Posteriorly, each vertebra has a spinous process attached to bilateral laminae to form an arch. The laminae connect to bilateral transverse processes and then the pedicles, until finally fusing with the anterior vertebral body. This forms a central opening, the vertebral foramen or spinal canal, wherein the spinal cord resides. Between the spinous and transverse processes are two sets of paired superior and inferior facets, or articulating processes, that allow vertical, forward, backward, and twisting motion and assist with anterior vertebral column stabilization.
Intervertebral disks (IVDs) act as resilient, vertical cushions between vertebral bodies. Disks are composed of circumferential fibrocartilaginous material, the annulus fibrosus, and a central gelatinous material, the nucleus pulposus.
The atlas or C1 is a ring-like formation with no spinous process, vertebral body, or inferiorly located IVD. Its superior facets articulate with a connection of alar ligaments to the occipital skull condyles bilaterally. It forms a unique joint able to swivel up to 50% around the cone-like dens or odontoid process protruding from the superior aspect of C2, the axis. The spinal cord passes through the canal next to this process as an extension from the brainstem through the foramen magnum of the skull.
The transverse atlantal ligament attaches between the medial tubercles of the superior facets bilaterally, crossing the vertebral column to provide odontoid stability anteriorly and free spinal cord motion posteriorly. Atlantoaxial instability (AAI) can develop from transverse atlantal ligament laxity, odontoid hypoplasia, or other boney abnormality. Transverse ligament laxity can drive the dens into the spinal cord as C1 subluxates anteriorly. On the other hand, if the dens is hypoplastic, C1 can subluxate posteriorly. AAI is associated with a number of syndromes and pathologic states such as trauma, oropharyngeal infection, Pierre Robin syndrome, Ehlers–Danlos syndrome, Marfan’s syndrome, hypermobility syndrome, nonachondroplastic dwarfism, and Morquio syndrome, but its greatest incidence is in patients with Down’s syndrome and rheumatoid arthritis (RA) [2]. Although 10% to 20% of patients with Down’s syndrome have AAI, only 1% to 2% are symptomatic, making it vital that airway management specialists be alert to this possibility. Degenerative changes may also develop secondary to fibrocartilage within the ligament, as found in RA patients, who have a 12% to 25% incidence of AAI.
Normal spinal curvatures include an anterior cervical convexity between the odontoid apex and mid-T2 vertebra, posterior thoracic convexity from mid-T2–T12, and an anteriorly directed lumbar convexity from mid-T12 until the sacral bone. Multiple ligaments maintain the S-shaped spinal integrity by preventing excessive motion. The anterior longitudinal ligament traverses the length of the spinal columns from the axis, along the anterior surface contiguously with the vertebrae and IVDs. Meanwhile, the posterior longitudinal ligament courses down the posterior surface of the vertebral bodies within the spinal canal. Posteriorly within the spinal canal, the ligamentum flavum lies opposite to the posterior longitudinal ligament, while connecting vertebral laminae. Lastly, the interspinous ligament joins the ligamentum flavum anteriorly. Below C7, it is joined by the supraspinous ligament, which connects the spinous processes.
Ligamentous disruption, or laxity, has a greater propensity for cervical instability, in contrast to C1 or C2 fracture with intact ligaments. Instability in the former situation demands surgical repair, while the latter pathologic state usually heals spontaneously. Magnetic resonance imaging (MRI) best detects ligamentous instability. In these instances, particularly with spinal cord injury (SCI), airway management specialists must take precautions to prevent excessive cervical motion and secondary neurologic damage.
2. Spinal cord and nervous tissue. The spinal cord extends downward from the medulla oblongata toward the L1–L2 vertebrae in adults, where it forms the conus medullaris [1]. Nearby, its nerve roots combine to form the cauda equina, and it finishes in the filum terminale between S2/S3 and the coccyx. It is surrounded by the meninges and spinal fluid. Excepting C1 and C2, 31 pairs of segmental nerves take off through the intervertebral foramina. Eight are cervical, while the three coccygeal elements form a single pair that exits through the sacral hiatus.
Examination of a transverse plane through the spinal cord reveals peripheral sensory and motor neurons in the white matter surrounding a central butterfly-shaped area of nerve cell bodies in the gray matter. The spinal cord is surrounded by the very thin pia mater with dentate ligaments serving to secure it. Cerebrospinal fluid (CSF) freely communicates between the cerebral ventricular system and the most caudal regions of the spinal subarachnoid space, between the pia mater and the arachnoid membrane. External to this lies the subdural space occupying an area between the arachnoid membrane and the dura mater. The dura is an extension from the brain that narrows near S2 and ends in the filum terminale.
The autonomic nervous system consists of complementary functioning sympathetic, parasympathetic, and nonadrenergic/noncholinergic-mediated divisions [3]. The sympathetic division encompasses preganglionic synapsing neurons between T1–L2, with cell bodies in the lateral gray horns on both sides of the spinal cord. The parasympathetic division consists of preganglionic synapsing neurons, with cell bodies in the nuclei of the brainstem (cranial nerves III, VII, IX, and X) and the lateral gray horns of S2–S4.
3. Blood supply to the spinal cord
a. Arterial supply. Similar to the cerebral circulation, spinal circulation supplies its gray matter with 3 to 4 times more blood flow than its white matter. Two vertebral artery branches originate from the aorta. Next, near the base of their confluence and before the formation of the basilar artery, a single anterior spinal artery is formed [1]. Constituting 75% of spinal circulation, this artery exits at the level of the foramen magnum to accompany the spinal cord while supplying the anterior surface and most of the lateral areas down to the level of the filum terminale.
Two posterior spinal arteries also arise to complete the supply, mainly serving the posterior spinal columns and horns. Considerable branching and plexus formations of arterioles and capillaries from these vessels develop in the cervical, thoracic, and lumbar regions. They anastomose with intercostal and lumbar arteries comprised of 4 to 10 radicular branches, thus permitting additional arteriolar sources of blood. Frequently, at the level of T8–T11, a left branch from the aorta supplies an important vessel, which may be the main source for the upper lumbar regions and often the lower thoracic spine, called the arteria radicularis magna or artery of Adamkiewicz [4]. Serious damage to the spinal cord may occur as a result of significant compromise of this artery from trauma, abdominal/thoracic aortic surgery, and so forth.
During spinal surgery, awareness of this blood supply has prompted anesthesia providers to avoid SCI by maintaining spinal cord perfusion pressure >60–70 mm Hg. SCI patients undergoing spinal surgeries require maintenance of perfusion pressures at or significantly above normal, depending on the amount of pre-existing neurologic damage. Of course, the possibility of greater blood loss is an ongoing concern. Maintaining perfusion can be accomplished by standard hemodynamic methods and for some surgeries, assisted with the use of a spinal drain to maintain CSF pressure <10 mm Hg. Occasionally attempts for draining ≤10 to 15 mL/h are undertaken to increase the arterial/spinal fluid gradient. Pre-existing SCI is very susceptible to vascular insufficiency. The use of mild hypothermia and mannitol may assist by decreasing metabolic demand and tissue edema, respectively.
b. Venous drainage. Venous outflow from the spinal cord is accomplished by up to six anterior and posterior superficial vessels draining into plexes or veins entering the internal epidural venous system [1]. From there, blood can escape cephalad into the basivertebral vein, terminal veins, or dural sinuses. Further drainage takes place via vertebral veins and inferior vena cava conduits. The valveless nature of this system makes it prone to reverse flow characteristics following elevations in intrathoracic or intra-abdominal pressure, due to coughing, pregnancy, Valsalva maneuvers, or obesity. This can result in symptoms such as headache, but even worse, the entrance of systemically carried neoplastic or infective cells into the central nervous system (CNS).
For spine surgery, anesthesia providers can employ techniques to avert conditions that promote higher venous pressure. Diligent attention to preventing excessive fluid load and minimizing intra-abdominal pressure is particularly important while prone. This optimizes visualization in the surgical field and diminishes blood loss.
B. Functional anatomy
1. Motor system. Cerebral cortical signals and brainstem motor nuclei impulses travel along motor neuron pathways [5] (Fig. 12.1). In the spinal cord, these are primarily divided among (1) pyramidal tracts (upper motor neurons in the lateral and anterior corticospinal tracts) and (2) extrapyramidal tracts (vestibulospinal, reticulospinal, olivospinal, and rubrospinal). In the anterior medulla, 90% of spinal motor neurons cross to the contralateral side at the decussation of the pyramids.
2. Sensory system. The sensory system has three major pathways. The dorsal column medial lemniscus transports signals for fine touch, conscious proprioception, and vibration. It decussates in the medulla on the way to the cortex (Fig. 12.1). The spinocerebellar tracts transmit signals for proprioception and terminate in the ipsilateral cerebellum. Lastly, the anterolateral system, within the anterior and lateral spinothalamic tracts, relays impulses for pain, temperature, and gross touch, ascends one or two levels, synapses, and decussates in the substantia gelatinosa on the way to the brain.
3. Spinal cord autoregulation. Research on human spinal cord blood flow (SCBF) is not as extensive in comparison to cerebral blood flow investigations [6]. Nevertheless, SCBF in multiple animal studies (rats, dogs, cats, and primates) have shown consistent homeostatic flow of approximately 55 to 60 mL/100g/min. Autoregulatory control is similar to the brain, in response to alterations in mean arterial pressure (MAP) between 40–70 and 120 mm Hg, arterial partial pressure of carbon dioxide (PaCO2) between 20 and 80 mm Hg, and arterial partial pressure of oxygen (PaO2) under 60 mm Hg. The concept of spinal cord autoregulation motivates caregivers to consider maneuvers or drugs applied to SCI patients in a mode parallel to that of brain injury cases.
2
II. Preoperative assessment of spinal cord injury. In urgent situations, routine history and physical examinations need to be hastened and perhaps abbreviated. The most important points to elicit include states of consciousness before and during examination, circumstances of SCI, neurologic signs and symptoms, severity of other injuries or illnesses (particularly those that might mask SCI complaints), and laboratory, spinal, and/or other radiographic results.
FIGURE 12.1 (Right) Motor and (Left) sensory branching and crossovers in the spine.
A. History and physical examinations. Whenever feasible, documentation is needed concerning prior anesthetic, surgical, and familial anesthetic history, airway history, allergies, medications, social history of tobacco, alcohol, or illicit drug consumption, recent oral intake, height, weight, airway and physical examination, neurologic assessment, and appropriate studies.
B. Neurologic assessment and clinical consequences of SCI. The American Spinal Injury Association (ASIA) dermatome chart is used for recording the extent and site of neurologic injury [7]. A 28-dermatome sensory section is used for recording “pinprick and light touch stimuli” sensory levels. Three scores are available: “0” (no sensation), “1” (impaired sensation), and “2” (normal sensation). A 0- to 5-point motor scale allows recording of specific muscle groups for strength, where “0” represents complete paralysis, and “5” is normal. It also permits documentation of proprioception, external anal sphincter tone/contraction, perirectal sensation, bulbocavernosus reflex, and anal-cutaneous reflex.
FIGURE 12.2 Surgery in a patient with severe ankylosing spondylitis: Nasal local anesthesia in a patient who uses a mirror during conversation.
Symptoms of SCI are frequently characterized by complete or incomplete losses of neurologic function below the point of damage in any of the three main nervous system tracts—motor, sensory, and autonomic. While motor injury involves a degree of paralysis, and somatosensory injury causes losses in touch, pain, temperature, vibration, and/or proprioception, autonomic deficits are evident by alterations in hemodynamic, respiratory, gastrointestinal, urinary, piloerective, and/or sexual function. The ASIA Impairment Scale is a classification meant to differentiate a patient’s SCI into one of the five categories (Table 12.1).
Table 12.1 ASIA Impairment scale
As discussed, complete SCI is indicative of <5% chance for recovery if no improvement transpires within 24 hours. An incomplete lesion is defined by ASIA when there is at least sacral or segmental sparing, even if only to pinprick, and has up to a 50% probability of functional return [7,8].
1. Spinal shock. Spinal shock includes sensory and motor neurologic reflex changes from sudden traumatic SCI or infrequently, injury developing over several hours. Simultaneously, neurogenic shock or hemodynamic changes caused by autonomic dysfunction may be present [8]. A review by Ditunno et al. [9] depicts four time frames correlating with presenting signs and symptoms of ongoing pathophysiologic changes.
Phase 1 of spinal shock is usually immediate, developing within 24 hours. Reflex loss below or possibly immediately above the level of SCI results in flaccid paralysis, arreflexia, and loss of descending facilitation due to hyperpolarized neurons. Hypotension results from muscular atony and loss of vascular tone below the SCI.
Over the next 1 to 3 days, the second phase involves returning reflexes, denervation supersensitivity, after upregulation of receptors within muscle groups. Consequently, polysynaptic reflexes reappear before the return of more rapid monosynaptic reflexes, such as deep tendon reflexes or aversion responses.
Within 1 to 4 weeks and lasting up to 6 months, the third phase is associated with hyperreflexia as interneurons and lower motor neuron axonal-supported synapses proliferate or “sprout” and the tempering effects from higher centers is absent.
During the fourth phase, over a period of 1 week to 12 months, an imbalance occurs between the alpha and gamma motor neurons with alpha neurons sending fewer or alternatively, more signals, resulting in either a weak or excessive contracture.
For anesthesia and other caregivers, post-SCI spasm is treated with baclofen, benzodiazepines, or other medications. Note that other influences such as hyperventilation can alter the motor neuronal receptors’ co-dependent action, but SCI spasms are unlikely to be relieved by hypoventilation or breathing CO2.
2. Neurogenic shock. Neurogenic shock results in a constellation of hemodynamic consequences from disrupted sympathetic pathways [8]. SCI above T6 precipitates decreased vasomotor input, systemic vascular resistance, and cardiac output resulting in hypotension and hypothermia. Injury including T1 will block cardiac accelerators (T1–T4), causing bradycardia due to unopposed parasympathetic activity.
Thus, it is important to be aware that SCI patients may be in spinal shock, neurogenic shock, and/or hypovolemic shock, in order to differentiate and administer appropriate treatments. Hypovolemic shock is usually characterized by hypotension, tachycardia, and a narrowed pulse pressure, requiring fluid administration therapy. On the other hand, pure fluid administration for spinal or neurogenic shock may result in symptomatic fluid overload. Bilello et al. [10] noted that vasopressors and chronotropic agents were required in 24% of high (C1–C5) versus 5% of low (C6–C7) cervical SCI patients. Of the high cervical injury group, 3% required a pacemaker.
3
3. Autonomic dysreflexia or hyperreflexia. Autonomic dysreflexia can occur in patients with an SCI T6–T7 or higher [11]. Following a noxious stimulus below that level (often of hollow viscous organs), the thoracolumbar sympathetic nerves transmit a strong afferent impulse to the spinal cord with the development of vasoconstriction below the SCI level and systemic hypertension. Normally, hypertensive carotid sinus activation (vasovagal) ceases sympathetic firing by sending inhibitory signals down the spinal cord. But, because of the SCI, inhibition does not reach the lower sympathetic outflow segments. Inhibition above the SCI level provokes reflex bradycardia, vasodilation, headache, and profuse sweating. Frequently, this rostral bradycardia and vasodilation are inadequate to overcome the severe, precipitous rise in blood pressure (BP), which may cause seizures, stroke, myocardial infarction, neurogenic edema, and death.
In anticipation of noxious stimuli below the level of SCI, even a full bladder during surgery, anesthesia providers must try to elicit a history of autonomic dysreflexia, sometimes using descriptive lay terminology. Spinal or epidural anesthetics completely block this reflex. General or monitored care anesthetics are often less effective because of the hit-or-miss nature of drug dosages. Very frequent BP monitoring while having immediate availability of rapid-onset, vasodilating agents such as sodium nitroprusside, is strongly advised with these techniques.
3
4. Cervicomedullary syndrome. The cervicomedullary syndrome is very similar to the central cord syndrome with the caveat that it is characterized by loss of facial sensation, starting from the mouth to the nose and extending outwardly to the chin, eyes, and forehead in a Dejerine or “onion peel pattern” [12,13]. Pathologic changes involve the nucleus or fibers in the descending spinal tracts of the trigeminal nerve. Upper extremity sensory loss and weakness is typically greater than that in the lower extremity, in a similar fashion to the anterior cord syndrome.
Inciting factors frequently include disk pathology or spondylosis in the cervicomedullary region down to C4. This may be worsened by cervical hyperextension or trauma. Compression due to atlas–axis dislocation, excessive traction, odontoid fracture, or anterior–posterior complications from a burst fracture or atlantal ligament disruption may be involved. Vascular injury to the vertebral artery is also a possibility.
These patients need close monitoring to detect hemodynamic or respiratory compromise, and anesthesia or other caregivers must be prepared to institute immediate management.
5. Central cord syndrome. Hyperextensive compression mechanisms involving the ligamentum flavum, syringomyelia, and tumors causing violation of the integrity of the mid to lower cervical spine can result in the central cord syndrome. Symptoms are typical for more significant upper extremity loss of motor function, with variable sensory deficit due to centrally crossing, corticospinal tracts carrying pain, fine touch, temperature, and proprioception information [13]. Often patients have a good recovery even without surgery, but hemodynamic and respiratory monitoring is important.
6. Anterior cord syndrome. The anterior cord syndrome is usually brought on by flexion injuries, disk pathology, or anterior spinal artery disruption [13]. Damaged corticospinal and/or spinothalamic tracts result in deficits in motor, pain, and temperature conduction below the SCI. Sacral sparing and autonomic dysfunction can occur only if cervical lesions are involved. Prognosis for complete recovery has been reported to be as high as 50%.
Anesthesia and other caregivers particularly monitor these patients for hemodynamic and respiratory functions if cervical injury is suspected.
7. Posterior cord syndrome. Vascular compromise of the posterior spinal arteries may be responsible for the rare occurrence of the posterior cord syndrome. Patients lose ipsilateral fine touch, vibration, and proprioception sense, resulting in ataxia and a faltering gait [13].
8. Brown-Séquard syndrome. This syndrome is a rarity due to a hemisection type of SCI from tumor, penetrating wound, vascular obstruction, infection, myelitis, multiple sclerosis, or trauma [13]. Injury to the dorsal medial lemniscus, decussating at the medulla, results in ipsilateral motor, fine touch, vibration, and proprioception losses. Uniquely, damage to contralateral corticospinal tracts and spinothalamic tracts, decussating in the substantia gelatinosa, results in pain, coarse touch, and temperature sensation losses on the opposite side of the body.
9. Conus medullaris syndrome. The conus medullaris syndrome can be incurred following injury at T12–L1 from intrinsic cord compression. Consequences include perianal analgesia, bladder and bowel areflexia, impotence, and disordered ankle reflex and lower limb motor function. Knee reflexes are intact because the cauda equina is unaffected [12].
10. Cauda equina syndrome. Lastly, the cauda equina syndrome often develops from spinal, disk, ligament, or tumor pathology at L1–L5 [13]. Although appearing similar to peripheral nerves, the proximity of these nerve roots to their spinal cord motor neurons where axonal growth inhibitors reside, may prevent axon regrowth, causing permanent damage. Patients exhibit signs of bladder dysfunction, sensory and motor dysfunctions involving L4–S3 with asymmetric paraplegia, anal dysfunction, sciatica, unilateral or bilateral saddle anesthesia, pubic anesthesia, and lower extremity numbness.
C. Neurologic effects on other systems
1. Respiratory system. Compete SCI at C3 or higher is a leading cause of prehospital deaths from total diaphragmatic paralysis. Lesions below C5 provoke paradoxical breathing with 33% to 50% of normal vital capacity, due to decreased functional residual capacity as well as maximal inspiratory and particularly, expiratory volumes [14]. Any SCI above T6 with loss of abdominal muscle strength may result in difficulty with coughing, atelectasis, pneumonia (≤50% incidence, particularly the left lung), and respiratory failure. Recovery is only weakly predicted by the lesion level, whereas spontaneous recovery and motivation to improve recovery are important factors.
Since SCI may lead to acute respiratory failure, hypoxemia, or hypercarbia, close monitoring is essential. Critical levels of PaO2 ≤ 50 mm Hg, PaCO2 ≥ 50 mm Hg, or vital capacity <10 mL/kg are indications for proactive support by caregivers, assuming no pre-existing chronic changes due to obstructive pulmonary or other disease.
2. Gastrointestinal system. Paralytic ileus is more likely in tetraplegics than paraplegics. Pulmonary aspiration is more likely, particularly after heightened vomiting episodes, hypokalemia, and hypovolemia. Abdominal distension can impede adequate inspiration. Constipation and pseudo-obstruction may occur. In addition, unopposed vagal efferents may lead the way to the development of gastric stress ulcers and bleeding.
3. Genitourinary system. Two types of bladder dysfunction can result from SCI. A spastic or reflex bladder may develop from injury above T12. When the bladder fills, it reflexively empties without the patient’s awareness. Bladder contraction with an unrelaxed sphincter raises pressure, making ureteral reflux likely, and increases the possibility of bladder infection spread to the kidneys. In contrast, a flaccid bladder and overdistension problems may develop from SCI below T12/L1. Both problems have attendant requirements for frequent catheterization, danger of infection, and potential for kidney dysfunction, damage, failure, and hypertension.
4. Thermoregulatory system. With high SCI, sympathectomy, vasodilation, and lack of piloerection may result in a partial poikilothermic-type situation, resulting in significant temperature loss in cold environments. Whether conversely, an inability to perspire below the level of damage may prevent cooling in hot environments is somewhat controversial. It may be that at higher environmental temperatures the thermoregulatory set point is increased, while the opposite may result at lower environmental temperature conditions.
5. Thrombogenesis. Historically, vasodilation and immobility with venous pooling, low blood flow, and increased blood viscosity were associated with increased risk of deep vein thrombosis (DVT) in SCI patients, particularly 3 days post injury. The result was a DVT rate of 48% to 100% and chances of pulmonary embolism were 8% to 25%. With the advent of prophylactic anticoagulants, patients treated with low molecular weight heparin (LMWH) and a targeted international normalized ratio (INR) of 2 to 3 resulted in reductions in DVT rates to 5.4%.
Some SCI patients presenting for surgery have a history of, or need for, anticoagulant therapy. Discontinuation of warfarin 5 days prior to surgery is a common goal. For urgent surgeries where blood loss is unlikely, the Australian Society of Thrombosis and Hemostasis consensus guidelines recommended reversal with injectable vitamin K to achieve an acceptable INR above the subtherapeutic range so that postoperative return to warfarin therapy may be seamless [15]. If bleeding is more probable the most desirable INR value should approach normal. Since fresh frozen plasma (FFP) has an INR of 1.6, it cannot be the sole treatment. FFP, recombinant factor VIIa, and prothrombin complex concentrates are alternative “anti-warfarin effect” therapies.
For patients receiving heparin, careful protamine titration can easily reverse its effect. At this time LMWH reversal is uncertain. Protamine binds preferentially to larger weight molecular heparin but not as well to LMWH, with the result that reversal success rate approaches only 85%.
Direct thrombin inhibitors (DTI) used for DVT prophylaxis are also difficult to reverse. Attempts with plasma complex concentrates and recombinant factor VIIa have had varying success depending on the DTI and dosages.
D. Preoperative testing. Radiologic testing, evoked potential (EP) analysis, electromyography (EMG), ultrasonography, urologic incontinence studies, and spinal fluid analysis can demonstrate sites, types, and degrees of SCI to formulate therapeutic courses and prevent deterioration.
1. Radiologic studies of the spine
a. Diagnostic radiologic studies
(1) Plain radiographs. In trauma cases, the triple view series visualizing all seven cervical vertebrae to the C7–T1 junction with a “swimmer’s view” is important if CT or MRI is unavailable. Particularly lateral images can reveal fractures of the odontoid, vertebral bodies, and spinous processes. Anterior–posterior and oblique images are best to locate pedicle, lamina, or facet damage and lateral mass vertebral body detection, especially at C1. Flexion/extension films can be judiciously used to assist in determinations of ligamentous injury by looking for increased dens and C1 arch distance, abnormally spread apart posterior elements or kyphosis in flexion, or vertebral subluxation. Care must be taken not to worsen an unstable spine with flexion/extension movements, which should only be allowed in conscious and cooperative patients so that they can vocalize discomfort if pain occurs. Plain radiographs elsewhere along the spinal column can also be used to detect boney changes, including prevertebral soft tissue swelling.
Plain x-ray criteria for lower cervical spine instability include the presence of greater than 11 degrees and 3.5 mm of sagittal plane translation. Interspinous widening, absence of the cervical lordotic curve or facet joint correspondence, and vertebral body compression greater than 50% are diagnostic of spinal pathology.
(2) Computed tomography. Although clinical judgment to predict diagnostic SCI may be inadequate, plain x-ray studies may be woefully worse [16]. Among symptomatic SCI patients, the correct diagnosis after plain radiographic study may be missed in ∼5% of patients and 30% of those may develop permanent sequelae. In contrast, SCI is identified in 95% to 100% of patients by cervical spine computed tomography (CT scan) in comparison to less than 50% by cervical spine plain radiographs. Thus, many investigators and clinicians view CT scans as the definitive study for acute SCI suspicions for boney structure and spinal canal diameter to detect impingement. Neuroforaminal distances and facet joints can be examined for ligamentous instability. Intrathecal contrast can help somewhat to visualize neural elements.
(3) Magnetic resonance imaging. MRI is superior for soft tissue visualization, including the spinal cord, myelomalacia, nerve roots, cauda equina, epidural and subarachnoid spaces, hematoma, edema, syringomyelia, IVDs, ligaments, and compression in any of these areas. SCI without radiographic abnormality (SCIWORA) occurring particularly in children and the elderly is diagnosed by MRI only. Although not as sensitive in detection as CT, MRI can demonstrate boney changes, including fractures, fragmentation, and osteophytes.
b. Criteria for radiologic studies for acute cervical spine injury. How can clinicians decide on which type of radiologic examination is best? While less accurate, plain x-rays have minimal radiation exposure and are much cheaper than CT or MRI. Clinicians worry about missing critical SCI, particularly in blunt trauma patients known to have risks for SCI [17]. Fear has prompted anywhere from 15.6% to 91.5% of clinicians to order radiographic studies on most, if not all, of their patients. Attempts were made to develop clinical criteria to determine the following: Which patients would benefit from radiologic studies, such that no clinically important SCI would be missed and fewer patients would receive needless irradiation or incur excessive expenditures?
Two sets of criteria were developed to decrease the inappropriateness of radiologic testing for alert patients: The National Emergency X-Radiography Utilization Study (NEXUS), Low-Risk Criteria (NLC) study, and the Canadian C-Spine Rule study. Their salient points are summarized (see Table 12.2). In general a Glasgow Coma Score <15, acute paralysis, history of spinal disease/surgery, vital sign instability, and at-risk suspicion in minors (depending on the mechanism) indicate a need for computed axial tomography (CT) or magnetic resonance imaging (MRI). Indicators for radiologic study in awake, mobile patients include injury associated with a dangerous mechanism, extremity paresthesias, age ≥65 years, or inability to rotate the neck 45 degrees to the left or right beyond the acute phase of neck pain.
Table 12.2 Alert-patient criteria to determine if radiography is needed to rule out cervical SCI (Glasgow Coma Scale score ≥ 14–15)
4
The comparative values of the two sets of criteria showed that the sensitivity (99.4% vs. 90.7%) and specificity (45.1% vs. 36.8%) were significantly higher when using the Canadian C-Spine Rule versus the NEXUS criteria, respectively [17]. Note that acute paralysis, age of minors, changes in consciousness, vital sign instability, and/or history of spinal disease or surgery are also likely to make patients candidates for radiographic studies. In 45 clinically unimportant cases, the sensitivity was 97.8% using the Canadian C-Spine Rule versus 80% with the NEXUS criteria.
4
2. Additional diagnostic techniques.
a. Cortical somatosensory evoked potentials. Cortical somatosensory evoked potentials (SSEPs) generated by electrical square wave stimulation of peripheral nerves can identify the location and extent of sensory radiculopathy or other pathology [18]. Recording electrodes, located proximally along the spine and/or on the scalp in a similar pattern to the international EEG 10–20 system, detect recordable computer-averaged waveforms with specific amplitudes and poststimulus latencies. Even though only sensory tract EPs are monitored, mixed nerves (median, posterior tibial, and so forth) are chosen for stimulation with surface electrodes or needles, so that a visible twitch gives assurance that the nerve is in fact being targeted correctly. In the event of SCI, EP waveforms demonstrate decreased polarity amplitudes and delayed latencies (assuming intact peripheral nerves) (see Chapter 26).
Successful preoperative use of intraoperative neurophysiologic monitoring techniques (IOM) has translated to the surgical suite with a presence in approximately 75% of major spinal surgeries and an incidence of SSEP abnormalities approaching 2% to 65%. Preoperatively, SSEPs are also useful in uncooperative patients unable to undergo CT or MRI or for patients needing better detection of the extent of damage.
If preoperative SSEPs show extensive pre-existing SCI, anesthesia providers may change plans to include total intravenous anesthesia (TIVA) instead of inhalation anesthetics to avoid injury-mimicking effects on SSEP waveforms.
b. Cortical motor evoked potentials. Transcranial motor evoked potentials (MEPs) can be generated by electrical stimulation while observing for neuroelectrical signals at Recording electrodes in epidural space or muscle responses in peripheral muscles. Magnetic transcortical stimulation is another possibility in awake patients with the advantage of being less painful. Coiled magnets generate ≥5,000 A of current (2,800 V) to produce magnetic field strengths of 2.5 T with a 1-millisecond pulse duration. SCI is more easily detected by cortical MEPs in comparison to SSEPs.
c. Electromyography. Preoperatively, EMG may be valuable to differentiate neurologic damage from peripheral nerve pathology versus SCI and as a diagnostic tool for determining and differentiating the site of functional impairment following SCI.
d. Ultrasonography. Ultrasonography can be utilized if MRI or CT is contraindicated, unavailable, cost-prohibitive, or more difficult to obtain as in the neonatal or infant period. Diagnostic criteria for use include enthesitis in ankylosing spondylitis (AS), syringomyelia, meningomyeloceles, other myeloceles, and congenital malformations such as spina bifida occulta, tight filum terminale, spinal soft tissue mass, dorsal dermal sinus, and misshapen coccyx.
3. Urologic incontinence studies. EMG, cystometrography, postvoid residual urine measurement, and videourodynamic studies are used to determine the likelihood and degree of urinary incontinence impairment secondary to SCI for preoperative testing prior to or during surgeries.
4. Cerebrospinal fluid testing. Detection of increased CSF pressure and abnormalities in chemistries or cell count related to tumor, infection, or syringomyelia may be important in determining potential outcomes.
III. Disorders with potential for spinal cord injury. More common causes for SCI and treatments are presented in two broad groupings: Nontraumatic or traumatic. Nontraumatic entities include noninflammatory disorders, inflammatory disorders, or infectious diseases, and mixed origin demyelinating diseases or upper neuron disorders. Traumatic SCI is further grouped as either cervical SCI or thoracic/lumbar/sacral SCI.
A. Nontraumatic spinal cord disorders
1. Noninflammatory disorders
a. Spondylosis. Cervical spondylosis is a degenerative spinal disease found in 90% of patients ≥65 years of age, but rarely at other ages. No gender inequality exists. Spondylosis results in osteoarthritis of facet joints and vertebral bodies with osteophyte formation, spinal stenosis, foraminal narrowing, associated IVD herniation, and ossification of the longitudinal ligament and ligamentum flavum. Patients may develop radiculopathy due to cervical spine nerve root and/or nerve damage or myelopathy from SCI. Symptoms include suboccipital headache, cervical pain, and radiculopathic and/or myelopathic symptoms.
Cervical spondylosis with myelopathy is a very commonly surgically treated spinal disorder in the elderly, usually by anterior cervical fusion (ACF), anterior cervical diskectomy with fusion (ACDF), posterior decompression and fusion, or cervical laminoplasty. Indications for surgery include failed medical/physical therapy, intractable pain, progressive neurologic deficits, and/or progressive painful or deficit-associated spinal cord or nerve root/nerve compression.
Thoracolumbar spondylosis is usually asymptomatic but can progress to the point of spinal stenosis, instability, and spondylolisthesis (subluxation of one vertebra in relation to another). Lumbar spondylosis, particularly at L3, has a far higher rate of occurrence (80%) and younger age of onset (40 years). There is an association of this spondylosis and osteophytosis with transforming growth factor β1 genotype in some populations.
Anesthesia providers often employ arterial lines for hemodynamic control if specific BP ranges are necessary for perfusion. Vasoactive agents, fluids, colloids, blood products, laboratory testing, and adequate intravascular access with volume or venous air embolism (VAE) monitoring may be required. Airway management may be undertaken with minimal or no neck motion in patients suffering from cervical spine instability or myelopathy, often using awake-fiberoptic intubation (awake-FOI) techniques. Endotracheal tube (ETT) cuff pressures must be minimized for ACF/ACDF during instrumentation to prevent recurrent laryngeal nerve (RLN) injury. Vigilance is required for possible caudad displacement of ETT resulting from neck flexion in sitting, prone, and other abnormal positions.
b. Disk herniation. IVD herniation is more frequent in patients over 40 years of age due to degenerative changes from dehydration and tears or crack formation in the annulus fibrosus; or, it may present at any age from lifting or other trauma. The net result is that the nucleus pulposus extrudes beyond its normal limits causing compression of the spinal cord or nerve roots.
In the cervical region, degenerative disc pathology occurs most frequently at C5–C6, then at C6–C7, and less commonly at C4–C5. Symptomatology often includes cervical pain, radicular dysfunction, and if located centrally, myelopathy.
Rarely in occurrence, thoracic disk herniations usually develop at T8–T12. In contrast, lumbar disk herniation occurs in up to 2% of the overall population. L4–S1 is most often affected, usually posterolaterally, while higher lumbar levels are more involved in older patients. Symptomatology may vary from transient sciatica to radiculopathy, which normally resolves with conservative medical therapy. Significant SCI, such as cauda equina syndrome from central involvement, is indicative for immediate surgical therapy. Other surgical criteria include pain that does not resolve after 6 to 12 weeks or large disk herniation.
Anterior cervical and lumbar diskectomy are preferentially selected surgical techniques. Fusion, hemilaminotomy, and/or laminectomy may also be required.
Anesthesia provider concerns are similar to those for spondylosis surgery, although blood loss and invasive monitoring are less common.
c. Spinal stenosis. Spinal stenosis in any spinal region develops in 0.5% to 1% of the population. Congenital stenosis predisposes to worsening conditions as the body ages. Acquired causes include osteoarthritis, disk degeneration, ligament flavum thickening, neoplasm, trauma, infection, boney inflammatory disease such as AS, and bony metabolic disorders such as Paget’s disease. Most etiologies result in slow central canal or lateral recess stenosis with dysfunction that rarely goes on to major significant SCI. Trauma and other more rapidly evolving etiologies can be much more injurious.
Cervical spine stenosis typically develops from arthritis, AS, or posterior longitudinal ligament calcification. Here and in the thoracic areas, radiculopathy has a greater incidence because of the higher likelihood of cord compression.
Lumbosacral stenosis is more associated with radicular pain and painful cramping, tingling, or weakness in the legs. The lowest levels, particularly L4–L5, are most likely to be affected because of the larger dorsal root ganglia diameter in the face of foraminal stenosis and other stenotic disorders that come with aging. Medical management of underlying causes and their resultant SCI tends to be effective. Otherwise, decompression and perhaps fusion surgery may be necessary. Long-term benefits show greater improvement in symptoms and quality of life after surgery.
Anesthetic management is dependent upon the cause, specific anatomical areas involved, extent of SCI, risk of blood loss, or intraoperative risk of new SCI.
d. Spondylolisthesis. Spondylolisthesis can precipitate SCI when IVD degeneration narrows the disk space, causing buckling of the ligamentum flavum and slippage of one vertebra over another in an anterior–posterior direction. Mild rotational effects from segmental instability often coincide and degenerative scoliosis may complicate the condition. Sagitally remodeled arthritic facet joints and multiparity might be a risk factor for the development of this disease. Congenital dysplasia, which is fortunately rather rare, tends to cause more extensive SCI due to rapid progression. Its concomitant poorly developed posterior elements and transverse processes make attempts at surgical fusion quite difficult. The form that is most frequent is isthmic spondylolisthesis. It is associated with a defective neural arch developing in mid-childhood and is estimated to occur in 5% to 7% of the population over 40 years of age with a 4:1 male:female ratio. Traumatic spondylolisthesis can result from facet skipping, damage to articular processes, and/or laminal fractures. Infection, and rare boney tumor or boney metabolic disease with posterior element compromise, can also result in pathologic spondylolisthesis.
The lumbar area, especially L5–S1, is a prime site for this entity, which is rare in other spinal regions. Mild or no symptomatology is common but rare severe SCI can develop, especially with more extensive subluxation. The Meyerding classification of anterior–posterior slippage grades illustrates the percent of subluxation based on lateral x-rays measuring distances from posterior edges of adjoining vertebrae [19].
Conservative therapy is often successful, with up to 70% of Meyerding Class 1 and 2 patients (<50% subluxation) improving quite well. In a minority, signs for surgical intervention include Meyerding Class 3 or 4 (50% to 100% subluxation), progressive subluxation beyond 30%, and perhaps cosmetic or gait deformity. Outcomes are often good with a variety of procedures such as decompression, posterior fusion, pedicle screw fixation devices, anterior interbody fusion, and prosthetic facet joint replacement, but almost 20% of the more complex techniques result in neuronal deficits.
Anesthetic requirements are similar to those previously discussed, with possibly more blood loss depending on the extent of surgery.
e. Osteoporosis. Osteoporosis is a generalized skeletal boney disorder found in 10% of the population in the United States, characterized by reductions in bone mineral density with altered microarchitecture leading to fractures. Spinal fractures are rather numerous, particularly compression fractures of the thoracic or lumbar vertebrae. Primary osteoporosis includes type I (postmenopausal) and type II (senile osteoporosis) found mostly in over 70 years of age population. Secondary osteoporosis is related to genetics, endocrine disease, inflammatory disease, hypogonadal syndromes, calcium balance disorders, and chronic steroid use.
Anterior compression is most likely in the thoracic spine and kyphosis may develop. Pain amenable to conservative therapy is the commonest result. When indicated, vertebroplasty or kyphoplasty is a suitable surgical alternative.
For anesthesia providers, vertebroplasty and kyphoplasty are frequently performed under monitored anesthesia care. Layton et al.’s [20] study on 552 patients undergoing 1,000 fracture vertebroplasties documented a drop in the general anesthesia rate from 96% to 3%.
f. Scoliosis. Scoliosis or spinal curvature greater than 10 degrees is of three types: Congenital (∼15%), idiopathic (∼65%), or neuromuscular (∼10%). Up to 5% of pediatric populations have some scoliosis, especially in females and increasing with age. Neuromuscular causes include degenerative disease, osteoporosis, infection, and trauma.
Curvatures are most prevalent in lower thoracolumbar and upper lumbar regions.
Backache is most common, but progression to radiculopathy, fatigue, and respiratory compromise may also occur. Uniquely, thoracic scoliosis may develop restrictive pulmonary disorders correlating directly to the degree of deviation as the spinal column angle worsens from 60% to 100%. Patients may experience dyspnea and should undergo periodic pulmonary function studies to monitor progression.
Conservative therapy is normally sufficient but surgical criteria uniquely include cosmetic appearance and respiratory insufficiency.
Anesthetic providers are mindful that major corrective, fusion surgery for scoliosis is often extensive enough to require two stages to complete. Awareness of preoperative status permits detailed preoperative planning to encompass possibilities of external obstructive pressures on the airway system, pre-existing restrictive respiratory effects, IOM use, and large-scale blood loss. Anesthetic choices might include ≤0.5 minimum alveolar concentration (MAC) adaptations to volatile anesthetics with supplemental intravenous propofol and narcotic to prevent IOM effects and “deliberate hypotension” methods to reduce blood losses, provided that SCI and other comorbidities are minimal. Preoperatively, awareness heightens regarding greater rates of respiratory, hemodynamic, and coagulation complications, particularly in older patients. In McDonnell et al.’s [21] retrospective analysis on 447 patients undergoing anterior procedures on the thoracic, thoracolumbar, or lumbar spine, 11% had at least one major complication (most commonly pulmonary) and 24% had at least one minor one (frequently, genitourinary). Preoperative awareness of these factors advocates for patient preparation regarding invasive lines, a “wake-up test” (because of the potential for anterior spinal artery or the artery of Adamkiewicz injuries with anterior surgical approaches), and chances of postoperative intubation.
g. Spinal dysraphism. Spinal dysraphism involves defects resulting from incomplete fusion of the neural tube during fetal development. This relatively common occurrence, one in 1,000 live births, is more frequent in the presence of maternal folic acid or vitamin B deficiency. Open neural tube defects have coexisting vertebral abnormalities, including various forms of spina bifida or myelomeningocele. When the defect fills with mesenchyme, it can evolve into fat or a spinal lipoma and often present with a tethered, “dorsally-cleft” cord (see Chapter 16).
Syringomyelia entails progressively elongating and expanding fluid-filled cavities or cysts formed within the spinal cord. CSF obstruction or spinal cord damage from multiple sources, Arnold–Chiari malformation, arachnoiditis, trauma, hemorrhage, radiation necrosis, tumors, meningitis, and idiopathic origins, can develop a syrinx.
The average age at diagnosis is 30 years, after a slow course of minor symptoms such as back pain, upper body numbness, painless hand ulcers, loss of temperature sensation, and autonomic changes. The syrinx location dictates the symptomatology and can lead to severe SCI, scoliosis, or even cranial nerve and cerebral signs. Rarely, syringomyelia patients may experience singular manifestations such as RLN dysfunction, obstructive sleep apnea (OSA), or attention deficit syndrome. Depending on the cause, CSF pressure, protein, or red cell counts may be elevated or abnormal cells may be found.
Chronic, stable syringomyelia patients, who are accurately diagnosed with nonurgent etiologies, need periodic monitoring even if placed on analgesics or physical rehabilitation. Percutaneous aspiration can temporize acute decompensation or SCI. Surgical therapy includes removal of known causes, decompression, laminectomy, duraplasty, shunting, syrinx excision, or even suboccipital craniotomy.
Avoidance of excessive neck movement and attention to rising intracranial pressure (ICP) are among major concerns for anesthetic providers.
h. Spinal neoplasm. Spinal neoplasms can be benign or malignant, but most are metastatic from prostate, breast, renal, pulmonary, or gastric primaries. Absence of valves in the spinal venous architecture predisposes to metastatic invasion. Anatomic pathologic locations correlate with diagnoses and treatment. See Chapter 13: Anesthesia for Intramedullary Spinal Cord Tumors for further details. They are classified into three groups:
(1) Intramedullary tumors (10%) reside in the interstitium of the spinal cord and are usually malignant. They may originate from glial, ependymal, or metastatic sources (ependymoma, astrocytoma, ganglioblastoma, hemangioblastoma, lymphoma).
(2) Intradural/extramedullary tumors (40%) lie within the subarachnoid space, often arising from meningeal cells or metastases (schwannoma, neurofibroma, meningioma, paraganglioma).
(3) Extradural tumors (50%) often remain outside the dura in the vertebral body or epidural space, but can extend into the spinal cord through the intervertebral foramina in a “dumbbell shape.” They may be benign, developing from cells near nerve roots (hemangioma, osteoma, bone cyst, giant cell tumor, osteochondroma). However, most are malignant (multiple myeloma, chordoma, chondrosarcoma, osteosarcoma, Ewing sarcoma, metastasis).
Symptomatology depends on the mechanism of pathologic development, causing nerve root or spinal cord impingement, stretching and erosion of neural tissues, collapse of IVDs, boney erosion, or blockage of CSF migration. An indolent course of pain (usually thoracic), fractures, deformity, radiculopathy, or cord compression may result.
Treatment varies according to the type of tumor, size, site, symptoms, concomitant disease, or even predicted life span, where palliative therapy may play an important role. Benign, intradural/extramedullary, and intramedullary tumors are more amenable to surgical excision and radiotherapy. Radiotherapy and high-dose steroid therapy to diminish vasogenic edema are temporizing measures. Chemotherapy is less successful due to difficulties in crossing the blood–brain barrier. Anesthetic planning hinges on effects of tumor location and surgical approaches as described previously. Bleeding is much more likely with neoplastic lesions but can be preempted by interventional radiologic embolization.
2. Inflammatory/infectious disorders
a. Rheumatoid arthritis. RA is an inflammatory autoimmune disorder prevalent in 1% of the general population with a threefold incidence in women. Synovial joints may produce an antigen that stimulates rheumatoid factor (RF) production. RF is an “anti” immunoglobulin that attacks autologous glycoproteins, resulting in increased inflammatory responses, polymorphonuclear leukocyte infiltration, and cytotoxic enzymes, which destroy ligaments, tendons, cartilage, and bone.
In the cervical region, RA affects C2–C4 almost exclusively. Subsequently, progression may extend to AAI (49% rate of occurrence), odontoid process migration upward (38%), and anterior subluxation of the first cervical spine.
Patients may exhibit neck pain, headache, radiculopathy, myelopathy, vertebrobasilar insufficiency (vertigo, imbalance, dysphagia, cranial nerve disorder, and so forth), and rarely, brainstem compression.
Analgesics, physical rehabilitation, and single/combination drug therapy to retard progression and induce remissions are popular. Medicines include disease-modifying antirheumatic drugs (DMARDs) including immunosuppressants such as methotrexate, antimalarial drugs such as hydroxychloroquine, tumor necrosis factor (TNF)-α antagonists such as etanercept, corticosteroids, and nonsteroidal anti-inflammatory drugs (NSAIDs).
Anterior decompression ± corpectomy with fusion or posterior decompression and fusion are favored approaches. C1–C2 subluxation may precipitate the need for emergency surgery including use of stabilizing hardware and/or bone grafting.
Anesthesia providers must ensure that airway management produces little cervical motion. Hypoperfusion should be prevented in vertebrobasilar insufficiency cases. Concern is often directed toward the interactions and effects of pre-existing drug therapy.
b. Ankylosing spondylitis. The diagnosis of AS is carried by 0.02% to 0.20% of the population. Of this population, 80% have an onset before 30 years of age while 10% to 20% become symptomatic before 16 years of age. The male to female ratio is 2 or 3 to 1 with greater severity of radiologic evidence of disease in men, but better functional capacity than women. Caucasians (90% to 95%) and African-Americans (50%) can express the HLA-B27 genotype, a class I surface antigen on B chromosome that is associated with seronegative spondyloarthropathies and autoimmune disease.
This chronic, progressive inflammatory disease is characterized by enthesitis or inflammation of skeletal ligamentous boney insertion foci, especially in vertebral and sacroiliac articular/para-articular structures. Osseous excrescence or syndesmophytes form and attach to ligaments, bridging across calcified IVDs. An ingrowth of synovial material into joints, myxoid bone marrow, and superficial cartilage results in destruction with joint fusion. Paradoxical osteopenia may develop secondary to decreased joint motion, increased osteoclasis, and degenerative erosion by inflammatory cells. In the cervicothoracic area, where 75% of AS fractures occur, the disease may progress to severe kyphotic deformity, accompanied by a myriad of health and psychosocial problems.
Related comorbidities include peripheral polyarthritis (40%), aortic valve thickening (42% to 82%), aortic regurgitation (45%), aortitis (5%), restrictive lung disease (42%), renal amyloidosis (15%), and uveitis or iritis (30% to 50%).
Symptoms revolve around low back pain and limited spinal flexion, plus ankylosis and kyphosis advancing in a caudad to cephalad direction. With increasing ankylosis toward the point of “chin-on-chest” sign, dysphagia may follow, causing lack of oral hygiene, formation of mouth ulcerations, malnutrition, weight loss, anemia, fatigue, declining general hygiene, and eventually social estrangement.
Medical treatment is aimed at decreasing inflammation by use of immunosuppressant, DMARD, TNF-α antagonist, and NSAID therapy.
Surgery to stabilize the cervical spine is a challenging scenario for anesthesia providers, particularly when reaching the “chin-on-chest” stage (Fig. 12.2). Preoperative assessment of severe cervical kyphosis, difficult airway, or SCI often makes awake-FOI the safest airway management plan, especially when even the site for a tracheotomy is obliterated by the mandible juxtaposition. Often, the inhibited mouth opening will require preparations for a nasotracheal route. During nasal local anesthesia application, epistaxis must be avoided in an already compromised situation and contraindicates utilization of blind nasal intubation (BNI) methods. Cardiac, respiratory, renal, and malnutritional disorders present an interesting challenge, necessitating preoperative optimization and plans for extensive intraoperative monitoring.
c. Osteomyelitis. Osteomyelitis of the vertebrae and/or IVDs occurs in ≤2.4% of the population, particularly in older patients. The most typical site of infection is the lumbar spine and then the thoracic. Single-proton–emission computed tomography (PET scan) is the most sensitive early radiologic indicator of osteomyelitis.
Symptoms include back pain and spasm that can be treated with analgesics and a 4- to 6-week intravenous course of intravenous antibiotics, followed by oral therapy as outpatients.
Older patients are more susceptible to rare epidural abscess formation, primarily in multiple thoracolumbar and posterior column segments. Formation tends to develop from contiguous spread of infective osteomyelitic organisms, boney erosion, vertebral collapse, bacteremic seeding, and/or direct postsurgical inoculation or invasive needling. Pathologic expansion causes severe back pain, fever, radiculopathy, and myelopathy. Immediate antibiotic therapy plus debridement, drainage, and/or decompressive laminotomy are indicated for abscesses. In patients who pose high surgical risks, especially those with a fixed SCI (beyond 72 hours), antibiotics and possibly CT needle aspiration is a therapeutic alternative.
Anesthesia provider concerns for osteomyelitis or epidural abscess surgeries are similar to that for decompressive SCI in addition to addressing infection, its systemic effects, and intense pain, even during CT needle aspiration.
3. Demyelinating diseases and upper neuron disorders of mixed origins. Genetic, autoimmune, infectious, chemical, or idiopathic factors may result in myelin sheaths destruction or neuronal damage. Some demyelinating (e.g., multiple sclerosis) or upper motor neuron (e.g., amyotrophic lateral sclerosis) disorders originate within the CNS, while others begin more peripherally and spread centrally, perhaps gaining access through the blood–brain barrier. Symptomatology depends on regions affected and the degree of neurologic involvement. Most patients who develop SCI are treated with medical therapy and/or physical therapy and/or with surgery, as indications arise.
Anesthesia providers often choose monitored anesthesia care for these exceedingly debilitated patients with a view to minimize psychotropic, respiratory depressant, and cardiovascular depressant agents.
B. Traumatic spinal cord disorders. Traumatic SCI is defined exactly as stated, with symptoms ranging from mild pain to life-threatening myelopathy. Both traumatic cervical SCI and thoracic/lumbar/sacral SCI have singular characteristics. These patients must be placed in a system to treat existing damage and prevent secondary neurologic injury (SNI). SNI is CNS damage due to tissue ischemia, excessive spine movement, or spinal compression. Vale et al. [22] followed 77 patients who were hospitalized with SCI for whom immediate immobilization and resuscitative measures including intravenous fluids, blood products, and/or vasopressors were instituted to sustain a MAP of ≥85 mm Hg, in order to avoid secondary injury. Selected patients meeting criteria for decompression and/or stabilization and fusion had those surgeries performed. The authors found that neurologic outcomes improved on the average of one ASIA grade in most patients. Even those with complete injuries, had a 10% to 30% chance of walking, while 10% to 20% had a chance of returned bladder function within 12 months of injury.
The incidence of concomitant spinal cord injury (SCI) involved with any major blunt “head-trauma” patient is in the range of 5% to 8%, while for those suffering multiple, blunt bodily injuries it is almost 8%. Older patients have a 10-fold greater chance of simultaneous cervical SCI in comparison to children. Conversely, of patients admitted with known cervical SCI, approximately 33% have head injury. Approximately 20% of patients with SCI have multiple spinal levels of damage.
5
1. Traumatic cervical spine injury. In the setting of generalized trauma patients, the National Emergency X-radiography Utilization Study (NEXUS) group’s prospective research with radiologic confirmation of damage, noted that cervical SCI was discovered in 2% of cases [16]. Over 50% of the cervical SCI patients who developed neuropathy or complete/incomplete tetraplegia suffered injury at C6 or C7, while approximately 33% had C2 damage.
Whiplash is the most frequent cervical spine injury and is most often caused by acceleration/deceleration forces. With frontal collisions, the upper vertebral areas are directed anteriorly while the lower ones appear to shift posteriorly. This may damage cervical muscles, IVDs, the alar ligament complex, and/or facet joints, causing dislocations and fractures. Rarely, IVDs suffer annular tears or herniation and ligaments can rupture, risking nervous system impingement.
Some human and simulation studies indicate that frontal impact is most likely to result in abnormal motion involving lower (C6–T1) and middle (C2–C5) cervical areas. Other simulation studies indicate that rear-end collision is most associated with a predominantly hyperextension effect and if violent, is more prone to injure the lower cervical (C5–T1) spine.
Symptomatology from sprained muscles, tissue damage, and/or SCI is dependent upon the pathologic insult. Patients with underlying spondylosis or other compromising disorders are subject to develop cervical myeloradiculopathy.
The development of the Emergency Medical Services (EMS) in 1971 and initiation of spinal immobilization decreased by 10-fold the occurrence of SNI in cervical spine risk patients. Subsequently, as a direct result, the historical estimate of ≥25% chance of suffering an SNI dropped to 1% to 3%.
For simple cervical injuries, such as neck strain or a mildly herniated disk, medical treatment, physical therapy, and/or soft collar immobilization is sufficient [23]. For more significant SCI, rigid immobilization devices and further therapy are recommended. Early surgical correction including decompression, diskectomy, and/or fusion for severe cervical spine trauma is advised.
Anesthesia providers must be diligent in preventing SNI by precise airway management and maintenance of appropriate monitoring and operative care. Concomitant bodily injuries, particularly to the head, should not be overlooked.
2. Traumatic thoracic/lumbar/sacral spine injury. Classically, spinal trauma tends to be inflicted on more mobile spinal locations. Thus, 75% of SCI insults involve C3–C7, while the next most commonly injured area is at L1, affecting 16% of SCI patients. Holmes et al.’s review found that thoracolumbar injuries were detected in 6.3% of patients suffering from general trauma [16].
At higher thoracolumbar levels of SCI, complete or incomplete paraplegia may result, whereas neuropathy is possible at any level. Frequently, there may be injuries to other organs since spinal trauma in the thoracic/lumbar/sacral regions requires more force to cause SCI. Cardiac, pulmonary, and chest wall injury are more likely to accompany thoracic spine trauma whereas fractures at the L1 transverse process may be associated with ipsilateral renal trauma. Lumbar spine trauma is often accompanied by intra-abdominal and diaphragmatic damage.
Most thoracic and many lumbar fractures tend to be more stable, reliably treated with immobilization and conservative therapy. In patients with thoracolumbar compression or burst fractures with no signs of neurologic injury, no loss of vertebral body height less than 50%, and no narrowing of the spinal column of less than 30%, random treatment protocols with braces, casts, physical therapy, and/or postural instructions for 6 to 12 weeks showed no difference in outcome. Sacral spine injuries, which are 20 times less frequent than lumbar injuries, are very amenable to conservative therapy.
For anesthesia providers, attendance to immobilization, invasive monitoring for hemodynamic control, and blood product acquisition will depend on the injury and type of surgery.
5
IV. Special considerations with complete spinal cord injury. Many bodily systems may be affected by complete SCI (paraplegias, tetraplegias, and so forth) prompting patients to undergo repeated surgeries and anesthetics. Some coexisting factors and complications related to SCI may have significant impact on patient care.
If patients require airway control, the presence of semirigid collars, halos, and cervical fusions all tend to spur anesthesia providers into implementing protocols for management of a difficult airway with minimal cervical motion.
SCI patients may incur a wide spectrum of respiratory aberrancies. Abnormal pulmonary functions include up to 32% drops in vital capacity during changes from seated to supine positions. In the supine position, decreases of up to 70% mean vital capacity and 45% forced expiratory volume in 1 second can occur. Complete injury at C3–C5 results in ventilator dependency. Some patients may be assisted and have fewer infections following special surgeries such as diaphragmatic pacing. Respiratory infection may result from hypoventilation following diaphragmatic, thoracic, and abdominal muscle paralysis with impaired coughing ability to clear secretions and increased aspiration risk. Pulmonary embolism is a complication associated with venous stasis and DVT. Also finally, hypotension due to neurogenic shock may provoke excessive fluid administration, resulting in pulmonary edema.
5
In these situations, providers have a heightened awareness of intolerance to hypoxemia and likelihood of respiratory deterioration, particularly during acute airway management. Supplementary oxygen, ventilatory techniques, and suctioning are worthwhile.
5
Patients with complete SCI are more susceptible to cardiovascular abnormalities such as early hypotension from neurogenic shock and/or concomitant organ injury. Treatment goals include limiting crystalloids to 2,000 mL to prevent pulmonary edema and acute respiratory distress syndrome, using fluid plus vasoactive therapy to achieve systolic BP levels ≥90 to 100 mm Hg and heart rates 40 to 100 beats per minute, maintaining oxygenation, and keeping urine output at ≥0.5 mL/kg/h. Days to months later, hypotension may develop from sympathectomy, infection, hypoproteinemia, anemia, and/or insufficient fluid intake.
1
SCI patients with complete levels at T6–T7 or higher have a greater possibility of autonomic dysreflexia. When planning for anesthetic management during surgery (more commonly nonspinal types), dysreflexia risks can be completely avoided if anesthesia providers choose neuraxial blocks. Continuation of epidural block postoperatively provides the same benefit, particularly in those with a history of frequent events.
Hypothermia is a significant problem in tetraplegics or paraplegics, both in the acute phase of spinal shock and in chronic cases, where patients have a relatively greater surface area due to weight loss. Active fluid warming, use of warming blankets, and increasing ambient temperature stave off excessive hypothermia and consequences such as infection, postoperative shivering, and adverse coagulation effects.
Patients with lack of sensation, contractures, and pressure sores may require special padding and diligence to prevent additional injury. Catheterizations, urine retention, and ureteral reflux expose SCI patients to repeated infections and kidney failure problems that anesthesia providers must consider. In addition to effects of sepsis in these situations, the safety of regional anesthesia must be evaluated.
Periodically, effects of SCI may actually alter anesthetic choices [24]. Spasticity may preclude the use of monitored anesthesia care if spastic movements have a chance of causing field motion or impeding surgical technique. Large-scale paralysis causing upregulation of acetylcholine receptors 12 to 24 hours beyond the onset of SCI is a contraindication to succinylcholine administration for fear of precipitating severe hyperkalemia. Prior to 12–24 hours, there is insufficient time for significant upregulation and administration is safe, theoretically. The duration of contraindication may span one or more years or even forever, especially in the face of fluctuating neurologic deficit.
Finally, after major spine surgery, pain control is most problematic in patients receiving chronic, high-dose analgesic regimens.
V. Management approaches for spinal cord injury. The main objective of therapy is to improve recovery from the effects of SCI, halt worsening of the condition from whatever caused the injury, and prevent secondary neurologic damage. Acute SCI is a more “immediate, therapy-intensive” situation and even subacute or chronic SCI cases may fall into an urgent status category. This discussion will provide an overview of the two major modes of treatment for acute SCI: Conservative medical and/or physical therapy or surgical therapy with anesthetic management.
A. Medical/physical therapy
1. External immobilization. Initial treatment phases of most trauma/suspicion for acute SCI frequently include immobilization in the prehospital setting. Immobilization consists of a hard collar (superior restriction to soft ones) for cervical injuries or a backboard plus strapping/taping, or orthopedic brace for cervical/thoracic/lumbar injuries. Interestingly, for penetrating trauma patients, immobilization is not recommended unless SCI is highly suspected, and may have been harmful to a small percentage of those immobilized, by causing twice the mortality rate, perhaps due to delayed definitive therapy. For thoracolumbar risk, there does not seem to be a consensus on the value of immobilization, unless criteria for surgery exist. Studies on thoracolumbar spinal fracture patients with no specific surgical criteria, who randomly underwent surgery versus conservative therapy and similarly treated lower cervical spine patients showed that surgically treated patients had greater speeds of recovery, less pain, and returned to work faster than conservatively treated ones. However, the middle long-term effects of the two therapeutic courses were comparable for residual pain, quality of life, and residual employment-limiting disability.
In cervical spine patients, immobilization may involve use of a halo. Halos are the most restrictive but do not totally prevent movement. Complications of halo fixators include scars, pressure sores, infection, pins getting loose, periorbital edema, burns at pin sites during MRI, osteomyelitis, penetration of the cranium and subdural abscess, VAE, nerve palsy, and failure to prevent motion.
Chan et al.’s [25] review revealed that of 21 healthy subjects immobilized for 30 minutes on a backboard, 100% developed head, sacral, lumbar, and mandibular pain. Indeed, half considered the degree of pain to be moderate to severe and 29% developed more symptoms within 48 hours. Considering these results, and the fact that backboards allow greater degrees of cervical motion compared to vacuum mattresses, perhaps this information should result in a recommendation that EMS services eliminate backboards in lieu of purchasing mattresses.
2. Temporizing therapy (analgesics and anti-inflammatory drugs). For conservative therapy, opioid analgesics, tramadol, oxycontin, gabapentin, pregabalin, or fentanyl patches alone or in combination may be administered to make pain tolerable. Judicious dosing avoids the loss of patient cooperation, prevents inability to perceive neurologic changes, and stays away from adverse hypoventilation. Attentiveness to ventilation must be greatest in the presence of coexisting respiratory embarrassment from SCI, other traumatic respiratory disorders, or concomitant cerebral injury with increased ICP.
Beneficial nonsteroidal anti-inflammatory drugs (NSAIDs) such as ibuprofen, acetylsalicylic acid, naproxen, indomethacin, mefenamic acid, or piroxicam which all reduce prostaglandin synthesis, should be avoided in the face of “high risk-of-bleeding” acute SCI or contraindications. Acute pain from less-damaging SCI, such as a herniated disk, might be treated with a week-long course of potent anti-inflammatory steroids (prednisone) to inhibit lipid peroxidation and calcium influx. Epidural, interlaminal, or transforaminal steroid injections under fluoroscopy are useful, but best avoided if concerns for SCI are present, except in cases unlikely to be candidate for other therapy.
3. Cervical traction or lumbar traction. Cervical traction with cerebral tongs and a 20- to 40-lb weighted pulley system can be used for cervical radiculopathy, disk herniation, or neural foraminal stenosis. Lumbar traction with a hip padding system and pillows under the feet for acute SCI (<6 weeks from onset) has had considerable success. Vertebral separation can decrease spinal cord pressure, lessen disk herniation, or modify pressure on disk bodies. Length of immobilization time, possibility of pressure sores, and hygiene factors are disadvantages.
4. Spinal cord protection
a. Implementation of moderate hypothermia. Hypothermia has been studied considerably as a protective strategy for cerebral ischemia. Because of the many parallels between the spinal cord and the brain regarding effects of metabolism, autoregulation, and ischemic injury, some clinicians think that hypothermia may be a “cord-protective” proposition. Hypothermia decreases metabolism fourfold per 10°C, maintains adenosine triphosphate (ATP), and reduces glutamate release, excitotoxicity, inflammatory changes, vasogenic edema, neutrophil response, calpain activation, and apoptosis. A prospective, definitive study is still needed to determine the relationship between SCI and hypothermia.
b. Recent/future therapeutic alternatives. An assortment of therapies has been investigated in animal studies and human subjects with varying success while searching out the best treatment for SCI. These include angiogenic protein, Schwann cell transplantation, gangliosides, calcineurin inhibitors such as cyclosporin A and tacrolimus, omega-3 fatty acids, adult stem cell transplantation, riluzole, and transplantation of many cell types, such as bone marrow cells onto damaged spinal tissue.
c. The high-dose steroid therapy controversy. In 1990, a prospective acute SCI outcome study by the Second National Acute Spinal Cord Injury Study (NASCIS II) used high-dose methylprednisolone (HDMP) with the object of ameliorating the effects of SCI and preventing secondary damage [26]. The study assessed only unilateral neurologic testing and found no benefit overall (according to ASIA, motor, or sensory scores) in the HDMP group, compared to naloxone or placebo groups. In the NASCIS III study, 24 to 48-hour infusions of HDMP compared to tirilazad showed no overall benefit between groups [27]. A trend toward sepsis, pneumonia, gastrointestinal bleeding (1.5-fold), wound infection (2-fold), pulmonary embolism (3-fold), and death was detected in the HDMP group.
On post hoc analysis, both studies found a subgroup that had improved neurologic changes, but the magnitudes were not objectified. In spite of this, the authors recommended that patients with acute SCI should be treated with HDMP for 23 hours, initially 8 hours postinjury, and after their next study, at 3 to 8 hours postinjury. Perceived pressure to administer anything that might be beneficial for SCI, made use of HDMP very popular.
Some researchers questioned this therapy’s validity, particularly considering risky adverse side effects. Recently, meta-analysis of the literature found that significance of the subgroup data was unknown. Multiple independent reviewers had trepidation regarding randomization, clinical endpoints [28], statistical analysis, plus the lack of having levels I, II, or even the majority of level III evidence clearly demonstrated that HDMP definitely was not warranted to be considered as a standard of care or indeed, even as a recommendation for SCI patients. They warned of adverse complications with HDMP far outweighing the “limited suggested benefits.” Presently, the Canadian Association of Emergency Physicians (CAEP) has renounced the use of HDMP and the Congress of Neurosurgeons has stated that its use is more likely to be associated with adverse side effects rather than clinical benefit.
B. Surgical management
1. Indications for spinal cord injury surgery. Halo immobilization may be insufficient to prevent cervical spine motion from deteriorating into acute SCI, progression of existing SCI, or development of SNI. Radiographic evidence of fracture site motion in the upright position may occur in 77% of patients with halos. Studies have additionally demonstrated that surgery can be more beneficial than immobilization techniques.
Criteria for surgical treatment of SCI include: (1) Spinal instability, (2) intractable pain unresponsive to conservative therapy for ≥6 weeks, (3) progressive neurologic deficits, and/or (4) progressive spinal cord or nerve/nerve root compression with pain or neurologic deficit. Other indications may involve the following: (1) Adequate medical status and stability following numerous organ injuries, (2) radiographic evidence of intrinsic damage to the spinal cord, (3) significantly extensive bone, disk, fracture/dislocation, or hematoma compression, and (4) inability to immobilize or prevent spinal instability through closed reduction, rigid external immobilizers, or natural healing.
Acute SCI is frequently considered an urgent problem if the need for decompression is apparent. The idea of urgency in timing was advocated because of numerous experimental projects suggesting greater chances of irreversibility or SNI beyond certain lengths of time. For acute spine or SCI what is the optimal timing for surgical correction and/or stabilization? Review articles researched this question and found no class I evidence to support improved neurologic outcome after “early” institution of surgery (8 to 72 hours). Studies were retrospective, poorly randomized, and had contradictory outcomes with levels II to IV evidence [29]. Regardless, some authors felt that newer evidence tended to be inclined toward the safety of early surgical therapy and that outcomes are associated with faster and potentially better recoveries following surgery within 24 hours of traumatic SCI onset.
2. Types of surgical procedures and anesthetic caveats. Once a patient meets surgical criteria, a number of techniques are available. Many are very beneficial, but even these are fraught with the possibilities of persistent or worsening symptomatology, adjacent degenerative changes due to motion abnormalities and complications.
Newer surgical techniques are beginning to be explored. Among these are artificial disk replacement and artificial facet replacement techniques designed to permit more natural movement of the spine in contrast to fusion surgery. Although they boast shorter recovery periods, outcomes have not been encouraging. There was insufficient support in the literature to recommend these procedures and further intimated possibilities of hazardous revision after pre-existing artificial disk surgery. Disk replacement surgery in 93 patients with no control group revealed results indicating that adjacent spine degeneration was lessened by 10% [30]. However, many patients fared worse clinically than they were beforehand, with more limited motion and progression to facet joint degeneration. This finding was highly informative since facet joint disease and spondylosis are listed among contraindications to this surgery. Facet joint implant is yet another unproven technique, performed since 2007 in Europe. Studies there had no controlled outcome and the method is only now being researched in the United States.
a. Anterior cervical diskectomy. Anterior cervical diskectomy (ACD) is an approved technique employed for patients diagnosed with herniated cervical disks. Through anterior neck approaches, plans are executed for repairing a disk, removing fragments, or performing complete diskectomy, frequently with removal of nearby osteophytes.
These patients tend to have few risk factors regarding cervical motion. However, if present, then airway management techniques should be chosen according to the method with which anesthesia providers feel most expert [23]. If general anesthesia (GA) and ETT are planned, laryngotracheal anesthesia (LTA; 4% lidocaine) spray to the trachea minimizes surgical stimulation and lowers anesthetic requirements. Muscle relaxants help prevent movement, but may have to be abandoned if MEPs are monitored.
This surgery is less complex than diskectomy with fusion. IOM selection depends on the degree of SCI and risk of surgery. Invasive hemodynamic monitoring, additional intravenous access, or blood products are unlikely.
Anesthetic agents should be chosen with the object of early postoperative neurologic testing. Stomach decompression eliminates gastric distension and at least two good antiemetics assist in achieving a “quiet” emergence from anesthesia to avoid gagging, coughing, hypertension, and hematoma formation. Intravenous lidocaine, judicious lowering of inhalation agent, continuous small-dose remifentanil infusion or other narcotics, and “near the end of surgery” use of nitrous oxide/oxygen can make for a very smooth wake-up. Postoperative pain is minimal, especially after local anesthesia in the incision.
b. Anterior cervical diskectomy or corpectomy with fusion. For patients with unstable cervical spines or excessive spine motion, ACD and fusion (ACDF) is often chosen. Anterior corpectomy (removal of the vertebra) with fusion may be considered if the vertebral body is excessively eroded. A patient’s hip site for a donor bone graft is usually is the greatest source of postoperative pain. This autograft or alternatively, an allograft from cadaver donation can be used as a solid stabilizing plug or groundup and packed into the fusion site between vertebrae and/or around instrumentation. Fusion will eventually result at vertebral levels above and below the disk site after the bone graft is inserted or if the entire disk endplate is excised.
Awake- or asleep-FOI, or other intubation method with manual in-line stabilization (MILS) is advised for at-risk cases. Anesthesia providers must avoid complications of ETT-surgical retractor-related RLN palsy associated with this surgery [31].
If IOM indicates little or no SCI, BP can be kept in the low normal range to lessen bleeding. An arterial line is recommended if tight BP control is required for adequate perfusion in the face of SCI or if greater blood loss is anticipated from ≥2 levels of fusion. Tumor pathology or corpectomy plans increase blood losses and needs for appropriate lines and blood products. IOM will mandate keeping inhalation agents at ≤0.5 MAC. Supplementary propofol or dexmedetomidine can assist with hypnosis. TIVA may be required if the initial EPs are so poor that inhalation agents are contraindicated.
Occasionally, a surgeon may request aid by asking for cervical traction to create sufficient space for boney grafts or “plugs.” While carefully keeping fingers out of the surgical field, anesthesia providers exert a constant, slow cephalad pull on the angles of the mandible until the surgeon indicates when to maintain position. The provider can slowly let go after being informed that the graft is in place. An alternative is to pull on the cord of a cervicocranial traction device, if present. Subsequently, plates are added for further stability.
Similar to ACD, wake-up should be gentle and quite early. Postoperative pain is mild to moderate.
c. Posterior diskectomy, hemilaminectomy, or laminectomy/fusion. Posterior diskectomy surgery is targeted for patients with posteriorly protruding disks. Hemilaminectomy is a posterior body surface approach designed to eliminate encroaching bone, impinging ligaments, and/or herniated disks by cutting lamina to free up the spinal cord and nerve roots. With pre-existing spinal stenosis and myelopathy, the entire lamina at one or more levels needs to be excised (laminectomy). If boney removal is sufficient, an otherwise impinging disk may be left untouched because it is now “free.” Vertebral movement is unlimited after this surgery. Motion-limiting fusions are undertaken to provide stability by performing a laminectomy and/or diskectomy followed by insertion of interbody stabilization instrumentation and ground-up bone for structural support.
Although these are lower-risk procedures, anesthetic concerns for venous access, blood availability, and invasive monitoring depend on the extent of surgery, patient comorbidities, and diagnosis. For cervical surgeries, care is required to avoid excessive neck flexion, particularly with pre-existing SCI. Soft, prone headframes or pins are employed for facial protection. Prone positioning is a concern for the usual reasons including ensuring sufficient room for abdominal and chest expansion to prevent restrictive ventilatory effects.
d. PLIF, TLIF, ALIF, and XLIF. These acronyms are attributed to approaches for fusion of at least two vertebrae for patients with diagnoses of scoliosis, spondylolisthesis, spinal fractures, or multiple severely deteriorated disks. Surgeries may be minimally invasive or may be quite extensive.
A posterior lumbar interbody fusion (PLIF) is approached through a posterior midline incision. After laminectomies and diskectomies, vertebral surfaces are prepared and ground-up bone and instrumentation is placed.
A transforaminal lumbar interbody fusion (TLIF) approaches from a posterior midline incision but aims more posterolaterally through less muscle with less risk of nerve disruption. Otherwise the salient objectives are similar to the PLIF.
Anterior lumbar interbody fusion (ALIF) is akin to PLIF but is approached anteriorly or laterally.
The extreme lateral interbody fusion (XLIF) is an analogous technique completed through a number of small incisions in the flank for a maximum of perhaps two vertebral levels.
These extensive surgeries frequently involve time spent on major boney stressing anesthesia considerations for airway management, respiratory status, invasive lines, IOM and anesthetic interactions, positioning, monitoring and treatment of major blood loss/coagulation abnormalities, temperature control, wake-up tests, ischemic optic neuropathy (ION), extubation, and postoperative concerns.
Providers are keenly aware of when pedicle screw testing is needed with regard to effects of neuromuscular blockade (NMB). The sought-for response is similar to watching for nervous system irritation on free-run EMG, except that the surgeons specifically use a probe to test on the screw. Needle electrodes record from appropriate nearby muscles that correlate with nerve root innervation. The purpose is to detect screws that have penetrated the spinal canal or breeched the pedicle wall, resulting in direct contact with nerve roots. Muscle responses to lower currents (<6–10 mA) indicate excessive proximity and suggest surgical adjustment. Chronically compressed nerves may have higher thresholds of concern.
Individual nerve root identification and threshold testing are performed in a similar fashion during surgeries where spinal tumor excision, boney work, or freeing up of a tethered spinal cord is planned. In these cases, a probe in the operative site emits low levels of electrical stimulation so that responses and thresholds are measured in corresponding muscles. Dermatomal EP responses are highly sensitive to volatile agents. With the likelihood of chronically injured nerve roots, many anesthesia providers recommend against muscle relaxants. See Chapter 26: Electrophysiologic Monitoring for further details.
Transthoracic approaches for anterior and lateral pathology often necessitate one-lung ventilation by a double-lumen ETT or bronchial blocker, or hypoventilatory jet ventilation techniques. A double-lumen ETT in a patient in a prone head holder can be problematic if the ETT has shifted and is unable to isolate the lung. It may be very difficult to adjust through the small frame opening after softening body heat causes it to become excessively flexible.
e. Minimally invasive spinal surgery, microdiskectomy. Endoscopic or minimally invasive spinal surgeries utilize small incisions. These posterior, posterolateral, anterior cervical spine, or extreme lateral endoscopic approaches have the advantage of using dilation technology to traverse soft tissues.
Indications include spinal or foraminal stenosis, facet joint cysts, cauda equina syndrome, or radiculopathy accompanying smaller-sized disk herniation with motor dysfunction. Spinal instability and central canal impingement are contraindications.
General ETT anesthesia is preferred, although monitored anesthesia care is possible in highly motivated patients unlikely to move body parts. Preparation must always be considered for conversion to GA.
f. Vertebroplasty and kyphoplasty. During vertebroplasty under x-ray guidance, a hollow bore needle is inserted within a collapsed vertebral body for cement injection to strengthen its structure. The kyphoplasty method inserts a dilating balloon through the needle to expand the vertebral body. After the balloon is deflated and withdrawn, cement is injected into the “cavity.”
Both surgeries are easily amenable to monitored anesthesia care. Anesthetic providers should remember the distinctive problems that arose in Layton et al.’s series of patients, including a 1% incidence of rib fracture or rib pain secondary to prone positioning and an episode of cement-induced pulmonary embolism [20].
VI. Anesthetic management for surgical spinal cord injury procedures. The bulk of this description of anesthesia for SCI will be directed toward anesthetic strategies for patients with acute cervical SCI and/or surgery with the possibility of major blood loss. Preoperatively, patients should already have had maximal evaluation of history, physical examination, MRI and/or necessary testing. There are many unique aspects in anesthetic care. When indicated, five important areas include explanations for awake-FOI or other airway management, wake-up testing, use of invasive monitors, postoperative intubation, and postoperative pain management. The degree to which laboratory data can be obtained, preanesthetic discussions completed, and anesthetic plans developed, depends on the speed and urgency for surgery.
A. Optimization of patient status, psychological preparation, premedication. The patient should be on optimal therapeutic regimens for surgery if possible, with regard to volume status, laboratory data, and major organ systems function. When anticipated, major spine surgery should prompt blood bank requests for availability of packed red blood cells (PRBC), platelets, cryoprecipitate, arginine vasopressin, coagulation factors, and/or FFP. Keeping eight units of PRBC or a major trauma protocol in nearby refrigerators is most efficient.
Complete airway assessment and knowledge of neurologic examination data are extremely important. A thorough, reassuring, and explanatory preanesthetic visit is an extremely worthwhile psychological preparation of the sequence of events from induction to the postoperative phase. Discussing the importance of awake-FOI while giving reassurances and comparisons of local airway anesthesia to otolaryngology throat examinations with sedation makes it very tolerable.
A case could be made for explaining the “wake-up test” to all patients undergoing SCI surgeries where risk to the motor system may occur. Fear is allayed by descriptions of being asleep throughout the surgery and having a wake-up test similar to waking up with heavy narcotics once the surgery is finished. Patients should be informed of not being able to open taped eyes to prevent injury, and not being able to speak temporarily because of a “breathing tube” that probably will not bother them. The provider should explain requests to squeeze their hands, move their feet, and most importantly, not move the rest of their bodies. Going back to sleep after a few moments of testing is also conveyed. Remarking that most patients typically do not remember anything bad about the wake-up test is very much appreciated.
An explanation of arterial, central, and peripheral intravenous lines should be given with reasons for placement, sensations felt after awakening, and possible risk.
Patients with difficult airways, complex cervical surgery (especially simultaneous anterior–posterior), lengthy surgery in the prone position, or high volume resuscitation are prime candidates for postoperative intubation. Explaining the benefits, concomitant sedation, sensations, and expectations for extubation is important.
One method of discussing postoperative pain relief expectations with patients might relate pain judged on a scale of 0 to 10, where 10 is the worst pain imaginable. Meeting expectations is more likely with descriptions for pain scores objectives ≤3 or 4 being better than zero, where concern may arise about too much narcotic and respiratory arrest. Preempting worry about awakening at a zero score can be achieved by describing extra vigilance at that level.
Premedication for patients with acute SCI depends on surgical urgency, overall medical status, aspiration risk, airway difficulty, hemodynamic circumstances, degree of cooperation required, anxiety, presence of pain, drug therapy, allergies, age, height, and weight. Anxiolytic agents and/or narcotics should be titrated carefully in patients with significant risk and at extreme ages.
Excepting contraindications, glycopyrrolate is recommended for awake oral approaches to airway management, to improve intraoral local anesthetic absorption and airway viewing by lessening secretions.
Drugs for significant comorbidities such as anticonvulsants, antihypertensives, immunologic suppressants, and so forth may be administered if benefits exceed disadvantages. It is advantageous to administer antibiotics prior to incision unless cultures of the wound are planned at which time antibiotics should be administered after the cultures are acquired.
B. Anesthetic management
1. General anesthesia. GA is the most frequently chosen technique with benefits of controlled ventilation, freedom to deal with the complexity of anesthetic patient care, and a nonmoving field in the face of intricate spinal surgery. Complexities may involve airway management, monitoring, anesthetic choices, invasive techniques, and positioning.
a. Airway management. Striking spinal deformities, SCI, respiratory restriction, and effects of patient positioning often lead to the selection of specialized airway devices and techniques. For intubated patients, if airway pressures rise significantly, but simple causes and solutions are not found, early institution of fiberoptic examination of the ETT and airway can be invaluable, particularly when prone, and may prevent hypoxemia, lung trauma, and/or accidental extubation from ETT manipulation.
(1) Airway devices, cervical spine motion, maneuvers. Unique considerations for airway devices for spinal surgery include the use of reinforced anode ETTs, particularly in thoracic scoliosis patients to prevent boney deformity compromises of the ETT lumen. Some might consider this type of ETT to be beneficial during cervical spine surgery when the external pressure of surgical instrumentation might cause narrowing or occlusion of the ETT lumen. Two points argue against this. Firstly, external pressure might harm the RLN. If airway pressures rise during instrumentation, the surgeons should be informed so that they can make adjustments. Secondly, the wire spiraling may interfere with intraoperative radiologic studies.
Classically, the use of a rigid laryngoscope for intubation with midline stabolization (MILS) is considered a relatively rapid and reliable technique that might be preferred particularly in urgent situations. Nevertheless, considerable difficulty with this method can arise. Difficult intubation occurs in 1% to 13% of patients and impossible intubation in 0.05% to 0.35%. Complications from difficult intubation can be devastating and cervical SCI with poor mobility may result in difficult intubation.
No retrospective or prospective studies have shown adverse SNI subsequent to airway management, but isolated case reports have raised concerns about cervical motion. Of the case reports none had preoperative scans. Most but not all had unknown preoperative pathologic states with no attempt made to limit cervical motion and of the postairway management deficits, most were transient.
Both location and cause of cervical spine instability (bony vs. ligament abnormality) are significant to airway management if at-risk areas are more susceptible to movement following the use of intubation equipment. Routinely during rigid laryngoscopy (RL) usage, 40 N of force (750 mm Hg) is applied. With the dramatically lowered incidence of SNI following the EMS recommendations for spinal immobilization (one-tenth of that previously found), Crosby’s editorial noted another finding [23]. In terms of airway management, the popularity of immobilization broadened into an extrapolation of two suppositions: (1) That RL intubation causes cervical spine movement and (2) that awake intubation, when a patient could “theoretically protect the cervical spine due to intrinsic muscle tone and vocalization of symptoms” may be better than asleep intubation.
MILS is a maneuver that was developed to limit neck motion during airway management. To perform MILS, an assistant severely braces both hands and forearms on either side of the patient’s neck, between the clavicles and occipital/ear/mandibular/maxillary regions, while at the patient’s side facing cephalad or by the head facing caudally. Radiologic studies reveal that MILS decreases RL-provoked movements by 25% to 100% [32].
Problematically, employing MILS can result in 50% to 100% incidence of Cormack–Lehane grade 3 or 4 views with a doubling of RL force in an attempt to intubate the trachea, and an intubation failure rate of 10% to 33%. A doubling of motion may be encountered while using MILS while using RL in cadavers having complete C4–C5 ligamentous laxity versus RL use alone, due to the difficult view [33].
In contrast to RL usage, flexible FOI has a 98% to 99% success rate in the operating room and causes virtually no cervical motion (<5% to 10% of that with RL) in both live subjects and cadavers [34]. Note that patients can self-position after awake intubation. In anticipation of awake-FOI, antisialagogue administration (glycopyrrolate) is advantageous when given 15 to 20 minutes beforehand, particularly if local airway anesthesia is intended. Sedation must be judicious to prevent respiratory arrest and many anesthesia providers advocate supplemental oxygen. Airway conditions should be perfect by instituting glossopharyngeal, superior laryngeal, and transtracheal anesthesia. These can be accomplished by a number of approaches. For patients with significant aspiration risks, to keep airway protection intact, the latter two blocks should be carried out moments before ETT insertion by using a “spray as you go” technique down the fiberscope at the vocal cords and intratracheally. This is followed by immediate fiberscope insertion, ETT insertion, and ETT cuff inflation. FOI may also be successful after induction of GA. Coughing with local anesthetic application has not caused SNI.
ETTs with centrally bending tips improve rates of successful passage over the fiberscope and through the glottis. However, use of regular polyvinyl chloride ETT can also be successful if the most distal tip is rotated 90° or 180° from its customary direction so that the Murphy eye is parallel to the patient’s frontal or coronal plane.
Many other intubating devices can be utilized in both awake and asleep states. The introduction of the videolaryngoscope (VL) has given rise to many similar devices with ≤60 degree-angled MacIntosh-like blades including some with ETT-loading channels. Decreased spinal motion with VL devices has been attributed to their superior capability for laryngoscopic visualization and intubation. Crossover studies of normal patients with MILS found a 50% motion reduction in C2–C5 segments with the VL (Glidescope) in comparison to RL, with little difference at Oc-C1, C1-C2 or C5-T1. There is no question, however that the glottic views during MILS were better with the VL and that successful intubations had a higher likelihood. Patients placed in semi-rigid collars with their heads taped to trolleys while intubated with the RL resulted in modified Cormack–Lehane views of three and four almost 100% of the time. In contrast, the VL views were Grade 1 or 2 over 90% of the time [35]. The viewing difficulties were partially related to the collar’s anterior portion effectively reducing the mean interdental distance to 2 cm. Rigid halo fixators are even more likely to produce a difficult airway.
Decreased spinal motion has been observed with other VLs and optical laryngoscopes including the Pentax Airway Scope and the Airtraq, which are associated with 40% and 66% less cervical spine motion, respectively at Oc–C1, C2–C5, and C5–T1. Differences were negligible at C1–C2 [36].
Lightwands are battery-powered, malleable stylet-like devices with distal bulbs that can be inserted in an L-shape within an ETT for blind placement through observation of transillumination in the neck, while room lights are darkened. Jaw manipulation transmitted to the spine from lightwand usage incurred 41% to 72% less motion than RL at multiple segments involving Oc–T1 while employing MILS in normal spine patients. Successful lightwand intubation has been observed at over a 95% rate with a single attempt in elective surgery patients.
The Fastrach is an intubating LMA that employs a fixed metallic body within a much more angulated LMA covering. It is frequently used for blind intubation with its specialized silicone ETT having a very soft, centrally directed tip. Blind intubation in cadavers with no spinal instability elicited 25% to 50% of extension at C1–C3 in comparison to RL extension. In cadavers with unstable spine and axial traction, the Fastrach produced up to 50% of the movement caused by RL. Similar motion findings have been noted in living subjects. What is the success rate? In patients with cervical immobilization and position adjustments, blind intubation was successful in up to 90% by the third intubation attempt, whereas with concomitant use of a flexible fiberoptic bronchoscope through the device, successful intubation approaches 100% [37].
The Bonfils intubation fiberscope is a malleable optical stylet. In comparison studies during fluoroscopy in living subjects, it causes less extension at C1–C2 and C3–C4 than a MacIntosh RL, but atlanto-occipital distance was slightly more reduced with the MacIntosh RL [38]. When compared to Fastrach, the success rate for Bonfils intubation was 100% by the second attempt.
Cricoid pressure is another airway management technique that tends to worsen laryngeal views. It also may cause more cervical spine motion.
(2) SCI airway management outcomes and recommendations. What is expert opinion on the best intubation approach for SCI patients? Two techniques cause virtually no motion: (1) FOI, which requires patient cooperation in awake subjects or may be an expensive asleep alternative and (2) BNI, which has a smaller intubation success rate and is risky for epistaxis or other injury. Interestingly, for SCI patients, neither technique has been shown to be safer than any other intubation method. Practice surveys have yielded figures showing that a majority of anesthesiologists favor awake-FOI in 65% to 78% of cooperative patients with unstable cervical spines [39]. For the patients chosen for GA, RL use has trended downward from 66% to 8% due to greater preferences for techniques such as FOI (75%), VL (8%), and Lightwand (8%).
There are no outcome data that would support a recommendation for a best intubation technique in cervical spine at-risk patients. Experts support the idea that whichever technique is most practiced by the airway management provider is thought to be the best plan, with the caveat to include MILS. This is accepted with the recommendation for MILS by the American College of Surgeons as outlined in the Student Course Manual (7th ed., 2004) of the Advanced Trauma Life Support Program for Doctors, the Eastern Association for the Surgery of Trauma, and by experts in anesthesiology, trauma, and neurosurgery.
For supine and perhaps lateral position surgeries, supraglottic airways (SGA) could be chosen. Rarely, but more commonly in Western Europe, SGA is used in the prone position with the patient’s bed nearby. These choices would be more likely in simple, shorter duration surgery with normal low airway-risk patients. SGA do have an advantage for “smoother wake-up.”
CLINICAL PEARL
There is no known “best intubation technique” in cervical spine at-risk patients to prevent SNI. Experts recommend whichever technique is most practiced by the provider with the caveat to include MILS. Techniques associated with less spinal motion while improving glottic views by 1 to 2 grades, such as FOI or videolaryngoscopic intubation, are preferred to direct laryngoscopic (DL) intubation.

Full access? Get Clinical Tree
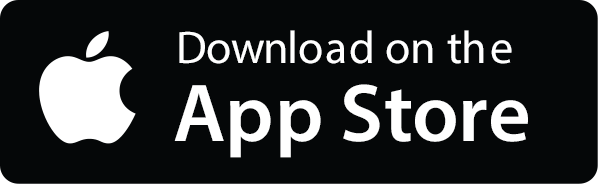
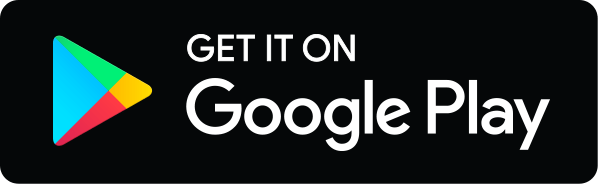