TOPICS
4. Transposition of the great arteries
6. Anesthesia for noncardiac surgery in adults with congenital heart disease
7. Cardiac surgery and pregnancy
The incidence of congenital heart defects is approximately 8 in 1000 births.1 Over the course of the past decades survival of children with congenital heart disease has improved. Today, adults can present following various cardiac surgical repairs, for routine general and obstetric anesthesia care. Unfortunately, the frequently multistaged surgical repairs necessary to improve survival in children often result in complications in adulthood. Knowledge of the anatomy of the original structural defect and the repairs undertaken is essential in the choice of appropriate monitoring and anesthetic techniques for otherwise routine procedures. Moreover, these patients may require heart surgery and/or cardiac transplantation later in life. This chapter highlights the anatomy and physiology of common congenital heart defects and outlines the repairs associated with them. In general, surgical repairs are directed at ensuring the delivery of oxygenated blood to the systemic tissues and eliminating communications between the right and left heart. Of course, for some congenital heart disease (CHD) patients the distinction of which is the right heart and which is the left heart may not be entirely clear. Consequently, when considering the CHD patient, tracing the flow of blood through the chambers of the heart into the circulation and back again provides the basis toward acquiring an understanding of CHD.
ATRIAL SEPTAL DEFECTS
Atrial septal defects (ASD) are abnormal communications between the left (LA) and right atrium (RA). A patent foramen ovale (PFO) can be present in up to 25% of the population and produces a small interatrial communication as a consequence of failure of the septum primum and septum secundum to fuse. ASDs account for 6% to 10% of the CHD population and present in a variety of ways.2 Eighty percent of ASDs are of the ostium secundum type (Figure 12–1 and Videos 12–1A and 12–1B) located in the middle of the interatrial septum. Defects located lower in the atrial septum (toward the atrial-ventricular [AV] valves) are ostium primum ASDs and are at times associated with a ventricular septal defect (VSD) as well. Sinus venosus ASDs occur close to the junctions of the superior vena cava (SVC) or inferior vena cava (IVC) with the RA. Sinus venosus ASDs are often associated with pulmonary vein anomalies and anomalous return of oxygenated pulmonary vein blood to the right atrium.
Figure 12–1. An ostium secundum atrial septal defect is seen in this schematic (ASD) pressures and oxygen saturations in the various parts of the heart are displayed.
ASDs generally produce a left-to-right shunt of blood across the defect. Thus, oxygenated blood is delivered into the right heart circulation to be returned to the lungs through the pulmonary artery. The oxygen saturation of the RA is increased and RA and right ventricular (RV) volume is increased. The amount of blood, which flows between the chambers, is dependent upon both the size of the defect and the pressure gradient between the chambers. A ratio of the pulmonary blood flow (Qp) to the systemic blood flow (Qs) less than 1.5 is often adequately tolerated; however, when the Qp/Qs ratio exceeds 3, symptoms secondary to overload of the pulmonary circulation appear.3 Over time, pulmonary artery hypertension can develop resulting in RV hypertrophy and failure. Eventually, right-sided pressures can exceed those of the left heart resulting in an Eisenmenger syndrome where deoxygenated venous blood flows in a right-to-left direction producing hypoxemia.
Over time many small defects of < 3 mm will close spontaneously; however, defects greater than 8 mm generally will not resolve spontaneously.4,5 Approximately 20% of ASDs will close during the first year of life and many go undetected even into adulthood. However, patients with ASDs are at risk for bacterial endocarditis and paradoxical right-to-left embolism and stroke. Larger ASDs often become manifest in the patient’s third decade heralded by complaints of dyspnea, arrhythmias, and congestive heart failure (CHF).3
The indications for surgical or catheter-mediated repair of an ASD include:
• RV volume overload
• Qp/Qs > 2
• Elective closure prior to entering school
Although some ASDs will close spontaneously, those children with sinus venosus and ostium primum ASDs have no chance of resolution without intervention.
Surgical closure of ASDs requires the use of cardiopulmonary bypass (CPB). Depending upon the size of the defect being repaired surgeons can perform either a primary suture closure of the defect or use a pericardial or synthetic patch.
Children taken to surgery for ASD repair are premedicated with oral or intravenous midazolam. Invasive arterial pressure monitoring is employed. Anesthesia induction can be through the use of inhalational agents or intravenous agents. The intravenous induction of anesthetic may be slower as the concentration of induction agent is diluted by flow from the left atrium. Conversely, the recirculation of vapor rich blood from the left heart to the pulmonary circulation will raise the partial pressure of inhalational agent in the lungs and thus in the brain, speeding induction. A reduced fraction of inspired oxygen (FiO2) is suggested for patient management following control of the airway to maintain pulmonary vascular resistance (PVR) and thus decrease left-to-right shunt. Maintenance of anesthesia in the pediatric population is with a combination of opioids, inhalational agents, and muscle relaxants. Prior to cannulation for the initiation of CPB, heparin is administered as in the adult population. Often CPB times are relatively brief and the patients are generally separated from CPB with little need for inotropic support. Following repair of the ASD, pressure within the LA will increase and right atrial pressure (CVP) will decrease secondary to elimination of left-to-right flow into the RA. A left atrial pressure line can be placed by the surgeon to discern left ventricular preload.3 Surgical mortality is low and hospital length of stay generally less than 4 days.
Many ASDs can be closed with devices delivered by a cardiac catheter. These procedures are performed under fluoroscopic and echocardiographic guidance and eliminate the need for sternotomy and CPB. Patient selection is essential to identify those most amenable to catheter-mediated repairs. Ostium primum and sinus venosus ASDs generally are not correctable by catheter-based approaches due to the location of the defect and associated pathologies. The Amplatzer septal occluder system (AGA Medical Corporation, Golden Valley, Minnesota) consists of two attached circular discs. The larger of the two discs faces the LA while the smaller disc faces the RA (Figure 12–2). The device straddles the defect in the atrial septum after being introduced via a catheter placed in a femoral vein. Over time, heart tissue overgrows the device incorporating it into the heart and occluding the ASD. The procedure is usually performed under general anesthesia. Complications are rare but include: air embolization, device dislodgement into the left atrium, and device impingement onto the tricuspid and mitral valve. An antithrombotic regimen is required for 6 months or longer to prevent clot formation on the surface of the device. Additionally antibiotic prophylaxis is necessary prior to certain surgical procedures in patients following device placement.
Figure 12–2. An Amplatzer septal occluder is positioned across an atrial septal defect.
VENTRICULAR SEPTAL DEFECTS
Ventricular septal defects (VSDs) are abnormal communications between the left and the right heart through the ventricular septum (Video 12–2 and 12–3). VSDs represent 20% of congenital heart defects.6 VSDs are classified by their location along the ventricular septum7:
• Type I: 5% to 7% of VSDs are Type I or sub-arterial VSDs. They are also known as supracristal, outlet, subpulmonary, infundibular, conal, and doubly committed. They are present near the aortic and pulmonic valve (Figure 12–3). They can cause herniation of the right coronary cusp of the aortic valve into the VSD resulting in aortic insufficiency.
Figure 12–3. Type I, supracristal VSD.
Figure 12–4. Type II, perimembranous VSD.
• Type II: Approximately 80% of VSDs are perimembranous VSDs. They are also known as infracrista, paramembranous, and conoventricular VSDs. The defect is in the membranous portion of the interventricular septum near the atrial ventricular valve (Figure 12–4). This defect is associated with septal malalignment, overriding aorta, subaortic stenosis, and tricuspid valve leaflet herniation into the septal defect.
• Type III: 5% to 8% of VSDs are inlet VSDs. These are also known as canal type VSDs or endocardial cushion defects. They are located inferior to the atrial ventricular (AV) valve.
• Type IV: 5% to 20% of VSDs are muscular VSDs and are found in the muscular part of the interventricular septum (Figure 12–5).
Figure 12–5. Type IV, muscular VSD.
Many VSDs are small and will close spontaneously. Spontaneous closure occurs most frequently during the first year of life. Some small, restrictive VSDs result in a minimal trans-septal blood flow and limited risk.8 Others result in free communications between the ventricles and the degree to which blood is shunted across the septum is dependent upon the ratio of systemic to pulmonary vascular resistance. When pulmonary vascular resistance is low, flow from the left heart into the right is increased. The result is volume overload and congestive heart failure. Over time, pulmonary vascular resistance increases secondary to development of pulmonary vascular occlusive disease leading to reversal of the shunt (flow becomes right-to-left) so-called Eisenmenger syndrome.7 Approximately 10% of patients with an uncorrected VSD will develop Eisenmenger syndrome, which carries a high incidence of early death.
Prevention of overcirculation in the pulmonary vasculature is essential to avoid pulmonary hypertension, right ventricular failure, and Eisenmenger syndrome. Initial anesthetic management for surgical closure of VSDs is similar to that described for ASD repair. However, nitric oxide (NO) along with infusions of the inotropic medications may be required to effectively separate from CPB. Conduction defects secondary to manipulation of the interventricular septum may occur necessitating temporary and at times permanent pacing following repair.
Defects in the atrioventricular canal or endocardial cushion can result in an ostium primum ASD, a cleft anterior leaflet of the mitral valve, and an inlet VSD. They represent approximately 2.9% of CHD patients. AV canal defects are associated with Down syndrome. Figure 12–6 demonstrates a so-called “partial” AV canal defect consisting of a primum ASD and a cleft mitral valve. Figure 12–7 reveals a complete AV canal defect with the addition of an inlet VSD. Patients develop an initial left-to-right shunt with associated congestive heart failure. If left untreated pulmonary hypertension develops much earlier leading to flow reversal from right to left. Surgical therapy often consists of not only closure of the atrial and ventricular septal defects but also correction of any abnormalities found in the patient’s atrioventricular valves. Inotropic support, NO therapy, and temporary pacing are often necessary to successfully separate the AV canal patient from CPB.
Figure 12–6. A partial AV canal with cleft mitral valve and ostium primum ASD.
Figure 12–7. Complete AV canal.
VSDs can occur acutely in the adult population following myocardial infarction or secondary to iatrogenic mishap (eg, too much myocardium resected during hypertrophic cardiomyopathy (HCM) surgery. Patients are often quite unstable and are taken to surgery for attempts at salvage.
TETRALOGY OF FALLOT
Tetralogy of Fallot (TOF) is the most common cyanotic heart disease representing 10% of all CHD patients (Video 12–4). TOF consists of four anatomic abnormalities:
1. Right ventricular outflow tract (RVOT) obstruction
2. RV hypertrophy
3. A large, unrestricted VSD
4. An aorta which overrides both the left and right ventricle receiving flow from both chambers (Figure 12–8).
Figure 12–8. Tetralogy of Fallot: VSD, overriding aorta, RV hypertrophy and right ventricular outflow obstruction.
Additionally, coronary artery abnormalities may present (5%-12%) with the left anterior descending coronary artery (LAD) branching from the right coronary artery (RCA). Other associated anomalies include: pulmonary valve atresia, ASD, persistent left superior vena cava, anomalous pulmonary vein connections, and dextrocardia.
The presentation of the TOF patient is dependent upon the degree of RVOT obstruction. Patients with significant RVOT obstruction will have a right-to-left shunt resulting in severe hypoxemia and cyanosis with saturations ranging from 70% to 80%. On the other hand, those with minimal obstruction “pink tet” will have VSD physiology with a left-to-right shunt. Often RVOT obstruction has a dynamic component leading to acute increases of obstruction and exacerbation of right-to-left shunting. Such episodes referred as “tet spells” may produce transient cerebral ischemia and loss of consciousness. These may occur secondary to infundibular spasm secondary to increased activity in the sympathetic nervous system as might occur with crying.9
Induction of anesthesia can produce a fall in systemic vascular resistance (SVR) leading to a worsening right-to-left shunt and hypoxemia. Should tet spells present in the perioperative period they can be relieved by:
• Hyperventilation with 100% FiO2
• Volume administration
• Phenylephrine
• Knee-chest position
• Increasing anesthetic depth
Ultimately, therapy is directed at increasing systemic vascular resistance, relieving infundibular spasm, and decreasing pulmonary vascular resistance.
The presence of chronic cyanosis can lead to polycythemia and the potential for embolic stroke across the VSD. Infectious vegetations are also a source of systemic emboli in TOF and other CHD patients at increased risk for endocarditis.
The majority of TOF patients are repaired in infancy except those whose pulmonary arteries are too underdeveloped.9,10 A palliative Blalock-Taussig (BT) shunt can be performed whereby the subclavian artery is anastomosed to the ipsi-lateral branch of the pulmonary artery (Figure 12–9). The BT shunt provides additional blood flow to the pulmonary vasculature to relieve cyanosis until a definitive repair can be completed. In those patients thought candidates for a complete surgical repair TOF surgery consists of:
• Ligation of any previous BT shunt
• Relief of RVOT obstruction through resection of excess muscle mass (Figure 12–10)
• Closure of the VSD
• Patch to enlarge subannular or transannular area of RVOT
• RV to PA conduit if pulmonary atresia or anomalous LAD are present
Perioperative anesthetic management of the TOF patient is centered upon promoting pulmonary blood flow and avoiding cyanosis. Dehydration should be avoided preoperatively. Oral midazolam can be given with the child appropriately monitored so to reduce perioperative anxiety, crying, and potentially the incidence of tet spells. An inhalational induction with sevoflurane is acceptable if intravenous access is not available. Nonetheless, limited pulmonary blood flow may slow an inhalational induction. The myocardial depressant effects of inhalational agents may be useful in limiting any infundibular spasm, RVOT obstruction, and right-to-left shunting across the VSD. On the other hand, systemic vasodilation and tachycardia caused by inhalational agents may increase right-to-left shunting and hypoxemia. If an IV is readily available etomidate, ketamine, and fentanyl used in combination with muscle relaxants and vasopressors can be employed. The presence of a right-to-left shunt may decrease the time for anesthetic effect following intravenous induction with some drug bypassing the lungs. Obviously, in any patient with right-to-left shunts in the heart all IV lines must be purged of air to prevent systemic air emboli perioperatively.
Systemic vascular resistance is maintained perioperatively with the use of phenylephrine and the patient’s volume augmented with fluid boluses. Inotropes generally are not used in the peri-induction period as they might worsen infundibular spasm and promote right-to-left shunting. Should infundibular spasm be suspected small doses of esmolol could be given.

Full access? Get Clinical Tree
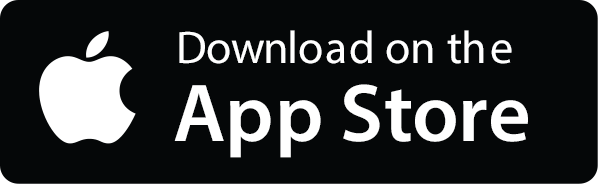
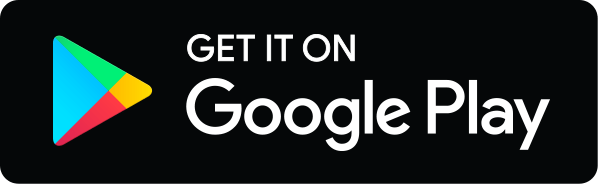