CHAPTER 26 Anesthesia for Orthopedic Surgery
The topic of anesthesia for pediatric orthopedic surgery encompasses the entire age and medical spectrum of pediatrics and includes the newborn and adolescent, the otherwise normal, the chronically ill, the patient with multiple complex congenital anomalies, the patient with emergent trauma, the elective inpatient, and the outpatient. Orthopedic surgeons operate on virtually every area of the body from the cervical spine to the pelvis to the toes. In many instances, the perioperative anesthesia plan for pediatric orthopedic patients depends more on their ages, on the site and emergent nature of surgery, and on the need for perioperative analgesia and sedation, than it does on the underlying disease or the specifics of the surgical procedure. In other cases, the underlying medical condition, associated anomalies, pathophysiology, and surgical procedure dictate the anesthesia plan. Positioning the patient on the operating room table may be difficult because of deformities or contractures, and often patients require special operating tables or frames to achieve the best posture for surgery. The anesthesiologist must be aware of unusual associated syndromes that have clear orthopedic implications, and syndromes with underlying clinical significance unrelated to the orthopedic condition. Conditions that are commonly encountered in pediatric orthopedic surgery and their anesthesia implications are listed in Table 26-1, but the list is by no means comprehensive (Campbell, 2009). The Online Mendelian Inheritance in Man website (www.ncbi.nlm.nih.gov/omim) is a good source for this information.
TABLE 26-1 Anesthetic Implications of Commonly Encountered Orthopedic Disorders
Disease | Surgical Interventions | Anesthetic Implications |
Congenital Malformations | ||
Amniotic band constriction | Soft tissue release | May have facial clefts |
Clubfoot | Tendon lengthening, release | Dictated by associated malformations |
Klippel-Feil syndrome | Release, scoliosis | Hemifused or fused vertebra; limited cervical spine mobility; possible difficult intubation; heart defects |
Radial dysgenesis | Tendon lengthening, pollicization release | Episodic thrombocytopenia; congenital heart disease |
Sprengel’s deformity | — | Associated only with Klippel-Feil syndrome |
Trisomy 21 (Down syndrome) | Cervical spine fusion | Large tongue; usually easy intubation; in-line stabilization during intubation; congenital heart disease; opioid sensitivity |
Acquired Conditions | ||
Charcot-Marie-Tooth disease | Tendon transfer | Possible sensitivity with nondepolarizing muscle relaxants; succinylcholine may result in hyperkalemia |
Legg-Calvé-Perthes disease | Osteotomies, pinning | None known |
Osteomyelitis | Culture, aspiration | Systemic bacterial infection |
Septic arthritis | Culture, irrigation | Systemic bacterial infection |
Slipped femoral capital epiphysis | Pinning | Obesity |
Tumors, benign | Excision, curettage | Possible significant blood loss; pathologic fracture |
Tumors, malignant | Radical excision, amputation | Blood loss; metastasis: CNS, lung; chemotherapy; cardiotoxicity |
Syndromes, Inherited Conditions | ||
Apert syndrome and Crouzon’s disease | Syndactyly repair; craniosynostosis; hypertelorism | Airway usually normal, but occasional mandibular hypoplasia; cardiac defect |
Ellis-van Creveld syndrome | Polydactyly | Cardiac defects; bronchial collapse |
Holt-Oram syndrome | Tendon lengthening, pollicization release | Cardiac defects (ASD, VSD) |
Jeune syndrome (asphyxiating thoracic dystrophy) | Chest reconstruction, scoliosis | Respiratory failure, prolonged mechanical ventilation; renal failure |
Marfan syndrome | Scoliosis | Cardiac (AI, MR), aortic aneurysm |
Moebius sequence | Syndactyly | Micrognathia; cleft palate; cranial nerve palsy |
Osteogenesis imperfecta | Pathologic fractures, scoliosis | Fractures on positioning or intubation; hypermetabolic fever, platelet dysfunction; blood pressure cuff may cause fractures |
VATER (vertebral, anal, tracheal esophageal fistula, renal, cardiac) | Tendon lengthening, pollicization release | Cardiac defects; tracheoesophageal fistula |
Short-Stature Syndrome | ||
Achondroplasia | Spinal fusion, cervical decompression, Ilizarov | Poor cervical mobility, difficult arterial catheterization |
Morquio-Ullrich disease | Cervical spine fusion | Poor cervical mobility, difficult airway |
Mucopolysaccharidoses (Hurler’s, Hunter’s, Morquio’s syndromes) | Kyphoscoliosis, bony abnormalities | Very difficult intubations; unstable necks; respiratory failure perioperatively |
Systemic Disease | ||
Juvenile rheumatoid arthritis | Varies | TMJ ankylosis; cervical spine immobility or instability; carditis; occasional pulmonary involvement; difficult airway |
Neurofibromatosis | Scoliosis | CNS tumors; occasional pheochromocytoma |
Sickle cell anemia | Osteomyelitis, Legg-Calvé-Perthes disease, pathologic fracture | Anemia; vasoocclusive crisis; acute chest syndrome; stroke; hypothermia; hypoxia; hypovolemia; immunocompromised host; avoid tourniquet when possible |
CNS Diseases | ||
Arthrogryposis multiplex | Tendon releases (multiple congenital contractures), scoliosis | Difficult intubation (TMJ ankylosis, cervical spine immobility); GE reflux; postoperative upper airway obstruction; congenital heart disease |
Cerebral palsy | Tendon releases | GE reflux; postoperative upper airway obstruction |
Myelomeningocele | Lower extremity tendon releases, scoliosis, kyphosis | Hydrocephalus |
Werdnig-Hoffmann disease | Scoliosis | Respiratory insufficiency; bulbar involvement (poor secretion handling); succinylcholine-induced hyperkalemia |
Myopathies | ||
Duchenne’s muscular dystrophy | Tendon releases, scoliosis | Respiratory insufficiency; cardiomyopathy; succinylcholine-induced hyperkalemia; anesthesia induced rhabdomyolysis |
Myotonia dystrophica | Tendon releases | Succinylcholine-induced myotonic spasm; cardiac conduction system involvement; avoid direct muscle stimulation |
AI, Aortic incompetence; ASD, atrial septal defect; CNS, central nervous system; GE, gastroesophageal; MR, mitral regurgitation; TMJ, temporomandibular joint; VSD, ventricular septal defect.
Scoliosis
Scoliosis, derived from the Greek root meaning “crooked,” is a lateral and rotational deformity of the thoracolumbar spine. With progression of the lateral spinal curvature, the spinous processes rotate toward the concave side of the curve. The ribs on the convex side are pushed posteriorly by the rotating spine, forming the characteristic gibbous deformity. The ribs on the concave side become prominent anteriorly and are crowded together. Occasionally, scoliosis is associated with kyphosis (“humpback”) or lordosis (“bent backward”) (Fig. 26-1).
The progression of scoliosis and the severity of its systemic manifestations correlate with the angle of curvature measured by the Cobb method (Table 26-2)—that is, the angle between the upper surface of the top vertebra and the lower surface of the bottom vertebra. The end vertebrae are those that are maximally tilted. Perpendicular lines are extended from these end vertebrae to the center of the curve. The angle formed by the intersecting perpendiculars determines the angle of curvature (Fig. 26-2). The curve is defined as facing to the right or to the left, depending on the convexity of the curve. A lateral curve of greater than 10 degrees is abnormal. Respiratory impairment rarely occurs with a curvature of less than 60 degrees.
TABLE 26-2 Correlation of Angle of Curve and Symptoms in Patients with Scoliosis
Angle of Curvature | Significance |
<10 | Normal |
>25 | Echocardiographic evidence of increased pulmonary artery pressures |
>40 | Surgical intervention |
>65 | Restrictive lung disease |
>100 | Symptomatic lung disease, dyspnea on exertion |
>120 | Alveolar hypoventilation |
Epidemiology and Etiology
The overall prevalence of spinal deformities in the North American population is between 1% and 2% (Weinstein et al., 2003). Curves can be described on the basis of their anatomic configurations, age of onset, and associated pathology. In the past, polio and tuberculosis infection were the most common causes. Today, most cases of scoliosis are classified as idiopathic because the basic pathophysiology is unknown. Pedigree analysis suggests that scoliosis is a sex-linked trait with variable expression and incomplete penetrance (Xiong and Sevastik, 1998; Lowe et al., 2000). The most common types of scoliosis are listed in Box 26-1.
Box 26-1 Classification of Scoliosis
Congenital scoliosis is a curvature of the spine that is the result of a rib or vertebral anomaly. Idiopathic scoliosis is the most common of the spinal deformities and has three periods of onset, all coincident with periods of rapid growth spurts: infantile (<3 years old), juvenile (3 to 10 years old), and adolescent (>10 years old). Progression of the deformity depends on the age of onset. Infantile idiopathic scoliosis is associated with an increased incidence of mental retardation, inguinal hernias, congenital dislocation of the hip, and congenital heart disease. Juvenile idiopathic scoliosis can usually be managed conservatively (Lowe et al., 2000). Adolescent idiopathic scoliosis is the most common form of scoliosis and occurs most commonly in girls (Weinstein et al., 2003). The curve may resolve, remain stable, or progress in severity. The most significant prognosticators of curve progression in girls are age at onset, premenarchal status, and bone age (Table 26-3) (Lowe et al., 2000; Ahn et al., 2002). Postulated mechanisms for the progression of adolescent idiopathic scoliosis include abnormal vertebral ossification, leptin-induced increased sympathetic nervous system activity, and increases in platelet-derived calmodulin (Lowe et al., 2004; Burwell et al., 2008; Burwell et al., 2009; Gu et al., 2009). Exotic scoliosis is a term introduced by Campbell and Smith (2007) to describe the spinal deformity found in rare pediatric conditions that pose special challenges beyond simply correcting the spinal curve. These very complicated patients with early-onset spinal deformity often tax the multisubspecialty resources of even the most sophisticated pediatric centers. Examples include patients with Jeune and Marfan syndromes.
TABLE 26-3 Incidence of Scoliotic Curve Progression at Time of Diagnosis of 10-Degree Curve
Age | Menarchal Status | Bone Maturity |
<11 yr (88%) | Premenarche (53%) | Immature (68%) |
>15 yr (29%) | Postmenarchal (11%) | Mature (18%) |
Natural History
The natural history of scoliosis varies according to the cause and the pattern of vertebral involvement. Uncorrected, scoliosis results in curve progression, cosmetic deformity, back pain, and physiologic compromise (Weinstein et al., 2003). In most cases of idiopathic scoliosis, the spinal curvature remains small, and conservative nonoperative management is appropriate (Richards et al., 1976). In 0.2% to 0.5% of cases, the curve increases, necessitating surgical intervention (Ahn et al., 2002). In patients with idiopathic scoliosis, only those with thoracic apices and curves of greater than 100 degrees are at increased risk for death from cor pulmonale and right ventricular failure (Asher and Burton, 2006). The grim prognosis of early death and respiratory failure is untrue in most patients with idiopathic scoliosis (Weinstein et al., 1981). The timing of surgery for this condition is controversial. The worse the curve and the more compromised the cardiorespiratory function, the greater the risk for perioperative morbidity and mortality. The pulmonary hypertension of progressive uncorrected idiopathic scoliosis often results in life-compromising respiratory failure in the fourth or fifth decade (Taylor and Gropper, 2006).
Respiratory Sequelae
Even asymptomatic scoliotic patients have demonstrable abnormalities in pulmonary function. As the degree of curvature progresses, vertebral rotation leads to thoracic cage asymmetry and deformation; lung volumes and pulmonary compliance are often but not always inversely related to the degree of this curve (Newton et al., 2005). When the scoliotic curve is greater than 65 degrees, pulmonary function tests demonstrate the characteristic pattern of restrictive lung disease. The first manifestation of this restrictive lung disease is a reduction in vital capacity; in many cases, the vital capacity (normal, 60 mL/kg) is severely reduced, often to less than 60% of predicted. Of the subdivisions of vital capacity, inspiratory capacity is affected to a greater extent than expiratory reserve volume. Functional residual capacity and residual volume are not as severely affected. These alterations in lung volumes are referable to the scoliotic changes in chest wall compliance and the resting position of the thoracic cage, rather than to parenchymal changes. The thoracic deformity of early-onset scoliosis damages pulmonary vascular development and inhibits physiologic alveolar growth, also producing decreases in pulmonary volumes (Charles et al., 1976; Fernandes and Weinstein, 2007).
The pulmonary impairment that results from the scoliosis of neuromuscular disease is exacerbated by coexisting abnormalities in central respiratory drive, coordination of swallowing, and innervation of the upper airway and respiratory musculature. Pulmonary dysfunction in these patients is exacerbated by an increased frequency of respiratory infections, a predilection to aspiration, and an impaired ability to clear pulmonary secretions. Patients who have abnormal results on their pulmonary function test, particularly a forced vital capacity (FVC) of less than 30%, or those who have hypercapnia preoperatively will probably require postoperative (or chronic) ventilation (Almenrader and Patel, 2006). Maximum inspiratory and expiratory mouth pressures (Pimax, Pemax) that the patient can generate against airway occlusion are the important indices for his or her ability to reexpand the lungs (sighs, Pimax ≤ 40 cm H2O) and to expel secretions (coughs, Pemax > 40 cm H2O). Unless the patient can generate more than these threshold pressures preoperatively, postoperative admission to the intensive care unit for ventilatory support should be anticipated.
Cardiovascular Sequelae
Mitral valve prolapse is found in 25% of patients with scoliosis but in less than 10% of age-matched controls. Echocardiographic evidence for increased pulmonary artery pressures has been demonstrated in individuals with only modest degrees of scoliosis in the absence of abnormal pulmonary function. Patients with angles of curvature greater than 70 degrees develop pulmonary hypertension on exercise; those with curves greater than 110 degrees have mean pulmonary artery hypertension at rest. Kafer (1980) proposed that this increase in pulmonary vascular resistance is not just the result of lung compression from thoracic cage abnormalities but is also the result of an increased incidence of hypoxic pulmonary vasoconstriction (Schur et al., 1984). In addition, development of the pulmonary vascular bed may be impaired, resulting in a fundamental reduction in the number of functional vascular units per lung (Kafer, 1980; Schur et al., 1984). Chronic hypoxia induces pulmonary vascular remodeling, which contributes significantly to the pulmonary hypertension in these patients (Morrell et al., 2009).
Any child with a myopathy or borderline respiratory status should have an electrocardiogram and an echocardiogram performed to assess the presence of cor pulmonale, ventricular wall motion, ejection fraction, and ventricular wall thickness. Many myopathies, particularly Duchenne’s muscular dystrophy, involve cardiac muscle and skeletal muscle (Manzur and Muntoni, 2009; Strehle, 2009). Duchenne’s muscular dystrophy is the most common muscular dystrophy in children who present for surgery. An X-linked recessive disorder, this progressive, debilitating disease affects skeletal, cardiac, and smooth muscle. Typically, afflicted boys become wheelchair dependent by the age of 10 years, and in the past, death from respiratory or cardiac failure occurred in this population before the age of 20. With the introduction of contemporary cardiopulmonary interventions, including noninvasive home ventilation, these men are living to previously unprecedented ages (Birnkrant et al., 2007; Birnkrant, 2009). Scoliosis is common, and surgery is often performed to improve the quality of life (see Chapter 3, Respiratory Physiology in Infants and Children).
Numerous anesthesia challenges are presented by patients with Duchenne’s muscular dystrophy. Clinically significant cardiomyopathies and rhythm disturbances manifest by 10 years of age (see Chapter 36, Systemic Disorders). Many of these children are obese because of muscle weakness, fatty degeneration of muscle fibers, and lack of exercise. Succinylcholine can cause a fatal hyperkalemia in these patients, who may present for surgery before the diagnosis has been definitively made; therefore, the routine use of this muscle relaxant is no longer recommended in all children (Birnkrant et al., 2007; Birnkrant, 2009; Gurnaney et al., 2009).
Preoperative Evaluation
The most important aspects of the preoperative evaluation include determination of the location and degree of spinal curvature, the cause of the scoliosis, the patient’s history of exercise tolerance, respiratory symptoms, and the presence of coexisting diseases. A directed physical examination of the cardiorespiratory system should evaluate the presence of tachypnea, crackles, wheezing, and signs of right heart failure, such as hepatomegaly, jugular venous distention, and peripheral edema. Any preoperative neurologic deficits should be recorded. Based on the severity of the curve and the degree of respiratory impairment, the preoperative laboratory studies listed in Box 26-2 should be requested.
Children with myelodysplastic syndromes are likely to develop an allergy to latex products (Kelly et al., 1994; Maxwell, 2004; Dewachter et al., 2009). All children with any of these syndromes should be considered allergic to latex, and nonlatex products (e.g., tourniquets, sterile and nonsterile gloves) should be substituted for the latex equivalents. Corticosteroids and antihistamines are not administered prophylactically.
Surgical Techniques
The treatment of spinal curvature is dictated by the type of scoliosis and by the surgeon’s expertise and preferences. Very few cases of congenital scoliosis can be managed conservatively. The mainstay of therapy is posterior spinal fusion without instrumentation, followed by prolonged immobilization. Instrumentation in these patients has been associated with a prohibitively high rate of paraplegia, which is presumed to be the result of coexisting cord and vertebral anomalies. Although conservative therapy is the most frequently used treatment for idiopathic scoliosis, when rapid curve progression is anticipated, surgical intervention is used for severe truncal deformities and for pain unrelieved by medical therapy (Weinstein et al., 2003).
Posterior Spinal Fusion
Several instrumentation techniques are available for treatment of the scoliotic spine. The Harrington rod, the original instrumentation system, consists of a stainless steel rod that is connected to the inferior facets and pedicles of the spine by multiple ratchet hooks placed at the terminal aspects of the curve. Distraction is adjusted using the ratchet principle (Harrington and Dickson, 1976; Harrington, 1988). The incidence of neurologic complications after this technique is 0.23%. The disadvantages of the Harrington rod include two-dimensional correction, curvature distraction by the end hooks, and the need for prolonged postoperative immobilization. Because of these problems, this technique is rarely used.
Segmental spinal instrumentation was introduced to provide three-dimensional correction and to achieve differential distraction at multiple levels. The Luque instrumentation system consists of sublaminar wires on each side of the spinous process and a long, L-shaped rod that can be contoured three dimensionally. The curve is corrected as the wires are tightened (Luque, 1986; Luque and Rapp, 1988). The internal fixation achieved is more rigid than that obtained with the Harrington system, and it can be extended to the pelvis. The most common deficit after Luque rod instrumentation is a dysesthesia, which is usually observed late (2 to 6 days) in the postoperative period. The proposed mechanism for these findings is expansion of an epidural hematoma in the area of the sublaminar wires (Johnston et al., 1986).
The Cotrel-Dubousset segmental spinal instrumentation system uses multiple laminar and pedicular hooks attached to a double-rod frame (Richards and Johnston, 1987). This system enables three-dimensional correction of complex curves and obviates the need for postoperative immobilization. It is more time consuming than the Harrington system, increases intraoperative blood loss, and has a lower incidence (0.6%) of neurologic complications than Luque rods. Double-curve patterns are more complex and require multiple hooks at multiple fixation sites, necessitating more extensive decortication and contributing to additional blood loss.
Pedicle screws are the most recent advance in posterior spinal fusion (Kim and Noonan, 2009). Initially used for lumbar curves, they are now used for total curve correction. The limitation of posterior spinal fusion with or without instrumentation is that the anterior growth plates, which play a major role in the development of the deformity, are not affected. Late torsional deformities can result.
Anterior Spinal Surgery
The anterior approach to spinal deformities has been advocated for several specific deformities, including severe kyphosis and lordotic paralytic curves in patients with cerebral palsy. Surgery consists of discectomies with or without instrumentation, performed alone or in combination with a posterior spinal fusion. Video-assisted thoracoscopic surgery can be used for this procedure if instrumentation is not being used (Sucato, 2003). The surgical approach used to expose the anterior portion of the spine depends on the exact spinal deformity. Thoracic curves are usually approached through a left thoracotomy, and the procedure is facilitated by insertion of a double-lumen endotracheal tube and one-lung ventilation. Alternatively, single-lung ventilation in young children is performed by advancing a tracheal tube into the main stem bronchus opposite the side of surgery, or by positioning a bronchial blocker into the main stem bronchus on the operative side.
Many techniques for placing a variety of bronchial blockers outside the tracheal tube have been described for use in children (see Chapter 23, Anesthesia for General Abdominal, Thoracic, Urologic, and Bariatric Surgery) (Hammer, 2001, 2004; Hammer et al., 2002). The combined curve of the thoracolumbar spine is exposed transdiaphragmatically by means of a high subcostal incision that necessitates taking the diaphragm down from its bony insertion. Lumbar curves can be approached extraperitoneally or transabdominally. In general, complications of the anterior approach include great vessel disruption, hemothorax, pneumothorax, paralytic interruption of spinal cord perfusion, and excessive angulation or compression of the spinal cord by rapid distraction of the curvature. Spinal cord injury can result from mechanical damage by a screw or disruption of segmental spinal arteries. At the beginning of the 21st century, performance of anterior scoliosis surgery diminished because of the corrective power of pedicle screw constructs and better surgical methods designed to improve flexibility, such as posterior release, posterior osteotomy, and vertebral resection (Kim and Noonan, 2009).
As an alternative to open thoracotomy, a video-assisted thoracoscopic surgery (VATS) approach is being used to perform anterior thoracic spine release in patients who require both anterior and posterior procedures to correct their scoliosis. The appropriate use of pedicle screws has resulted in a 50% to 66% curve correction, with good maintenance of the curve correction for a minimum of 3 years (Lehman et al., 1976).
Anesthesia Management of Scoliosis Surgery
Monitoring and Intraoperative Complications
Scoliosis surgery is very high-risk surgery. Common intraoperative problems are listed in Table 26-4. Complications are related to the surgery (blood loss and its therapy), the prone position, and cardiovascular collapse from myriad causes. Practiced crisis management is essential if patient survival is to be ensured.
TABLE 26-4 Potential Intraoperative Complications During Scoliosis Surgery
Problem | Monitoring Solution |
Endotracheal tube malposition | Securely tape tube before turning Benzoin Waterproof tape Bite block (rolled 4×4 gauze pads) After turning prone Hand ventilate and listen to both lung fields DO NOT ALLOW STRETCHER TO LEAVE THE OPERATING ROOM until you are satisfied that the endotracheal tube has not migrated Hourly arterial blood gas determinations Esophageal stethoscope |
Alteration in pulmonary compliance in the prone position | Proper position on bed frame: ensure chest can expand unimpeded Hourly arterial blood gas determinations |
Alteration in cardiac function in the prone position | Proper position on bed frame to ensure that venous return is not compromised Indwelling arterial catheter Central venous catheter Bladder catheter |
Hypotension | Blood loss until proven otherwise Ensure that typed and cross-matched blood is available when surgery starts. Two large-bore peripheral IV catheters and blood warmer Central venous catheter Hourly measurement of hemoglobin/hematocrit Consider Amicar |
Coagulopathy | Platelet count Prothrombin time, activated partial thromboplastin time, fibrin split products Thromboelastogram |
Electrolyte abnormalities (usually from blood transfusions) | Frequent measurements of sodium, potassium, and ionized calcium Avoid using “old” packed red blood cells NEVER use hypotonic solutions (including for maintenance fluid requirements) |
Excessive heat loss | Measure core temperature Heat conservation Active warming (forced air) |
Neurologic injuries | Proper positioning, with particular attention to eyes and elbows (brachial plexus) Intraoperative assessment of cord function (e.g., sensory and motor evoked potentials) |
Neurologic Monitoring
Postoperative paralysis or sensory loss is the most feared, devastating, and often unpredictable complication of scoliosis surgery (Owen, 1999). Neurologic injury may result from direct injury to the spinal cord or nerves during instrumentation, from excessive traction during distraction, or from compromised perfusion of the spinal cord. Because the ramifications associated with motor deficit are significantly greater than those associated with sensory deficit, surgically induced paraplegia has always been the major concern of scoliosis surgery.
Spinal Cord Blood Flow
The organization of the spinal cord blood supply is segmental in a cross-sectional and rostral-caudal fashion (Fig. 26-3). The intrinsic spinal cord vasculature consists of the anterior median and the paired posterior spinal arteries. The vasculature supplying these vessels arises from the segmental arteries of the aorta and branches of the subclavian—the vertebral arteries—and the internal iliac arteries. The solitary anterior median spinal artery runs along the entire length of the cord in the anterior sulcus, giving off penetrating branches that supply the ventral two thirds of the spinal cord. Blood flow in the anterior spinal artery is not continuous throughout its span; instead, the anterior spinal artery functions as an anastomotic channel between the terminal branches of successive radicular arteries. Blood that leaves the terminal aspects of these radicular arteries courses upward and downward in the anterior spinal artery. At points between adjacent radicular arteries, blood flows in either direction. The paired posterior spinal arteries, which supply the dorsal third of the cord, also have discontinuous segments and appear more like a plexus of pial vessels than paired arteries.
The regional circulation of the spinal cord is divided into four segments. The cervical and lumbosacral regions each receive double the blood flow received by the thoracic region (see Fig. 26-3). Although each vertebral level has paired segmental arteries, only six to eight important medullary arteries are formed. These medullary arteries join the spinal arteries. The segmental arteries at all other levels are functionally nonsuppliers of blood to the spinal cord itself. The vertebral arteries form the rostral origins of the anterior and posterior spinal arteries and represent the principal supply to the cervical cord. Branches of the thyrocervical and costovertebral arteries supply the lower cervical and upper thoracic cord. A radicular artery arising from T7 provides perfusion for the middle thoracic cord. The most consistent and important of the anterior medullary arteries is the artery of Adamkiewicz—the arteria radicularis magna—which usually joins the anterior spinal artery between T8 and L3. This artery is the predominant source of blood supply to the lower two thirds of the spinal cord. The implications of this design dictate the clinical manifestations of impaired cord perfusion. Watershed areas, which are subject to ischemia during low-flow states, exist between the anterior and posterior circulations and between the four different spinal segments. The segments of T4 to T7 appear to be highly susceptible to injury during periods of hypoperfusion. The dependence of the lower two thirds of the cord on the artery of Adamkiewicz puts this region at particular risk during surgical manipulation of the thoracolumbar aorta and spinal column, and this is referred to as the lumbar artery enlargement syndrome. Although the clinical picture of this syndrome is not constant, it is marked by the development of flaccid paraplegia or quadriplegia (depending on the level of the lesion) and dissociated sensory impairment in which heat and pain sensations are affected but deep sensation is spared.
The same principles that regulate the cerebral blood flow apply to spinal cord blood flow. Thus, cord blood flow depends on the perfusion pressure (i.e., mean arterial pressure [MAP] minus cerebrospinal fluid pressure), integrity of the circulation, microcirculatory autoregulation, and intrinsic regulation. If the perfusion pressure falls below 50 mm Hg, spinal cord blood flow is reduced. Spinal cord blood flow autoregulates within the range of a MAP of 60 to 150 mm Hg. Spinal cord blood flow is also regulated on an intrinsic basis in response to arterial oxygen and carbon dioxide tensions, pH, and cord temperature in a fashion identical to that of the cerebral circulation. Hypercapnia increases flow, whereas a Pao2 below 60 mm Hg results in a vasodilation that overrides the effects of hypocarbia and autoregulation (see Chapter 22, Anesthesia for Neurosurgery).
Minimizing Postoperative Neurologic Complications
The estimated risk for postoperative neurologic injury in patients undergoing spinal instrumentation is 0.72% to 1.6% (Cervellati et al., 1996). In a study of 7885 patients who underwent instrumentation or fusion without instrumentation, 87 patients developed acute neurologic changes, and 36% of these patients recovered without sequelae. Individuals with nonidiopathic scoliosis are at higher risk for neurologic injury. Children with congenital scoliosis suffer neurologic complications disproportionately (Cervellati et al., 1996).
Wake-Up Test
Vauzelle and colleagues (1973) first described the use of the wake-up test to assess the integrity of the spinal cord. In this technique, patients are awakened intraoperatively to assess spinal cord motor function. The wake-up test requires an anesthetic that allows rapid recovery of consciousness and motor function. Ideally, the test is rehearsed preoperatively. During rehearsal, patients are informed that they will be momentarily awakened at the time of rod insertion to test the function of the spinal cord. Patients must be reassured that they will neither remember the event nor experience pain while they are “awake.” Preoperative preparation increases the speed and success of the test. The effectiveness of neurophysiologic monitoring during spinal deformity surgery is now so well established that the wake-up test is rarely used except to confirm monitoring changes (Schwartz et al., 2007).
When a wake-up test is performed, the operating room must be quiet, the surgeon must stop operating, and an observer is positioned (usually under the drapes) to look for foot movement. After discontinuation of the anesthetic, the patient is first asked to move the hands (“squeeze my fingers”) to evaluate the level of consciousness and is then asked to move the feet (“wiggle your toes”). If the patient is unable to move the feet but can move the hands, spinal cord compromise is presumed, and the spinal rod instrumentation is removed immediately. Spinal cord perfusion is maximized by raising the MAP, increasing the hemoglobin concentration, and normalizing arterial carbon dioxide and oxygen tensions. In one series of 166 patients in whom the wake-up test was used, three patients had demonstrable neurologic deficits when awakened (Hall et al., 1978). These deficits disappeared immediately on release of the distracting force (i.e., rods) (Hall et al., 1978).
Neurometric Monitoring
Sensory-Evoked Potentials
Electrophysiologic (neurometric) monitoring provides real-time, continuous assessment of spinal cord function and does not require patient movement, arousal, or cooperation (see Chapter 11, Monitoring) (Gonzalez et al., 2009). The most common technique uses somatosensory evoked potentials (SSEPs), in which the cortical and subcortical responses to peripheral nerve stimulation are monitored (Banoub et al., 2003). Typically, a peripheral mixed nerve (i.e., posterior tibial nerve, peroneal nerve, or median nerve) is stimulated at fixed intervals during a procedure. SSEPs are recorded repeatedly during surgery, and their amplitude (height) and latency (time of occurrence) are compared with baseline values (Gonzalez et al., 2009; Mendiratta and Emerson, 2009). Based on changes in these characteristics, it is possible to determine the functional status of the spinal cord sensory tracts. SSEP monitoring requires specialized technology and expertise. To resolve the very low amplitude evoked potentials from background, random, or spontaneous cortical activity, computer signal averaging of repetitive sensory responses is required. The processed evoked-potential waveform is plotted as voltage against time and is characterized by the poststimulus latency and amplitude. The poststimulus latency reflects the time required for impulse transmission from the site of sensory stimulation. A reduction in amplitude of greater than 50% or an increase in latency of less than 10% relative to baseline values is generally considered significant (Banoub et al., 2003; Gonzalez et al., 2009; Mendiratta and Emerson, 2009).
SSEPs monitor only the dorsal columns of the spinal cord and provide no direct evidence of loss of motor function or anterior spinal cord injury. Motor deficits may occur in the absence of alterations in SSEPs, and numerous case reports have recorded the postoperative finding of paralysis despite unchanged intraoperative SSEPs (i.e., false-negative results). In the setting of spinal cord ischemia, the time to loss of SSEPs was almost three times longer than the time to motor evoked potential (MEP) loss (Banoub et al., 2003; Shine et al., 2008). The most comprehensive information regarding the false-negative rate of SSEPs comes from a survey of spine surgeons by the Scoliosis Research Society and the European Spinal Deformity Society, in which definite neurologic deficits, despite stable SSEPs, occurred during surgery in 0.063% of patients (Nuwer et al., 1995). Children with neuromuscular scoliosis frequently have unreliable SSEP data. Despite these limitations, SSEP monitoring reduces postoperative paraplegia by more than 50% (Nuwer et al., 1995). When SSEP monitoring is equivocal, many recommend an intraoperative wake-up test to assess motor function (Grundy, 1983).
Many pharmacologic and physiologic variables affect the latency and amplitude of SSEPs and have been estimated to account for up to 44% of intraoperative SSEP changes. The most important of these are the anesthesia agents, blood pressure, and body temperature (Table 26-5) (Grundy, 1983; Banoub et al., 2003; Gonzalez et al., 2009). All of the potent inhaled anesthesia agents produce dosage-dependent increases in latency and decreases in amplitude. These effects are less for sevoflurane and desflurane, permitting dosages of 1.5 minimum alveolar concentration (MAC) with minimal SSEP changes. Nitrous oxide compounds the effects of volatile anesthetics on cortical SSEPs (Banoub et al., 2003). Alone, nitrous oxide has no effect on SSEP latency but does decrease its amplitude by 50% (Schwartz et al., 2007). Substantial recovery of latency and amplitude is achievable with discontinuance of nitrous oxide and the inhaled vapors (da Costa et al., 2001). In general, the IV agents affect SSEP less than inhaled agents do; at high dosages, they produce slight to moderate decreases in amplitude and increases in latency. Midazolam has no effect on latency (Laureau et al., 1999; Banoub et al., 2003). Ketamine (Langeron et al., 1997) and etomidate (Thakor et al., 1991) augment SSEP amplitude. Propofol has no effect on amplitude or latency and is highly recommended as a component of total IV anesthesia for scoliosis surgery (Laureau et al., 1999; Boisseau et al., 2002). Opioids given in either analgesic or anesthetic dosages produce minimal SSEP effects (Banoub et al., 2003). In a very small study, dexmedetomidine had no effect on SSEP or MEP (Tobias et al., 2008).
TABLE 26-5 Effects of Anesthesia Agents on Somatosensory Evoked Potentials
Agent | Amplitude | Latency |
Desflurane | ↓ | ↑ |
Isoflurane | ↓ | ↑ |
Sevoflurane | ↓ | ↑ |
Nitrous oxide | ↓ | ↔ |
Barbiturates | ↓ | ↑ |
Etomidate | ↑ | ↔ |
Ketamine | ↑ | ↔ |
Midazolam | ↓ | ↔ |
Opioids | ↔ | ↔ |
Propofol | ↔ | ↔ |
Dexmedetomidine | ↔ | ↔ |
↓, Decreases; ↑, increases; ↔, remains the same.
The amplitude and latency of the waveform are also affected by age, preexisting neurologic deficits, body temperature, Paco2, hypoxia, and blood pressure (Fig. 26-4) (Banoub et al., 2003). The reliability of spinal cord monitoring may be dramatically affected by the variability of the evoked responses. Muscle relaxants have no direct deleterious effects on the SSEP but may produce a more reliable recording by providing “quieter” conditions.
An anesthesia milieu that is compatible with adequate neurometric monitoring and that allows rapid awakening can be created using a variety of approaches. Combining desflurane or sevoflurane with a remifentanil infusion produces ideal SSEPs and still allows for rapid wake-up if a wake-up test is required. Alternatively, the physician can substitute a continuous propofol infusion for the potent inhaled anesthetics in combination with an opioid (Kalkman et al., 1991a; Kalkman et al., 1991b). Because etomidate augments SSEP amplitude, it is particularly useful in patients with abnormal preoperative SSEPs. These individuals are at greatest risk for the development of postoperative neurologic catastrophes (Sloan et al., 1988; Samra and Sorkin, 1991).
Using the criterion of a decrease in amplitude of greater than 40% amplitude as a significant change, excellent specificity and sensitivity are achievable. In patients with idiopathic scoliosis (i.e., neurologically intact), SSEPs are reliable and can be obtained in more than 98% of patients (Padberg et al., 1998). However, in patients with preexisting diseases such as neuromuscular scoliosis, the reliability of the SSEP is less than 75% but can be improved with the addition of MEP monitoring (Sarwark and Sarwahi, 2007). Precise communication and coordination of efforts among the surgeon, anesthesiologist, and neurometric specialist are imperative when a change in SSEP is observed. Normalization of the SSEPs may occur spontaneously with relaxation of the distraction instrumentation or by improving spinal cord perfusion (e.g., increasing blood pressure and Paco2 levels).
Motor Evoked Potentials
SSEPs are not the modality of choice for monitoring motor tract function or for detecting the presence of a surgically induced motor deficit. The motor pathways can be activated by transcranial stimulation of the motor cortex or by spinal cord stimulation. Transcranial MEPs (TcMEPs) are exquisitely sensitive to compromised spinal cord function and should be used in combination with SSEPs to improve the accuracy of spinal cord monitoring. In a series of 1121 adolescents with idiopathic scoliosis, nine children awoke with a postoperative neurologic deficit, seven had motor or sensorimotor deficit, and two had pure sensory impairment. All seven patients with motor impairments had significant TcMEP changes; only three of the seven had significant SSEP changes. When changes were detected by both modalities, SSEPs lagged behind TcMEPs by approximately 5 minutes (Schwartz et al., 2007). As a result, some centers have abandoned using SSEPs entirely. Finally, the false-positive rate attributed to MEP monitoring during spinal surgery is less than 10% (Langeloo et al., 2003).
Anesthesia agents have profound effects on MEP recordings. Volatile anesthetics, benzodiazepines, etomidate, barbiturates, and even high-dosage propofol affect MEP fidelity (Sloan and Heyer, 2002; Sloan et al., 2008). A maintenance anesthesia that is based on a continuous infusion of propofol and remifentanil is titrated to maintain a MAP of at least 65 mm Hg, and that avoids nitrous oxide and muscle relaxants, provides adequate conditions for uncompromised TcMEP monitoring. Alternatively, high-dosage remifentanil in combination with low-dosage sevoflurane (0.4 to 0.7 MAC), or continuous infusions of propofol, fentanyl, and dexmedetomidine, are also regimens compatible with reliable monitoring (Joseph et al., 1976; Langeloo et al., 2003, 2007; Anschel et al., 2008).
Blood Loss
Exposure of a vast area of decorticated, raw, cancellous bone during spinal surgery results in extensive blood loss that can exceed 25 mL/kg, even in uncomplicated surgery. Children with neuromuscular scoliosis often require more extensive procedures, including pelvic stabilization, than children with idiopathic scoliosis and can easily have blood loss that exceeds a blood volume (Kannan et al., 2002; Meert et al., 2002). However, even if the extent of surgery is controlled, patients with neuromuscular diseases have an almost seven times higher risk for losing greater than 50% of their estimated total blood volume during scoliosis surgery than do normal controls (Edler et al., 2003).
An estimation of circulating blood volume should be made before the induction of anesthesia. The estimated blood volume (EBV) is calculated by multiplying the patient’s weight by the approximate blood volume based on age (Table 26-6). From the EBV, the initial hematocrit, and the minimum acceptable hematocrit, the maximum allowable blood loss can be estimated before packed red blood cell transfusion is indicated.
TABLE 26-6 Approximate Blood Volume Based on Age
Age | Total Blood Volume (mL/kg) |
Premature infant | 90-105 |
Term newborn | 80-85 |
1 to 12 months | 70-80 |
Older child | 70-80 |
Adult | 55-65 |
Multiple strategies have been described to reduce intraoperative blood loss and the need for blood transfusions of homologous blood in the perioperative period (see Chapter 14, Blood Conservation). Techniques that may decrease intraoperative blood loss include induced hypotension, alteration of the operative position, changes in surgical technique, and administration of antifibrinolytic agents such as ε-aminocaproic acid (Amicar) or tranexamic acid (Cyklokapron). Techniques aimed at decreasing the use of homologous blood products include intraoperative blood salvage, preoperative autologous blood donation, and perioperative normovolemic hemodilution and apheresis (Joseph et al., 1976). Each of these techniques has demonstrated efficacy in some clinical situations.
Preoperative autologous donation with and without erythropoietin treatment has been used extensively in children (Helfaer et al., 1998; Vitale et al., 1998). As this has become more widespread for pediatric scoliosis, 70% of children and adolescents have been able to donate sufficient units for their procedures, a figure similar to that for adults (Murray et al., 1997). Using preoperative autologous donation as the sole blood conservation method limited the number of adolescents undergoing scoliosis surgery who required allogeneic transfusions to 11% to 23%. The addition of autologous donation to deliberate hypotension further reduced allogeneic exposure to 10% (Murray et al., 1997).
Alternatively, perioperative hemodilution and apheresis, isovolemic hemodilution, or acute normovolemic hemodilution can substitute for or augment autologous blood donation (Bryson et al., 1998; Copley et al., 1999). Before incision, the patient’s blood is withdrawn through a large-bore peripheral IV cannula or through a central venous catheter and replaced with an isotonic balanced salt solution or colloid in a ratio of 3:1. When using this technique, cardiac index and oxygen extraction increase, and systemic vascular resistance, oxygen delivery, and mixed venous saturation decrease. Lactic acidosis develops when oxygen delivery falls to critical. Moderate acute normovolemic hemodilution, with a minimum hematocrit of 17%, did not result in changes in either oxygen delivery or oxygen consumption in healthy children. The removed blood is stored in anticoagulated bags and is administered as needed to maintain the hematocrit at a predetermined level, usually greater than 20%. Complications of this technique include postoperative pulmonary edema, anasarca, and prolonged postoperative mechanical ventilation.
Perioperative salvage of blood (i.e., use of a cell-saver system) allows blood lost in the operative field to be returned to the patient during the perioperative period (Huet et al., 1999). The efficiency of this technique, its benefits in limiting blood transfusion, and the survivability of red blood cells are unclear (Vitale et al., 2002). When using salvaged blood, it is imperative that the blood be processed and washed adequately to avoid life-threatening bleeding and pulmonary complications caused by transfusion of cellular and tissue debris. Salvaged blood can also cause profound hypotension (e.g., citrate, air embolism) and hemolytic and bleeding complications from centrifugation, cellular debris, or anticoagulant overdose.
Pharmacologic manipulation of the hemostatic mechanism is effective in limiting perioperative blood loss. A series of prospective, randomized, double-blind studies demonstrated that the antifibrinolytic agent ε-aminocaproic acid significantly reduces perioperative blood loss and transfusion requirements in patients with either idiopathic or neuromuscular scoliosis who are undergoing spinal fusion (Florentino-Pineda et al., 1976; Thompson et al., 1976a, 1976b

Full access? Get Clinical Tree
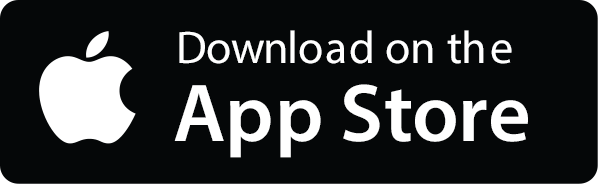
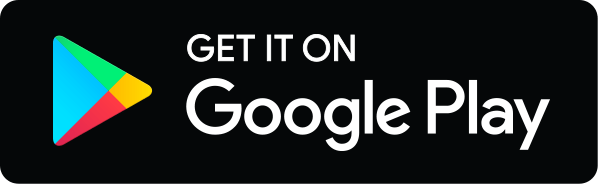