Key Points
- ▪
Ophthalmic procedures are considered to be “low-risk.” However, the patient population is higher risk because of the extremes of age involved and associated comorbidities.
- ▪
Some ophthalmic surgeries (cataract, glaucoma, simple vitrectomy) are short procedures, but high in volume. The demand for efficiency while maintaining patient safety is a challenge.
- ▪
The majority of ophthalmic procedures can be performed under topical anesthesia or orbital block in combination with monitored anesthesia care (MAC).
- ▪
A working knowledge of the anatomy and physiology of the eye is essential in providing safe anesthesia care. This includes the effect of anesthetic drugs and interventions on intraocular pressure and systemic effects of ophthalmologic medications.
- ▪
Orbital blocks, particularly retrobulbar blocks, can have severe complications, including retrobulbar hemorrhage, and retrograde spread of local anesthetic into the subarachnoid space causing brainstem anesthesia, loss of consciousness, and respiratory arrest.
- ▪
Routine preoperative laboratory testing is not necessary for cataract surgery and has not been shown to reduce adverse perioperative events.
- ▪
General anesthesia is required in 30% to 40% of ophthalmic surgeries. This includes pediatric ophthalmic surgery; complex procedures, some of which require muscle relaxation; and adults who cannot undergo MAC for a variety of reasons.
- ▪
Strabismus surgery is an independent risk factor for postoperative vomiting in pediatric patients.
- ▪
Succinylcholine can be used in unfasted patients who have an open-globe injury that requires emergent vision-saving surgery under general anesthesia.
- ▪
Prevention of prolonged postoperative anesthesia care unit stay and unanticipated hospital admission requires careful patient evaluation, optimization of underlying medical conditions, adequate pain control, prevention of postoperative nausea and vomiting, and maintenance of hemodynamic stability.
Acknowledgment
The editors and publisher thank Dr. Marc Allan Feldman for contributing a chapter on this topic in the prior edition of this work. It has served as the foundation for the current chapter.
Overview of Ophthalmic Anesthesia
Vision is one of the most important functions of the human body. Visual impairment and blindness limit a person’s ability to function normally in daily living, has a large economic impact in the United States, and results in significant loss of quality-adjusted life years. Currently, about 4.2 million adults in the United States are visually impaired. Early diagnosis and treatment, including surgery, can potentially reverse vision impairment, such as in cataract extraction, or delay and attenuate the pathophysiological process, as in glaucoma and diabetic retinopathy. Anesthesia providers specialized in ophthalmic anesthesia play an important role in helping patients undergo surgery comfortably and safely.
Ophthalmic surgeries are the most common operations performed in the elderly. They consist of a broad spectrum of procedures, ranging from cataract surgery with minimal sedation to more complex procedures such as orbital decompression, or combined corneal transplantation and retinal surgery that requires general anesthesia. The majority of ophthalmic surgeries are short in duration and primarily performed in ambulatory surgical centers. Patients who undergo ophthalmic surgery can be as young as preterm infants to nonagenarians. Ophthalmic procedures are generally considered low risk because they do not cause significant physiological perturbations, and do not involve a large volume of blood loss or significant postoperative pain. However, ophthalmic surgery is often carried out in elderly patients who have a high prevalence of coexisting illnesses such as diabetes, hypertension, coronary artery disease, and chronic obstructive pulmonary disease. Adequate preoperative evaluation and optimization are important in the prevention of same day cancellation and unplanned hospital admission.
Patient safety is paramount in ophthalmic anesthesia. In the American Society of Anesthesiologists (ASA) Closed Claims Project from 1980 to 2000, the identifiable causes of severe eye injury include intraoperative patient movement, either under general anesthesia or monitored anesthesia care (MAC), and needle trauma related to an orbital block with or without patient movement. In an ASA closed claims analysis of injury and liability associated with MAC for ophthalmic and nonophthalmic surgeries, the major mechanisms of injury included inadequate oxygenation or ventilation, cardiovascular event, equipment failure, inadequate anesthesia leading to patient movement, and oversedation leading to death or permanent brain damage. Presently, there is little data available regarding postoperative complications associated with ophthalmic anesthesia. A retrospective study of prolonged postanesthesia care unit (PACU) stay following ophthalmic surgery found the incidence was about 0.6%, with the major factors being hypotension, bradycardia, postoperative nausea and vomiting (PONV), and oversedation. A similar study found new onset cardiac dysrhythmia, pain, PONV, and pulmonary-related events (hypoxia and pulmonary aspiration) were the main reasons for unintended hospital admission with an incidence of 0.23%. Overall, ophthalmic surgery is safe with a low incidence of anesthesia-related complications.
Fast-track surgery has been successfully implemented in ophthalmic surgery to facilitate early discharge home and to allow patients to resume normal activities of daily living. Each patient’s anesthetic plan should be personalized based on the type, duration, invasiveness of surgery, underlying comorbidities, degree of anxiety, expectation of anesthesia, and history of anesthetic complications with the goal of “fast-tracking” and minimizing potential adverse events postoperatively. Perioperative surgical home is a patient-centric, team-based model of care to improve population health, reduce costs, and satisfy patients. It can improve outcome and may be a useful model in ambulatory surgery settings, including ophthalmic surgery.
Patient satisfaction has become a standard indicator of value in health care, including anesthesia care. For ophthalmic surgery, information, communication, pain control, comfort, and support are the most important predictors for patient satisfaction in the perioperative period.
Ocular Anatomy
The eye is a delicate and complex organ that allows us to see images, color, and movement, and helps with depth perception and balance. The eye is a sphere measuring approximately 24 mm in diameter. It is situated in the pyramidal bony orbit. The wall of the globe has three layers: the sclera, the uveal tract, and the retina. Fig. 69.1 shows the individual components of the eye.

The sclera is the outermost layer. It is the tough, fibrous white of the eye, and it is continuous with the cornea anteriorly. The tissue where the cornea and sclera meet is the limbus, which contains stem cells responsible for regeneration of the epithelium. About 60% of the focus power of the eye is from the curvature of the cornea.
The middle layer, the uveal tract, has three structures: the choroid, the iris, and the ciliary body. The choroid is a layer of blood vessels located posteriorly. Bleeding in this layer is one cause of intraoperative expulsive hemorrhage. The pigmented iris controls light entry with muscle fibers that change the size of the pupil. Sympathetic stimulation dilates the pupil by causing iris dilator muscles to contract, whereas parasympathetic stimulation causes meiosis, or pupillary constriction by causing the iris sphincter muscles to contract. The ciliary body lies just behind the iris; it produces aqueous humor. Ciliary muscle fibers adjust the focus by releasing tension on the suspensory fibers, or zonules, of the lens. Opacification of the lens causes a cataract. Uveitis is an inflammatory condition of these structures (iris, choroid, and ciliary body).
The innermost eye layer is the retina, which is a membrane consisting of highly specialized nerve tissue continuous with the optic nerve. Light stimulates retinal photoreceptors to produce neural signals that the optic nerve carries to the brain. There are no capillaries in the retina; the choroid layer provides oxygen and nourishment to the retina. Retinal detachment from the choroid layer compromises the retinal blood supply and is a major cause of vision loss. The retinal layer ends approximately 4 mm behind the iris. The area between the limbus of the cornea and the retina is called the pars plana . Because there is no retinal layer there, it is a safe entrance site for vitrectomy procedures.
The center of the eye is filled with vitreous gel. This thick fluid has attachments to blood vessels and the optic nerve. Traction of the vitreous on the retina is a cause of retinal detachment. Scarring, bleeding, or opacification of the vitreous is treated by vitrectomy.
The extraocular muscles move the globe within the orbit. They arise from a fibrous ring near the apex of the orbit and insert on the sclera. The six extraocular muscles lie within a cone behind the eye surrounding the optic nerve, ophthalmic artery and vein, and ciliary ganglion.
The eyelids have an outer layer of skin, a muscle layer, a tarsal plate of cartilage, and a layer of conjunctiva. The conjunctiva is a mucous membrane that lines the inner eyelids and covers the globe up to the corneal-scleral junction.
The lacrimal gland sits in the superior temporal orbit. It releases tears across the surface of the globe. Tears drain via the puncta near the medial canthus of the eyelids. Tears flow through the canaliculi to the lacrimal sac and duct, to drain into the nasopharynx.
The ophthalmic artery provides most of the blood supply to the orbital structures. It is a branch of the internal carotid artery, close to the circle of Willis. The superior and inferior ophthalmic veins drain directly into the cavernous sinus.
Cranial nerves (CN) innervate the ocular structures. The optic nerve (CN II) carries the neural signals from the retina. The oculomotor (CN III), trochlear (CN IV), and abducens (CN VI) control the extraocular muscles. Touch and pain sensations are carried via the trigeminal nerve (CN V). Sensation to the lower lid is via the maxillary nerve (CN V 2 ). Sensation to the upper lid is via the frontal branch of the ophthalmic nerve (CN V 1 ). The nasociliary branch of the ophthalmic nerve sends sensory fibers to the medial canthus, lacrimal sac, and ciliary ganglion.
The ciliary ganglion provides sensory innervation to the cornea, iris, and ciliary body. Parasympathetic fibers originate from the oculomotor nerve (CN III) and synapse in the ciliary ganglion before supplying the iris sphincter muscle. Sympathetic fibers originate from the carotid plexus and travel through the ciliary ganglion to innervate the dilator muscle of the iris. Local anesthetic blockade of the ciliary ganglion produces a fixed, mid-dilated pupil.
The facial nerve (CN VII) exits the base of the skull from the stylomastoid foramen. It supplies motor innervation to the orbicularis muscle via the zygomatic branch. Local anesthetic block of the facial nerve can prevent lid squeezing.
Oculocardiac Reflex
The oculocardiac reflex was first described by Aschner and Dagnini in 1908. It is also known as the trigeminovagal reflex . Traction on the extraocular muscles or pressure on the globe causes bradycardia, atrioventricular block, ventricular ectopy, or asystole. In particular, it is seen with traction on the medial rectus muscle, but it can occur with stimulation of any of the orbital contents, including the periosteum. The response fatigues with repeated stimulation.
The afferent of the reflex limb arises from the ophthalmic division of the trigeminal nerve and continues to the Gasserian ganglion and the sensory nucleus of the trigeminal nerve near the fourth ventricle. Here, the afferent limb synapses with the motor nucleus of the vagus nerve. The efferent impulses travel to the heart via the vagus nerve leading to decreases in both heart rate and contractility of the heart.
The reflex may be seen more often with procedures performed with topical anesthesia, and it occurs commonly in pediatric patients undergoing strabismus surgery. Retrobulbar block is not uniformly effective at preventing the reflex. Orbital injections can also trigger the response. The response is exacerbated by hypercapnia or hypoxemia. In the event of arrhythmia, the anesthesiologist should first ask the surgeon to stop manipulations. Any condition that may exaggerate the reflex such as hypoxia, hypercapnia, and an inadequate depth of anesthesia should be assessed and treated. If significant bradycardia persists or recurs, intravenous glycopyrrolate or atropine can be given. Rarely, epinepherine is given to treat severe bradycardia or asystole. Rarely, epinephrine is given to treat severe bradycardia or asystole.
Intraocular Pressure
Normal intraocular pressure (IOP) is 16 ± 5 mm Hg and a value in excess of 25 mm Hg is considered pathological. Normal IOP is necessary to maintain cornea curvature and a proper refracting index of the eye. The intraocular perfusion pressure, defined as the difference between the mean arterial pressure and the IOP, is part of the system regulating blood perfusion to the eye’s internal structures. High IOP impairs the blood supply, leading to a loss of optic nerve function.
The globe is a relatively noncompliant compartment. The volume of the internal structures is fixed except for aqueous fluid and choroidal blood volume. The quantity of these two factors regulates IOP. 80% to 90% of aqueous formation occurs through active secretion by the ciliary body mediated by Na-K ATPase and carbonic anhydrase enzymes. The remainder is from passive filtration and ultrafiltration across the ciliary epithelium. It then enters the angle of the anterior chamber to flow through the trabecular meshwork into the Schlemm canal and the episcleral veins. IOP is primarily regulated by the resistance at the trabecular meshwork.
Impairment of aqueous drainage at any point can elevate the IOP. Sclerosis of the trabecular meshwork is believed to cause the chronic pressure elevation in open-angle glaucoma. Closed-angle glaucoma occurs when there is an obstruction to aqueous drainage from closure of the anterior chamber angle. This happens from peripheral iris swelling or anterior displacement. Patients with a preexisting narrow angle may be predisposed to this condition. The acute increase in pressure causes severe pain and is an ophthalmologic emergency.
Anesthetic drugs and the conduct of anesthesia have important effects on IOP. All volatile anesthetic drugs cause a decrease in IOP. Nitrous oxide (N 2 O) has no effect on IOP. The commonly used intravenous induction anesthetics such as propofol, thiopental, and etomidate all reduce IOP, with propofol causing a moderate reduction in IOP even when used as low-dose intravenous sedation. Ketamine does not increase IOP (see the section Anesthesia for Pediatric Ophthalmology). The mechanism by which anesthetic drugs reduce IOP is not fully elucidated but involves depression of central nervous system ocular centers, resulting in relaxation of extraocular muscle tone. Short-acting opioids such as fentanyl, alfentanil, and remifentanil decrease IOP on induction of anesthesia. Midazolam has little effect on IOP, and sedation with midazolam has been used to facilitate the measurement of IOP in children. Nondepolarizing neuromuscular blocking agents (NMBAs) such as rocuronium, vecuronium, and atracurium have little effect on IOP. Succinylcholine increases IOP by about 8 to 10 mm Hg due to a variety of postulated mechanisms including reduced aqueous humor outflow, increased choroidal blood volume, and increased central venous pressure. IOP increases after reversal of nondepolarizing NMBA with neostigmine and atropine but remains unchanged with sugammadex.
The most significant rise in IOP during general anesthesia occurs at laryngoscopy and emergence; the increase in IOP is even greater with repeat laryngoscopy. Video-guided laryngoscopy causes a smaller increase in IOP than direct laryngoscopy. Insertion of a laryngeal mask airway results in little or no increase in IOP. Other anesthetic interventions that increase IOP include compression of the eyes by ventilation, hypoxia, hypercapnia, and hypertension. Coughing, straining, or vomiting can increase IOP 30 to 40 mm Hg. A poorly placed anesthesia mask can put enough pressure on the eye to reduce blood flow to zero. Ocular blocks initially increase IOP by 5 to 10 mm Hg, but this falls to below baseline values within 5 minutes. Peribulbar blocks cause the greatest increase in IOP, likely due to the greater volume of local anesthetic that is injected.
Physiological factors that increase IOP include being placed in supine, prone, or Trendelenburg positions. A normal blink increases the IOP by 10 mm Hg, while a forceful lid squeeze can increase IOP to more than 70 mm Hg.
Ophthalmic Drugs
Ophthalmic medications can be delivered by topical, ocular (intravitreal, subconjunctival, retrobulbar, and intracameral), and systemic methods. Topical eye drops may be absorbed systemically; significant routes of systemic absorption occur at conjunctival capillaries and nasal mucosa. Less significant absorption can occur through the lacrimal drainage system, pharynx, gastrointestinal tract, skin at the cheek and eyelids, and at the aqueous humor and inner ocular tissues. When systemic therapy is required, the blood ocular barrier may inhibit passage of lipophilic drugs; however, this barrier may be impaired by ocular inflammation, intraocular surgery and trauma, or ocular diseases. The population at risk of systemic side effects of eye drops are children and the elderly because of anatomical differences, risk of toxicity, the presence of concomitant diseases, and polypharmacy. Most drugs have negligible excretion into breastmilk, with the exception of Timolol eye drops, which can potentially cause adverse effects in the nursing infant.
The total dose of a drug delivered by topical eye drop can be significant. Consider that one drop (typically 50 μL) of 10% phenylephrine contains 5 mg of drug, which is appreciably more than the intravenous dose of phenylephrine (0.05-0.1 mg). Adverse reactions such as hypertension, arrhythmias, and adverse cardiac events have been reported.
Systemically administered drugs such as acetazolamide and mannitol can produce side effects such as fluid or electrolyte disturbance that can affect anesthetic management of these patients. Table 69.1 lists the systemic effects of ophthalmic medications.
Drug | Mechanism | Use | Side Effects |
---|---|---|---|
Acetylcholine | Cholinergic agonist | Meiosis | Bradycardia, bronchospasm, hypotension |
Acetazolamide (PO, IV, IM) | Carbonic anhydrase inhibitor | Decrease IOP, Glaucoma | Confusion, drowsiness, hypokalemia, hyponatremia, metabolic acidosis, abnormal hepatic function tests, polyuria, renal failure |
Anti-VEGF, e.g., ranibizumab, aflibercept | Inhibits vascular endothelial growth factor | Inhibits neovascularization | Conjunctival hemorrhage, eye pain, endophthalmitis, uveitis, stroke (in high-risk patients) |
Atropine | Anticholinergic | Mydriasis | Dry mouth, dry skin, fever, agitation (central anticholinergic syndrome) |
Cyclopentolate | Anticholinergic | Mydriasis, cycloplegia | Central anticholinergic syndrome (see atropine) |
Echothiopate | Irreversible cholinesterase inhibitor | Glaucoma | Total body inhibition of plasma cholinesterase Prolongs effects of succinylcholine |
Epinephrine | α-, β agonist | Mydriasis, decrease IOP | Hypertension, tachycardia, ventricular arrhythmias |
Mannitol | Osmotic diuretic | Decrease IOP | Initial increase in circulating blood volume; congestive heart failure in patients with poor ventricular function |
Phenylephrine | α Adrenergic agonist | Mydriasis, vasoconstriction | Hypertension |
Pilocarpine | Cholinergic | Constrict pupil | Bradycardia, bronchospasm |
Scopolamine | Anticholinergic | Mydriasis, cycloplegia | Central anticholinergic syndrome (see atropine) |
Tamsulosin | α 1 antagonist | Benign prostatic hyperplasia | Floppy iris syndrome |
Timolol | β 1 and β 2 antagonist | Glaucoma | Bradycardia, bronchospasm, exacerbate congestive heart failure |
Ophthalmic Procedures
- 1.
CATARACT EXTRACTION
Cataracts are opacities of the crystalline lens of the eye. Cataract extraction is performed under topical or regional block. General anesthesia is rarely used.
- a.
Phacoemulsification is the technique of choice for cataract surgery and refers to the use of ultrasonic vibration of fragments of the lens with simultaneous irrigation and aspiration. This technique allows for very small incisions. Femtosecond laser is a relatively new technique where laser energy is used for corneal incision, capsulotomy, and fragmenting the lens. Supplemental oxygen is contraindicated during its use because of fire risk.
- b.
Extracapsular cataract extraction refers to the removal of the lens, while leaving the posterior lens capsule and zonules intact to allow implantation of intraocular lens.
- c.
Intracapsular cataract extraction is the total removal of the opaque lens with the lens capsule, and it is rarely performed due to large incision and a relatively high rate of complications.
- a.
- 2
GLAUCOMA PROCEDURES
- d.
Trabeculectomy is a glaucoma surgery that creates a transscleral fistula to allow aqueous humor to drain into the subconjunctival space. Mitomycin C or 5-flurouracil (5-FU) is usually used to prevent scarring of the flap.
- e.
Baerveldt and Ahmed devices are drainage implantable devices that shunt aqueous humor under the conjunctiva.
- f.
Minimally invasive glaucoma surgery (MIGS) includes procedures such as CyPass, iStents, and trabectome.
- d.
- 3.
CORNEAL PROCEDURES
- a.
Penetrating keratoplasty is a full thickness corneal transplant.
- b.
Lamellar keratoplasty—In Descemet stripping endothelial keratoplasty (DSEK) and Descemet membrane endothelial keratoplasty (DMEK) procedures, only the diseased endothelium is removed and replaced with a corneal graft.
- c.
Pterygium excision—A pterygium is an abnormal growth of fleshy tissue on the conjunctiva. Excision is performed when it impinges on the cornea, affecting vision, or for cosmesis.
- a.
- 4.
VITREORETINAL SURGERY
- a.
Vitrectomy is the surgical removal of the vitreous humor and its replacement with a physiologic solution. A posterior vitrectomy is indicated for the removal of foreign bodies such as “dropped lens” through the torn posterior capsule during cataract surgery, to repair retinal detachments, to remove membranes and media opacities, and to alleviate vitreous traction on the retina.
- b.
Scleral buckle is a procedure used for the treatment of retinal detachment. A silicone band is placed around the globe within the extraocular muscles under the conjunctiva to push the sclera toward the detached retina. It can be used as the sole treatment or in combination with vitrectomy to treat retinal detachment.
- c.
Radioactive plaque implantation is done for the treatment of choroidal melanoma. It is frequently combined with prophylactic vitrectomy with silicone oil injection to prevent retinal detachment from radiation.
- a.
- 5.
OCULOPLASTIC SURGERY
- a.
Eyelid procedures. Eyelid procedures include the correction of ectropion (eyelid turning outward), entropion (eyelid turning inward), ptosis (drooping of the upper eyelid), and blepharoplasty (to remove redundant tissue of the eyelid).
- b.
Dacryocystorhinostomy refers to the surgical reopening of the obstructed channel between the lacrimal sac and the nasal cavity caused by a congenital defect or chronic infection.
- c.
Orbital surgery includes repair of blowout fractures, drainage of an orbital abscess, decompression for exophthalmos caused by hyperthyroidism, or tumor excision in the orbit or optic nerve.
- d.
Evisceration, enucleation, and exenteration. Evisceration refers to the removal of the contents of the eye. Enucleation refers to removal of the eye itself including the globe, but leaving orbital contents such as bone, extraocular muscles, and fat in place. Exenteration refers to the removal of the entire contents of the orbit including the lacrimal gland, optic nerve, and orbital bones.
- e.
Tarsorrhaphy is the partial or total suturing together of the eyelids.
- a.
Preoperative Evaluation
Exclusion criteria for ambulatory ophthalmic surgery under general anesthesia include noncompensated congenital cardiac and pulmonary disease, and some syndromes with multiple system involvement in pediatric patients. Severe cardiomyopathy, pulmonary hypertension, home-oxygen dependence, and super-morbid obesity (body mass index >50) are exclusion criteria in adult patients. Known difficult airway in either group may preclude outpatient surgery.
Laboratory Studies
Previous investigations have shown that preoperative laboratory tests are typically normal before low-risk ambulatory surgery, and the ASA recommends that routine preoperative laboratory testing before cataract surgery is not necessary in ASA physical status classification I and II patients. Routine preoperative testing has not been shown to decrease cancellation rate and adverse events, or to improve outcomes. While this is not extensively studied in other types of ophthalmic surgical procedures, a study examining preoperative medical testing for patients undergoing vitreoretinal surgery showed that preoperative testing did not influence rates of postoperative systemic complications. Any laboratory testing, including an electrocardiography, should be based on the patients’ history and physical findings rather than the indiscriminate ordering of a battery of tests.
Cardiovascular Evaluation
The American Heart Association and American College of Cardiology have published guidelines for perioperative cardiovascular evaluation for noncardiac surgery. Ophthalmic procedures, such as cataract extraction, are specifically identified as low-risk procedures. For these procedures, evaluation is focused on patients with major clinical predictors of risk.
Anticoagulation
Many elderly patients undergoing eye surgery are on antiplatelet or warfarin therapy. Perioperative management of anticoagulants involves weighing the risks of thrombosis versus hemorrhagic complications. In a study of more than 19,000 cataract procedures, the incidence of hemorrhagic and thrombotic complications was low. The risk of hemorrhagic complications depends on the degree of anticoagulation and the hemorrhagic potential of the surgical procedure. Serious hemorrhagic complications are most probable in orbital and oculoplastic surgery; of intermediate probability in vitreoretinal, glaucoma, and corneal transplant surgery; and least likely in cataract surgery.
It is safe to proceed with cataract surgery without stopping antiplatelet or warfarin therapy provided that the international normalized ratio level is within the therapeutic range. Ocular blocks are rarely associated with severe hemorrhagic complications. For intermediate risk surgery such as oculoplastic and glaucoma surgery, warfarin and antiplatelet drugs may increase the risk of intraoperative or postoperative bleeding. MIGS are associated with a 100% rate of hyphema, and the effects of anticoagulation therapy in patients undergoing these procedures is unclear.
There is limited data on the risk of hemorrhagic complications during eye surgery in patients who are taking novel oral anticoagulants (NOACs). A recent systematic review and meta-analysis found that NOACs reduced the risk of intraocular bleeding by onefifth compared with warfarin. However, another study comparing NOACs with antithrombotic drugs found no difference in the risk of substantial intraocular bleed, but the number of adverse events was scarce. In the authors’ institution, the ophthalmologist requests patients to stop NOAC therapy 2 days before intermediate-risk surgery.
If an interruption of anticoagulation treatment is needed, an individualized approach is recommended to minimize the risk of perioperative bleeding. Bridging therapy should be considered in patients with a high risk for thromboembolic events.
Orbital Blocks
Intraocular surgery can be performed under local, regional, or general anesthesia. Regional anesthesia is standard for most ophthalmic procedures such as cataract, glaucoma, cornea, and vitreoretinal surgeries. Regional anesthesia provides akinesia (immobility) and anesthesia of the eye. The requirement for akinesia will vary by type of surgery and surgeon preference. Facial nerve block may be required to obtain akinesia of the orbicularis oculi muscle of the lid, as its motor innervation is located outside the cone.
Regional anesthesia techniques are generally reliable, safe, and provide good postoperative analgesia. Compared with general anesthesia, regional anesthesia is less likely to be associated with PONV. Patients are often able to bypass Phase 1 recovery with a quicker discharge home. Patients often view eye blocks with anxiety and apprehension, and may have fears of being aware and awake during their procedure. Some patients may not be able to cooperate, to lay flat, or lie still during surgery, and therefore may not be able to tolerate surgery under regional anesthesia alone.
Retrobulbar Block
Retrobulbar block has long been considered the gold standard of regional techniques for ophthalmic surgery until the 1990s with the introduction of peribulbar block and sub-Tenon block. Retrobulbar block is achieved by injecting local anesthetic inside the muscular cone ( Fig. 69.2 ).

With the globe in primary gaze, a 3-cm, 23- to 27-gauge needle with its bevel opening faced toward the globe is placed at the junction of the inferior and lateral walls of the orbit just above the inferior orbital rim. The needle is advanced parallel to the orbit floor (with a 10-degree elevation from the transverse plane) for approximately 15 mm until it is past the equator of the eye. The needle is turned medially and slight upward to aim toward an imaginary point behind the globe on the axis formed by the pupil and the macula where 2 to 5 mL of local anesthetic solution is injected. The needle tip approaches but does not pass the midsagittal plane of the globe. Some intorsion on downgaze is expected because the superior oblique muscle is outside the muscle cone and may not be blocked. Many modifications to the classical retrobulbar anesthetic injection have been described to minimize complications of the block. Traditionally, blunt-tipped needles were advocated because they were thought to protect against ocular trauma and because they allowed more accurate definition of tissue planes. However, studies have shown that blunt needles are as likely as sharp needles to cause globe penetration and optic nerve injury. Fine cutting needles produce minimal tissue distortion with little pain. Compared with peribulbar block, retrobulbar block has a quicker onset and is associated with less chemosis.
Retrobulbar hemorrhage complicates about 1% of retrobulbar injections. Venous hemorrhage spreads slowly and does not usually result in long-term visual problems. Arterial hemorrhage can produce rapid orbital swelling, marked proptosis with immobility of the globe, inability to close the lids, and massive blood staining of the lids and conjunctiva. Compressive hematoma can threaten retinal perfusion leading to severe visual loss. Ophthalmoscopic examination should be performed to evaluate for ischemic damage to the optic nerve or retina. IOP should be measured and a lateral canthotomy may need to be performed to decompress the orbit.
Intravascular injection can occur despite a negative aspiration test. The total dose of local anesthetic used is small, and systemic effects are unlikely, even if the total dose is given intravenously. Accidental intraarterial injection can cause central nervous system excitation and seizures. This occurs because of retrograde passage of local anesthetic solution from the ophthalmic artery to the internal carotid artery and delivery to the thalamus and other midbrain structures. Injection of local anesthetic or retrograde tracking of local anesthetic along the optic nerve sheath into the subdural or subarachnoid space causes partial or total progressive brainstem anesthesia. Wide ranging symptoms occur, from aphasia, confusion, and dysphagia to apnea, cardiac arrest, loss of consciousness, and seizures. Management is supportive and should result in total recovery within several hours.
Optic nerve damage, or globe perforation with retinal detachment and vitreous hemorrhage, are devastating complications of retrobulbar block. Risk factors include physician inexperience and a highly myopic eye (axial length longer than 25 mm). This complication is associated with a poor prognosis, particularly in cases of delayed diagnosis.
Peribulbar Block
Peribulbar block was first reported in 1986. The needle is introduced outside the cone, thus preventing retrobulbar hemorrhage and reducing the risk of optic nerve injury (see Fig. 69.2 ). Many modifications of this block exist; the classic technique involves two injections—one inferiorly and temporally and the second superiorly and nasally. Peribulbar anesthesia may also be administered with one injection; a 3 cm, 23-gauge Atkinson needle is placed at the junction of the middle and lateral thirds of the lower lid just above the inferior orbital rim. The needle should be directed vertically backward, parallel to the floor of the orbit. The needle insertion depth should be less than 25 mm. If contact is made with bone, the needle should be redirected slightly upward then 5 to 10 mL of local anesthetic is injected. Studies support the use of peribulbar block in providing equivalent patient analgesia and operating conditions as retrobulbar block for a variety of ophthalmic procedures.
Sub-Tenon Block
To avoid the complications of sharp needles, a technique was developed using a blunt cannula under the fascia of Tenon. Various lengths of cannulas have been used. Using topical anesthesia with sedation, a speculum is placed to retract the lids. A 2- to 3-mm spot of cautery can be made 5 mm from the limbus in the inferonasal or inferolateral quadrant. A 2-mm incision is made in the conjunctiva with blunt dissection through the fascia. A blunt cannula is directed posteriorly, but not beyond the equator of the globe, with injection of 1 to 3 mL of local anesthetic. A small degree of conjunctival edema is often seen. Analgesia is usually excellent.
Facial Nerve Block
A facial nerve block is performed to prevent squeezing of the eyelids, which interferes with surgery and increases IOP. Akinesia of the orbicularis muscle, which is supplied by the facial nerve, can be achieved by blocking the nerve at its terminal branches (Van Lint block) or proximal trunk (O’Brien or Nadbath-Rehman block).
- 1.
Van Lint block: the needle is placed 1 cm lateral to the orbital rim, and 2 to 4 mL of anesthetic is injected along the superolateral and inferolateral orbital rims. The modified Van Lint block is a common variant achieved by inserting the needle 1 cm more lateral than the original insertion point to avoid lid edema. The disadvantages of this block include patient discomfort, proximity to the eye, and postoperative ecchymoses( Figure 69.3A ).
Fig. 69.3
Facial Nerve Blocks.
(A) Van Lint; (B) O’Brien; (C) Nadbath-Rehman.
Redrawn from Spaeth GL. Ophthalmic Surgery: Principles and Practice . Philadelphia: WB Saunders; 1982.
- 2.
O’Brien block: the mandibular condyle is palpated inferior to the posterior zygomatic process and anterior to the tragus of the ear as the patient opens and closes the jaw. The needle is inserted perpendicular to the skin approximately 1 cm to the periosteum. As the needle is withdrawn, 3 mL of anesthetic is injected ( Figure 69.3B ).
- 3.
Nadbath-Rehman block: a 12-mm, 25-gauge needle is inserted perpendicular to the skin between the mastoid process and the posterior border of the mandible. The needle is advanced its full length, and after careful aspiration, 3 mL of anesthetic is injected as the needle is withdrawn. This blocks the entire trunk of the facial nerve. The patient should be told to expect a lower facial droop for several hours postoperatively. The major disadvantage to this block is the proximity of the injection to important structures, such as the carotid artery and the glossopharyngeal nerve ( Figure 69.3C ).
Local Anesthetic Solutions for Regional Techniques
For local anesthesia, we use a 1:1 ratio of bupivacaine 0.75% and lidocaine 2% without epinephrine. Hyaluronidase is added to speed tissue penetration. Hyaluronidase can also be important in preventing anesthetic-related damage to the extraocular muscles. A human recombinant brand (Hylenex) is commercially available.
Monitored Anesthesia Care
Approximately 60% to 70% of ophthalmic procedures can be accomplished by a combination of orbital blocks and MAC. In the last 2 decades, the dramatic improvement in surgical techniques in ophthalmic surgery has had significant impact on anesthesia management rendering many ophthalmic procedures less invasive and shorter in duration.
MAC with regional anesthesia provides many advantages over general anesthesia in lowering postoperative morbidity, mortality, and pulmonary complications. There is better postoperative pain control, a lower incidence of intraoperative hypotension, faster postoperative recovery, less PONV, and less unplanned hospital admission.
Other than cataract surgery, most ophthalmic procedures require orbital blocks that are quick to perform but painful for the patient. Intravenous sedation and analgesia is provided to prevent pain and anxiety. Patient movement during orbital blocks, either from pain, anxiety, under sedation, or over sedation, has been linked to complications leading to blindness. Other unique intraoperative conditions make ophthalmic surgery challenging. Many elderly patients are anxious and cognitively impaired, making it difficult for them to lie flat and still during surgery. Covering the face with surgical drapes and rotation of the bed 90 or 180 degrees away from the anesthesia provider also increases difficulty by limiting access to the airway.
The technique for MAC is highly variable and depends on the training and experience of the anesthesia provider, the environment of practice, availability of drugs, and the surgeons’ and patients’ expectation for sedation. There is no universal regimen for MAC.
Intravenous sedation is a continuum ranging from mild and moderate sedation to deep sedation that overlaps with general anesthesia. The depth of sedation can quickly increase to a deeper level depending on age, weight, cardiac function, genetics, and general health. Some patients have a high tolerance to sedatives while others may be more sensitive. This variability presents a challenge for the fast-track practice of ophthalmic anesthesia. The newly published “Practice Guidelines for Moderate Procedural Sedation and Analgesia 2018” provides evidence-based recommendations for MAC that are also applicable in ophthalmic anesthesia.
The challenge of MAC in ophthalmic surgery is to quickly and safely sedate a patient adequately for an ocular block, and still maintain operating room efficiency. Ideally, the metrics of intravenous sedation in ophthalmic surgery should be quantitatively measurable, including the time required to reach the targeted level of sedation and analgesia, and the efficacy of pain control while avoiding respiratory depression or apnea. It is not necessary to use loss of consciousness as an endpoint of sedation. To do so can lead to apnea, hypoxia, involuntary movement in reaction to pain, and hemodynamic instability. During orbital blocks and surgery, patients should be sedated to a moderate level such as subscore 3 of the Observer’s Assessment of Alertness/Sedation Scale ( Table 69.2 ). The objective is to have a patient who is comfortable, free of pain and anxiety, yet able to follow commands in order to prevent movement that can lead to ocular trauma. Treating complications that occur during orbital blocks and being prepared to convert to general anesthesia in the event that MAC is inadequate, or the airway compromised are important responsibilities of the anesthesia provider.
Subscore | Responsiveness | Speech | Facial Expression | Eyes |
---|---|---|---|---|
5 | Responds to name spoken in normal tone | Normal | Normal | Clear |
4 | Lethargic response to name spoken in normal tone | Mild slowing or thickening | Mild relaxation | Glazed mild ptosis |
3 | Responds only after name spoken loudly or repeatedly | Slurring or slowing | Marked relaxation | Glazed marked ptosis |
2 | Responds after mild prodding or shaking | Few recognized words | ||
1 | Does not respond to mild prodding or shaking |
Monitored Anesthesia Care for Cataract Surgery Under Topical Anesthesia
Topical anesthesia has become popular due to its ease of administration and avoidance of complications of orbital blocks. Topical anesthesia requires patient cooperation because of the lack of ocular akinesia. Patients undergoing cataract surgery with topical anesthesia may experience some discomfort and require intravenous analgesic medication in small doses. Most patients undergoing cataract surgery want sedation, which can be given either intravenously or orally. Local anesthetic eye drops, such as 0.5% tetracaine, 0.75% bupivacaine, and 2% lidocaine, are very effective in producing analgesia although their effects can dissipate with constant irrigation.
With use of femtosecond laser, it may take up to 5 minutes to appropriately dock and position the patient, however it takes only 30 seconds for laser delivery. Discomfort during the procedure is managed with topical anesthesia. Ideally, sedation should be avoided to allow patient cooperation. If necessary, a small dose of a sedative or analgesic can be given.
Midazolam is the primary sedative used for anxiolysis during cataract surgery with topical anesthesia. Its side effects of over sedation, paradoxical reaction, postoperative neurocognitive disorders, and memory deficit are concerning in the elderly patient. Low-dose propofol, short-acting narcotics, or both are reasonable alternatives. Over sedation during cataract surgery should be avoided to prevent sudden awakening under surgery that may lead to undesired movement secondary to disorientation. Dexmedetomidine used as a sole medication is not appropriate for use in cataract surgery due to its slow onset, inadequate analgesic effect, and long elimination half-life. Ketamine alone is also not a good choice due to its dysphoric and hallucinogenic properties. Intraoperative handholding is helpful to provide reassurance and reduce anxiety.
Monitored Anesthesia Care for Ophthalmic Surgery with an Orbital Block
Orbital blocks, such as retrobulbar, peribulbar, and sub-Tenon blocks, are commonly performed nerve blocks for ophthalmic procedures. Orbital blocks are performed by either the ophthalmologist or the anesthesia provider. In an ASA Closed Claim Project analysis of complications associated with eye and peripheral nerve blocks, the risk of adverse events was increased when anesthesia providers performed both the orbital block and MAC.
Many sedatives and analgesics, either alone or in combination, are used during the performance of an orbital block. The use of sedatives alone such as midazolam, propofol, sodium thiopental, and dexmedetomidine can provide an adequate level of sedation, but their lack of analgesic effect may lead to involuntary head movement. The sole use of narcotics such as alfentanil, fentanyl, remifentanil, and sufentanil can provide profound analgesia, but inadequate sedation and increased nausea and vomiting.
Propofol is an excellent drug for providing titratable sedation. Its lack of analgesic effect makes it insufficient for orbital blocks as evidenced by a high rate of involuntary movement during injection. When ketamine is combined with propofol, up to onethird of patients who receive the combination will have undesired movement during the block.
The combination of a short-acting opioid such as alfentanil or fentanyl, and propofol, or a triple combination of propofol-midazolam-alfentanil produces a profound synergism of hypnosis and analgesia. When opioids are administered in combination with midazolam, propofol, sodium thiopental, ketamine, and dexmedetomidine, their sedative effects improved significantly.
The use of a mixture of propofol, alfentanil (500 μg/mL), and lidocaine 1% in a 6:2:2 ratio in volume is a novel approach in “balanced anesthesia” during MAC. It contains both a sedative and an analgesic in a single syringe that is easy to titrate based on the patient’s age and weight (or adjusted weight in the obese). The addition of lidocaine attenuates the pain from propofol. Within 30 to 90 seconds, most patients reach an OAA/S subscore 3 sedation-level and are ready for the ocular block. This mixture provides excellent analgesia and sedation, as well as hemodynamic stability, with minimal need for airway support. While infusing the mixture bolus, assessing the patient’s response to questions allows for detection of the target sedation level. Significant sedation may continue after the block for several minutes, however, conversing with and instructing the patient to breathe deeply will prevent hypoventilation and desaturation.
An orbital block provides analgesia for 2 to 3 hours, which will be sufficient for the duration of most ophthalmic procedures. For more complex procedures such as enucleation, dacryocystorhinostomy, orbital decompression, scleral buckle, and radioactive plaque implantation, the analgesic effect of a retrobulbar block may not be adequate to block all sensation. The addition of intravenous sedation with propofol and narcotics is sufficient for most patients. Low-dose ketamine and dexmedetomidine can be added to the sedation regimen in patients who continue to experience discomfort.
Intranasal dexmedetomidine is well absorbed without the profound bradycardia or hypotension associated with intravenous administration. When given as an adjunct agent, the dose should be reduced, 0.5 to 0.7 μg/kg, administered intranasally as a drop from a tuberculin (TB) syringe. The elimination half-life of dexmedetomidine is about 2 to 3 hours and even longer in elderly patients. Giving a single bolus toward the beginning of the procedure allows the drug to be eliminated during surgery and prevents adverse effects in the PACU. Intranasal dexmedetomidine can also be used in highly anxious patients who are undergoing complex procedures under MAC.
The following are key points of anesthetic management for ophthalmic procedures under MAC.
- 1.
Cataract surgery can be performed under topical anesthesia or an orbital block. Most patients require mild sedation (anxiolysis) for surgery performed under topical anesthesia. Moderate sedation is required for the performance of an orbital block, after which, mild sedation is adequate.
- 2.
Dacryocystorhinostomy: the success of the anesthetic management depends on an adequate initial dose of narcotics and propofol to prevent pain associated with the injection of local anesthetics near the tear duct and inside the nose. A continuous infusion of low-dose propofol and intermittent doses of narcotics, such as fentanyl, are usually necessary to provide adequate analgesia. The patient must be awake enough to protect their airway from aspiration of blood that enters the oropharynx from the nasolacrimal system. Adding intravenous low-dose ketamine or intranasal dexmedetomidine can provide additional analgesia if necessary. If the procedure is bilateral or a reoperation, general anesthesia is likely a better choice.
- 3.
Orbitotomy: the procedure can be done under MAC if performed primarily for debulking of soft tissue without bony involvement.
- 4.
Radioactive plaque implantation: the procedure requires incision of the scleral layer and suturing the plaque between the extraocular muscles. Significant manipulation during the procedure can cause discomfort. Even a well-executed retrobulbar block may not provide complete analgesia; a moderate to deep level of sedation is usually required. Many institutions perform this surgery under general anesthesia, but at the authors’ institution, MAC is routinely used. Continuous analgesia with narcotics, and a moderately dosed propofol infusion is highly effective. Low-dose ketamine or dexmedetomidine may be added for those patients who require additional analgesia.
- 5.
Cornea transplant: partial-thickness corneal transplant and penetrating keratoplasty can be carried out under retrobulbar block. Some surgeons also perform a facial nerve block to prevent eyelid squeezing. Intraoperatively, sedation with continuous infusion of propofol and titration of narcotics to achieve a moderate level of sedation is the goal. The patient must be awake enough that they will not move inadvertently especially during penetrating keratoplasty.
- 6.
Enucleation: this procedure can be performed with MAC in appropriate patients. In addition to a retrobulbar block, infiltration of the orbital area with bupivacaine at the end of the procedure can be helpful for postoperative analgesia. The anesthetic management is similar to that of radioactive plaque implantation discussed earlier.
- 7.
Ruptured globe: ruptured globe injury can be repaired under an orbital block and MAC depending on the degree of injury. It is crucial to minimize elevation of IOP during the block and surgery.
Special Considerations in Monitored Anesthesia Care
- 1.
Morbid obesity/obstructive sleep apnea (OSA). Morbid obesity has been identified as an independent predictor of sedation-related cardiopulmonary complications. In addition to obesity-associated comorbidities, such as OSA, restrictive lung disease, diabetes mellitus, and coronary artery disease, obese patients are at increased risk of airway closure and hypoxia during sedation. The pharmacokinetics of drugs are altered in the morbidly obese. Many drugs, including narcotics, should be dosed based on ideal body weight (IBW). When both propofol and alfentanil were given based on adjusted body weight, IBW + 30% of the difference between the patient’s total body weight—IBW, the sedation level, analgesic effect, and incidence of airway events were comparable to normal weight patients. Administration of 100% oxygen for several minutes, in addition to supplemental oxygen via nasal cannula before sedation, may alleviate hypoxemia during the orbital block.
- 2.
Elderly patients have increased sensitivity to all sedatives and narcotics, as well as increased risk of complications associated with MAC. In addition to a reduction in drug dose requirement, the onset of action will be slower compared with younger patients.

Full access? Get Clinical Tree
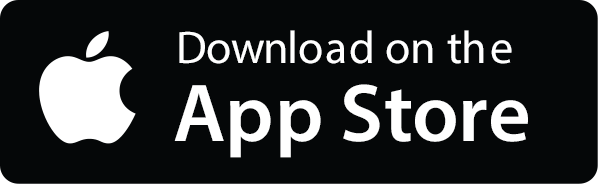
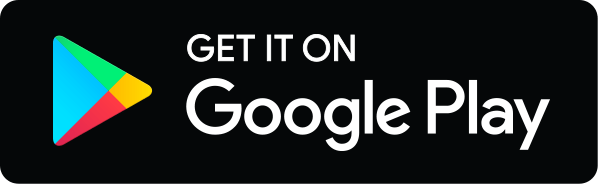