Antoun Koht
Neuroanesthesia is the practice of anesthesia related to the treatment of real or impending neurologic injury to the central nervous system (CNS) or peripheral nervous system (PNS). The CNS encompasses the brain and spinal cord, while the PNS includes all of the peripheral nerves of the body emanating from the spinal cord. As such, neuroanesthesia is the provision of anesthesia and analgesia for a multitude of procedures, including invasive, minimally invasive, and neurointerventional procedures, involving the brain, spinal cord, and peripheral nerves.
I. Neuroanatomy
The adult brain accounts for only 2% of total body weight but 20% of total body oxygen consumption. The different regions of the brain and spinal cord are responsible for distinct functions (Table 30-1). Blood flow to the brain is accomplished by two carotid arteries anteriorly (70%) and two vertebral arteries posteriorly (forming the basilar artery) (30%), which subsequently converge to form an anastomotic ring at the base of the skull known as the circle of Willis (Fig. 30-1). The spinal column is composed of 33 vertebrae (7 cervical, 12 thoracic, 5 lumbar, and 9 fused sacral and coccygeal), with nerve roots leaving the enclosed spinal cord and exiting through corresponding intervertebral foramina. The blood supply to the spinal cord entails one anterior spinal artery and two posterior spinal arteries. The anterior spinal artery originates from radicular arteries branching off the aorta, with the largest one being the artery of Adamkiewicz (usually at L1 or L2). The posterior spinal arteries originate from the posterior cerebral circulation (Fig. 30-2). The spinal cord itself terminates at L1 or L2 in adults, ending in structures known as the conus medullaris terminus and filum terminale.
Table 30-1 Functionality of Central Nervous System Structures
FIGURE 30-1 The circle of Willis, demonstrating the anterior and posterior blood supply to the brain.
FIGURE 30-2 The spinal blood supply. Note that the cervical spine is served by the posterior circulation emanating from the circle of Willis.
II. Neurophysiology
The cerebral metabolic rate of oxygen consumption (CMRO2) is normally 3 to 3.8 mL/100 g/min in adults. Normal cerebral blood flow (CBF) is 50 mL/100 g/min at rest, while glucose consumption is approximately 5 mg/100 g/min. The brain is dependent on a continuous supply of oxygen and glucose, with starvation and hypoxic damage resulting after roughly 5 minutes of global ischemia. The results of focal ischemia are less certain.
Cerebral perfusion pressure (CPP) is the difference between mean arterial pressure (MAP) and either intracranial pressure (ICP) or central venous pressure (CVP), depending on which is higher. Fortunately, even wide swings in MAP will yield a consistent CBF of 50 mL/100 g/min, thanks to autoregulation, which remains intact between a MAP of approximately 60 to 160 mm Hg (Fig. 30-3). The autoregulatory curve is shifted rightward in cases of chronic hypertension. Above and below these limits, CBF becomes pressure dependent as cerebral vessels are either maximally vasodilated (lower limit of autoregulation) or vasoconstricted (upper limit of autoregulation).
FIGURE 30-3 Autoregulation in the central nervous system. Cerebral blood flow remains constant between mean arterial pressures (denoted here as cerebral perfusion pressure) of 60 to 160 mm Hg.
Besides MAP, other physiologic parameters play an important role in controlling CBF. Arterial carbon dioxide tension (PaCO2) is the most important of these variables. CBF is linearly associated with PaCO2 between 20 and 80 mm Hg. Hence, hyper- and hypoventilation (both patient determined and iatrogenic) play critical roles in maintaining, decreasing (with hyperventilation), or increasing (with hypoventilation) CBF (Fig. 30-4). Oxygen tension in the arterial blood (PaO2) plays less of a role in controlling CBF unless marked hypoxemia (PaO2 <50 mm Hg) occurs, in which case CBF increases dramatically (Fig. 30-5). Temperature is also an important determinant of CBF, with a 6% to 7% decrease in CBF per 1°C drop in core temperature.
FIGURE 30-4 Autoregulation in the central nervous system. Cerebral blood flow varies linearly between arterial carbon dioxide partial pressures of 20 to 80 mm Hg.
FIGURE 30-5 Autoregulation in the central nervous system. Cerebral blood flow remains constant above an arterial oxygen partial pressure of 50 mm Hg.
Spinal cord physiology is very similar to brain physiology in that autoregulation is maintained and spinal cord perfusion pressure equals MAP minus ICP (pressure in the subarachnoid space).
III. Pathophysiology
Intracranial hypertension is any condition in which ICP is raised above 15 mm Hg. The cranium is a closed vault, composed of brain tissue, blood, and cerebrospinal fluid (CSF). When one of these components enlarges to occupy more space (e.g., brain tumor, bleeding), compensation occurs, usually by vasoconstriction and CSF drainage out of the cranium and into the spinal column. Intracranial elastance (as this is called), however, becomes very limited as ICP reaches a critical point, where sudden, even very small increases in volume can lead to dramatic increases in pressure within the cranium (Fig. 30-6). The results can be neurologically devastating, with herniation of the brain into the foramen magnum and subsequent irreversible damage or even death. Hence, meticulous care in those patients in whom elevated ICP is suspected (e.g., avoiding hypoventilation, emergent surgical decompression, or CSF diversion) is critical.
VIDEO 30-1
Intracranial Hypertension
FIGURE 30-6 The intracranial elastance curve is composed of three sections. (1) Intracranial pressure remains low and relatively constant at low volumes until the “elbow” of the curve is reached. (2) At this point, small changes in volume lead to moderate changes in pressure. (3) When a critical intracranial volume is reached, the pressure increases precipitously.
VIDEO 30-2
Intracranial Compliance Curve
As for the spinal cord, damage can be acute (leading to weakness, loss of sensation, or paralysis) or chronic (causing pain and deformity). Acute spinal cord compression, due to trauma or tumor, is usually a surgical emergency, as time to decompression is correlated with functional outcome. Patients may be flaccid initially and severely hypotensive, due to a relative sympathectomy. Resuscitation and hemodynamic support are mainstays of treatment at this time. The role of steroids in preventing secondary injury is much more controversial. Cervical injuries necessitate extremely careful management of the airway. These injuries are associated with more physiologic perturbations than lower injuries, including diaphragmatic paralysis, cardiac disturbances, and death.
IV. Monitoring
A. Central Nervous System Function
The most important monitor of CNS function is the awake and responsive patient who can be neurologically examined. Rarely, neurosurgery can be performed with an awake patient. Under general anesthesia, however, other modes of monitoring the CNS are necessary. Electrophysiologic (evoked potential) monitoring is commonly used in the operating room to assess the functional integrity of the CNS during surgeries that might put CNS structures at risk (1). The most commonly used modalities of evoked potential monitoring are somatosensory-evoked potentials (SSEPs), motor-evoked potentials (MEPs), and electromyography (EMG), with brainstem auditory-evoked potentials and visual-evoked potentials being less commonly used.
SSEPs are elicited from a peripheral nerve (e.g., median, ulnar, posterior tibial) and usually measured at the level of the subcortex and cortex. This modality is especially useful for monitoring the integrity of the dorsal columns of the spinal cord and the sensory cortex of the brain, where sensory fibers travel. MEPs are produced at the level of the cortex by direct or indirect stimulation and measured as compound muscle action potentials at the muscular level. MEPs are useful for assessing the motor cortex and the anterior spinal cord (corticospinal tracts) during surgeries that may put these structures at risk. EMG is a monitor that continuously assesses distinct nerve or nerve root integrity, either spontaneously or through elicited current, and is sensitive to mechanical and thermal damage to these structures.
Other monitoring modalities used to monitor the CNS (many referenced below) include transcranial Doppler ultrasonography, raw and processed electroencephalography (EEG), cerebral oximetry, and functional magnetic resonance imaging, among others.
B. Influence of Anesthetic Technique
Anesthetic drugs play a major role in determining how successfully these neuromonitoring modalities can be employed. Potent volatile anesthetics have the greatest inhibitory effect (decreased amplitude, increased latency) on obtaining robust evoked potential signals (SSEPs and MEPs). This is done in a dose-dependent fashion, especially as related to MEPs. Their effect on EMG is minimal. Nitrous oxide decreases signal amplitude with little effect on latency. Intravenous anesthetics have much less effect on SSEPs, MEPs, and EMG, but high doses of propofol can depress these signals. Etomidate and ketamine may increase the amplitude of SSEPs, whereas opioids generally have very little effect on evoked potentials. Neuromuscular blocking drugs inhibit MEPs and EMG by directly acting at the neuromuscular junction, but often will improve SSEPs by removing myogenic interference (2).
V. Cerebral Perfusion
A. Laser Doppler Flowmetry
Laser Doppler flowmetry (LDF) is a relatively new technique used in the study of hemodynamics to quantify blood flow in human tissue such as the brain. LDF employs a very small laser that can be implanted into the brain and measures the “scatter,” or Doppler shift, caused by passing red blood cells into microscopic vessels. Such measurements are useful in detecting the effects of various physiologic changes (e.g., anemia, hyperventilation) on CBF. Recent studies have shown LDF to yield measurements of CBF similar to xenon (133Xe), which is an isotope whose decay is measured in the brain as a marker of CBF. It is considered the gold standard of experimental CBF measurement (3).
B. Transcranial Doppler Ultrasonography
Transcranial Doppler ultrasonography (TCD) is a tool used in neurosurgery and neurocritical care by which an ultrasound probe is placed over a “window” (usually the temporal bone) to measure flow velocities of major cerebral vessels (usually the middle cerebral artery). Blood flow velocity is recorded by the ultrasound probe, which emits a high-pitched sound wave. That sound wave bounces off red blood cells and returns to the probe. The speed of the blood in relation to the probe causes a phase shift, with a higher or lower frequency directly correlated to a higher or lower velocity, respectively. Changes in these flow velocities (higher velocities) can indicate narrowing, emboli, or vasospasm of these vessels. Notably, TCD is unable to determine actual CBF; rather, it is primarily a technique for measuring relative changes in CBF over time (4).
Transcranial Doppler ultrasonography can noninvasively assess changes in cerebral blood flow.
C. Intracranial Pressure Monitoring
ICP monitoring is a useful tool for patients suffering from any cause of dangerously elevated ICP (e.g., brain trauma, bleeding, mass). Normal ICP is 5 to 15 mm Hg, and monitoring or treatment is generally initiated when ICP is >20 mm Hg. Waveforms of ICP can be transduced (A, B, and C waves) and may be useful diagnostically over time. ICP monitoring can be accomplished using a variety of devices, all of which currently are invasive. The most commonly used device is an external ventricular drain, which measures ICP by way of a transducer connected via tubing to the ventricle. It is also capable of removing CSF to relieve ICP. Other methods of monitoring ICP include the use of a subdural screw (usually performed urgently) placed through the skull and dura mater, an epidural sensor placed between the skull and the dura mater, or a tissue sensor placed directly in the brain parenchyma. These methods are incapable of diverting CSF.
D. Cerebral Oxygenation and Metabolism Monitors
Other devices used to monitor the homeostasis of the brain, including its oxygenation and metabolism, are available (often experimental) but may not be commonly used in the clinical setting. Jugular bulb venous oximetry is the most common of these techniques, which involves a fiberoptic catheter placed in a retrograde fashion into the jugular vein. This catheter is capable of measuring the mixed cerebral venous oxygen tension, which is indicative of the brain’s oxygen consumption or extraction. Other monitors used to measure cerebral metabolism include microdialysis catheters, which are multiparameter catheters that can detect focal brain tissue oxygen tension, glucose, pyruvate, lactate, glutamate, and glycerol levels by way of obtaining local perfusate from the brain. These catheters are becoming more popular in neurocritical care units but remain highly experimental (5). Lastly, cerebral oximetry has become more prevalent in the clinical setting recently, which involves a noninvasive measurement of regional cerebral blood oxygenation over the frontal cortices bilaterally. Oxygenation is given as a percentage, reflecting the contribution of both arterial (25%) and venous (75%) blood (6).
VI. Cerebral Protection
A. Ischemic and Reperfusion
Because of its high oxygen and glucose consumption, inability to store substrate, and inability to dispose of toxic metabolites, the brain is especially susceptible to rapid ischemic injury. With the accumulation of intracellular calcium under these ischemic conditions, neuronal damage quickly occurs and is compounded by the accumulation of lactic acid. Global ischemia, as is seen in conditions such as cardiac arrest, is responsive to interventions that restore total cerebral perfusion and oxygen-carrying capacity, such as cardiopulmonary resuscitation or red blood cell transfusion. Focal ischemia, on the other hand, is usually due to a regional insult, such as an embolus or intentional or unintentional arterial disruption. Treatment must be focused on restoring perfusion to the region in question. In cases of focal ischemia, a penumbra of salvageable tissue (watershed) usually surrounds the area that is damaged. Efforts must also be directed at “saving” this tissue, which is being supplied by some degree to collateral circulation. Much of the research being performed in cerebral protection today deals with this concept of “saving the penumbra.” Practical methods include augmentation of CPP and reducing brain edema in the acute setting (see below). Another area under heavy study is that of reperfusion and “reperfusion injury,” in which reperfusion of previously ischemic brain tissue can actually worsen neurologic outcomes largely due to the production of free radicals derived from oxygen and mediators of inflammation.
The brain is particularly susceptible to rapid ischemic injury because of its high oxygen and glucose consumption, inability to store substrate, and inability to dispose of toxic metabolites.
B. Hypothermia
Research into the cerebroprotective effects of hypothermia in humans has been largely disappointing, despite some encouraging animal studies. Theoretically, hypothermia should be extremely protective to the brain and spinal cord, as it lowers the CMRO2 for the CNS to a much greater extent than anesthetics would. Although anesthetics can cause an isoelectric EEG (electrical silence), reducing the brain’s metabolic activity by up to 60%, hypothermia can do far more by reducing even the brain’s homeostatic (e.g., mitochondrial) need for oxygen, which is required for basic neuronal survival. Despite this, mild to moderate reductions in core temperature in the face of cerebral ischemia have not yielded protective results in human studies and have been associated with worsened immunologic and clotting function (7).
C. Medical Therapy for Cerebral Protection
Similar to hypothermia, medical therapy for cerebral protection in its application to humans has been difficult to ascertain. Anesthetics, especially barbiturates, have been widely used in an attempt to lessen the burden of ischemia on neurons. Nearly all anesthetics (notable exceptions being ketamine and etomidate in low doses) can lower CMRO2 and theoretically protect the brain. But only barbiturates have been shown in humans to provide some protection from focal (not global) ischemia. No agents have been definitively shown to provide protection from global ischemia. Nimodipine, a calcium channel blocker, is frequently used in the setting of subarachnoid hemorrhage. It may have the benefit in neurologic protection during brain ischemia, although its protective mechanism remains elusive. Within 8 hours of acute spinal cord injury, methylprednisolone (a steroid) has been used to limit the degree of secondary injury due to edema, although controversy still surrounds this technique. Other more experimental agents, such as lidocaine, tirilazad (steroid), magnesium, dexmedetomidine (α2 agonist), and vitamin E (antioxidant) have been used in various ischemic settings, all with mixed results on neurologic protection and outcomes.
D. Glucose and Cerebral Ischemia
As mentioned previously, ischemia is rapidly detrimental to the nervous system not only because of oxygen starvation, but also because glucose is the only substrate that can be aerobically metabolized by the brain under normal conditions. Glucose is not stored in the nervous system, so when glucose is absent due to limited or absent cerebral circulation, adenosine triphosphate is no longer available to neurons and cellular injury quickly ensues. Cerebral glucose consumption (5 mg/100 g/min), on a time scale, mimics CMRO2, so hypoxemia and hypoglycemia are roughly equally detrimental to the brain. With cerebral ischemia and hypoglycemia, lactate is metabolized to some extent in the brain, but with much less efficacy than glucose. Hyperglycemia (serum blood glucose >180 mg/dL) in the setting of cerebral ischemia has also been shown to worsen neurologic outcomes, presumably by worsening cerebral acidosis in an anaerobic setting in which glucose is converted to lactic acid (8).
Glucose is the only substrate that can be aerobically metabolized by the brain under normal conditions.
E. A Practical Approach
“True” cerebral protection is hard to achieve or to prove, but practically speaking, certain techniques are commonly used for their possible benefit. Inhaled and intravenous anesthetics are generally “protective,” based on their known effect on CMRO2. For operations in which there is planned regional ischemia (e.g., temporary clipping of cerebral vessels during aneurysm surgery), propofol given in a large bolus (1 to 2 mg/kg) followed by a high-dose infusion (150 μg/kg/min) is often used and titrated to induce burst suppression on the EEG prior

Full access? Get Clinical Tree
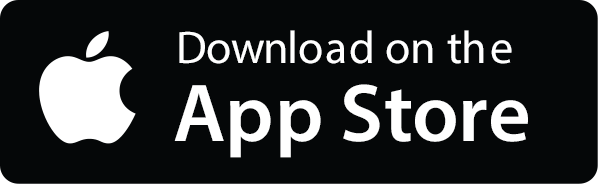
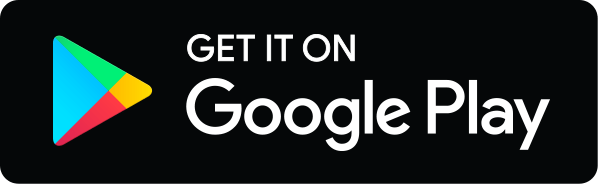