the carina. In this position, good breath sounds are heard bilaterally when ventilating through the bronchial lumen. No breath sounds are audible when ventilating through the tracheal lumen because the inflated bronchial cuff obstructs gas flow arising from the tracheal lumen. The cuff should be deflated and the DLT rotated and advanced into the desired main stem bronchus.
Fourth, a right-sided DLT may occlude the right upper lobe orifice. The mean distance from the carina to the right upper lobe orifice is 2.3 ± 0.7 cm in men and 2.1 ± 0.7 cm in women.49 With right-sided DLTs, the ventilatory slot in the side of the bronchial catheter must overlie the right upper lobe orifice to permit ventilation of this lobe. However, the margin of safety is extremely small, and varies from 1 to 8 mm.66 It is therefore difficult to ensure proper ventilation to the right upper lobe and avoid dislocation of the DLT during surgical manipulation. When right endobronchial intubation is required, a disposable right-sided DLT is perhaps the best choice because of the slanted doughnut shape of the bronchial cuff, which allows the ventilation slot to ride off the right upper lobe ventilation orifice and increases the margin of safety.
Fifth, the left upper lobe orifice may be obstructed by a left-sided DLT. Traditionally, it was believed that the take-off of the left upper lobe bronchus was at a safe distance from the carina and that it would not be obstructed by a left-sided DLT. However, the mean distance between the left upper lobe orifice and the carina is 5.4 ± 0.7 cm in men and 5 ± 0.7 cm in women.67 The average distance between the openings of the right and left lumens on the left-sided disposable tubes is 6.9 cm. Therefore, an obstruction of the left upper lobe bronchus is possible while the tracheal lumen is still above the carina. There is also a 20% variation in the location of the blue endobronchial cuff on the disposable tubes because this cuff is attached to the tube at the end of the manufacturing process.
Bronchial cuff herniation may occur and obstruct the bronchial lumen if excessive volumes are used to inflate the cuff. The bronchial cuff has also been known to herniate over the tracheal
carina, and in the case of a left-sided DLT, to obstruct ventilation to the right main stem bronchus.
carina, and in the case of a left-sided DLT, to obstruct ventilation to the right main stem bronchus.
Another rare complication with DLTs is tracheal laceration or rupture (Fig. 37-14). Overinflation of the bronchial cuff, inappropriate positioning, and trauma owing to intraoperative dislocation that resulted in bronchial rupture have been described in association with the Robertshaw tube and the disposable DLT.68 Therefore, the pressure in the bronchial cuff should be assessed and decreased if the cuff is found to be overinflated. If absolute separation of the lungs is not needed, the bronchial cuff should be deflated and then reinflated slowly to avoid excessive pressure on the bronchial walls. The bronchial cuff should also be deflated during any repositioning of the patient unless lung separation is absolutely required during this time.
In a recent prospective trial, 60 patients were randomly assigned to two groups. OLV was achieved with either an endobronchial blocker (blocker group) or a DLT (double-lumen group). Postoperative hoarseness and sore throat were assessed at 24, 48, and 72 hours after surgery. Bronchial injuries and vocal cord lesions were examined by bronchoscopy immediately after surgery. Postoperative hoarseness occurred significantly more frequently in the double-lumen group compared with the blocker group (44% vs. 17%, respectively). Similar findings were observed for vocal cord lesions (44% vs. 17%). The incidence of bronchial injuries was comparable between groups.69
Lung Separation in the Patient with a Tracheostomy
Occasionally, a patient with a permanent tracheostomy is scheduled for surgery on the lung that requires isolation. Examples of such patients include those who have undergone resection of a tumor in the floor of the mouth or on the base of the tongue, followed by extensive reconstructive surgery with the creation of a permanent tracheal stoma. Routine follow-up may reveal a lung lesion that requires a diagnostic procedure. Conventional double-lumen endobronchial tubes are designed to be inserted through the mouth, not through a tracheal stoma. The standard DLTs are usually too stiff to negotiate the curve required for insertion through a tracheal stoma and are difficult to position.70 A separately inserted bronchial blocker may permit adequate lung separation.71
Saito et al.72 described a spiral, wire-reinforced, double-lumen endobronchial tube made of silicone (Koken Medical, Tokyo, Japan) that is designed for placement through a tracheostomy. The middle section of the tube consists of two thin-walled silicone catheters with an internal diameter of 5 mm, glued together and reinforced with a stainless steel spiral wire and covered with a silicone coating with two pilot balloons. The distal section, which contains the bronchial lumen and the bronchial cuff, is made of wire-reinforced silicone to avoid excessive flexibility. The dimensions are based on the Mallinckrodt DLT (Hazelwood, MD). The bronchial cuff is located 1.2 cm from the tip, and the distance between the tip orifice and the tracheal orifice is 4.9 cm. In a clinical trial in patients with permanent tracheal stomas, the tubes functioned well in achieving lung separation, with no sign of kinking or movement, and permitted easy passage of a suction catheter.
Lung Separation in the Patient with a Difficult Airway
An airway may be recognized initially as difficult when conventional laryngoscopy reveals a grade III or IV view (see Chapter 27). When separation of the lungs is required and the patient has a clearly recognized difficult airway, then awake intubation using a flexible fiberoptic bronchoscope can be used to place a DLT, Univent tube, or single-lumen tube (SLT). The SLT may then be exchanged for a DLT or Univent tube using a tube exchanger. Furthermore, depending on the extent and duration of the surgical procedure, and the amount of fluid shift, an airway that was not initially classified as difficult may become difficult secondary to facial edema, secretions, and laryngeal trauma from the initial intubation.73,74
A logical approach to lung separation is shown in Figure 37-15. When lung separation is mandated and the patient has a recognized difficult airway, awake intubation using flexible fiberoptic bronchoscopy can be attempted using a DLT, Univent tube, or SLT. The same approach may be used for the patient with an unrecognized difficult airway and a failure to intubate with conventional laryngoscopy. When using a DLT over a fiberoptic bronchoscope, the anesthesiologist should keep in mind that it is a bulky tube with a large external diameter and because of the length of the DLT, only a limited part of the fiberoptic bronchoscope is available for manipulation. In addition, the mismatch between the flexibility of the fiberoptic bronchoscope and the rigidity of the DLT makes it more difficult to advance over the fiberoptic bronchoscope. The Univent tube has the same bulky external diameter and is also often difficult to pass between the vocal cords, particularly in a patient who is awake.
Single-lumen Tube can be Successfully Placed
If a failure to provide lung separation could result in a life-threatening situation, there are two possibilities to provide OLV when an SLT is already in place. First, depending on the indication for lung isolation, a tube exchanger can be used to switch to a DLT or a Univent tube. The second possibility is to direct a
bronchial blocker through the SLT into the selected main stem bronchus. These two methods, however, offer limited protection or an inadequate seal in cases such as lung lavage, pulmonary abscess, or hemoptysis, where a DLT would be the tube of choice.
bronchial blocker through the SLT into the selected main stem bronchus. These two methods, however, offer limited protection or an inadequate seal in cases such as lung lavage, pulmonary abscess, or hemoptysis, where a DLT would be the tube of choice.
Use of a Tube Exchanger
Several tube exchangers are commercially available (Cook Critical Care, Bloomington, IN; Sheridan Catheter Corporation, Argyle, NY). On these tube exchangers, the depth is marked in centimeters; they are available in a wide range of external diameters and easily adapted for either oxygen insufflation or jet ventilation. The size of the tube exchanger and the size of the tube to be inserted should be tested before use in a patient. The 11-Fr tube changer will pass through a 35- to 41-Fr DLT, whereas the 14-Fr tube exchanger does not pass through a 35 Fr. To prevent lung laceration, the tube exchanger should never be inserted against resistance. Because the first generation of tube exchangers was very stiff, there was a risk for tracheal or bronchial laceration. Recently, a new tube exchanger with a soft flexible tip was released by Cook Critical Care that is safer to use and is less likely to cause airway laceration. Finally, when passing any tube over an airway guide, a laryngoscope should be used to facilitate passage of the tube over the airway guide past supraglottic tissues.
Use of Modern Bronchial Blockers

Indications for the use of a bronchial blocker are shown in Table 37-2. An independently passed bronchial blocker may be used with an SLT to obtain lung isolation, thereby avoiding the use of a DLT in a patient with a difficult airway. The use of a bronchial blocker also eliminates the potential risk of needing to change a DLT to an SLT at the conclusion of the procedure. The blockers are discussed later, in the chronologic order in which they were developed, and came into practice. In the past, Fogarty vascular embolectomy catheters were used for lung separation, but there is no indication for their use in the current practice of thoracic anesthesia. The balloon of the Fogarty is high pressure, low volume, and there is no lumen to allow egress of gas from the lung to facilitate deflation.

mediastinoscopy followed by thoracotomy), or in cases of bilateral lung transplantation. The Univent tube has the advantage common to all bronchial blockers: It is an SLT, and there is no need to change the tube at the end of the procedure if postoperative ventilatory support is required. This is particularly important in cases of difficult intubation, prolonged surgery with airway edema, such as thoracic aortic aneurysm surgery or extensive neurosurgical procedures on the spine with massive fluid replacement, and altered anatomy of the airway. It is also possible to suction through the blocker lumen or to apply continuous positive airway pressure (CPAP) to improve oxygenation in cases of hypoxemia.

Full access? Get Clinical Tree
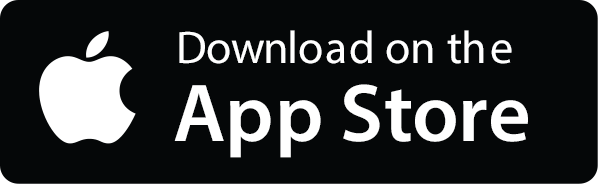
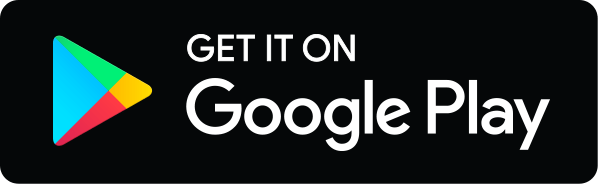
