18 Anesthesia for Myocardial Revascularization
The role of the anesthesiologist in the perioperative care of patients presenting for myocardial revascularization continues to evolve. The anesthesiologist has to be well versed not only in a safe anesthesia technique, but in all areas of perioperative management in patients with coronary artery disease (CAD). This includes advances in pharmacologic risk reduction, new surgical techniques, and anesthetic management including monitoring techniques aiming to improve patient outcome. The overall number of patients presenting for coronary artery bypass graft (CABG) surgery has declined, mainly because of the growth of percutaneous coronary interventions (PCIs). Ischemic heart disease still accounts for approximately 1 of every 6 deaths in the United States. For 2010, it is estimated that 785,000 Americans will have a new heart attack, and every minute someone will die of it.1 Those patients who are not eligible for PCIs typically have an increased risk for perioperative morbidity and mortality and depend more than ever on optimal anesthetic management. This chapter provides a sequential approach to the major management issues faced by the practitioner who provides anesthesia for patients presenting for CABG surgery with cardiopulmonary bypass (CPB) or for off-pump coronary artery bypass (OPCAB) procedures.
Epidemiology
In 2010, it is estimated that 1 in 3 Americans in the United States have one or more types of cardiovascular disease, with CAD estimated to occur in 17,600,000 individuals in the United States. The total direct and indirect cost of all cardiovascular disease and stroke in the United States for 2010 is estimated to be $503.2 billion. Ischemic heart disease constituted 13.8% of the conditions of all Medicare beneficiaries in 2004; this number increased to 39.1% when only patients in the top 5% for all expenditures were considered.1 According to the same source, the number of cardiac catheterizations decreased slightly from 1996 to 2006 (Figure 18-1). There were 1,115,000 cardiac catheterizations performed in 2006, with 1,313,000 PCIs performed the same year.
Although precise data on the annual number of CABG and PCI procedures performed are not available, estimates based on sampling of large administrative and clinical databases, together with publications from discrete health care systems, have been used to derive strong estimates.2–4 The most widely cited data for nonfederal institutions in the United States are obtained from the U.S. Department of Health and Human Services National Hospital Discharge Survey.5 The latest data available estimated that, in 2006, 253,000 patients underwent a total of 448,000 CABG procedures.
Pathophysiology of coronary artery disease

Anatomy
The anesthesiologist should be familiar with coronary anatomy if only to interpret the significance of angiographic findings. An extensive review of cardiac anatomy can be found in reference cardiology or cardiac surgery texts.6,7 The following is an abbreviated description of the epicardial coronary anatomy. The coronary circulation and common sites for placement of distal anastomoses during CABG are shown in Figures 18-2 to 18-4.
The right coronary artery (RCA) arises from the right sinus of Valsalva and is best seen in the left anterior oblique view on coronary cineangiography (see Figure 18-2). It passes anteriorly for the first few millimeters, then follows the right atrioventricular groove, and curves posteriorly within the groove to reach the crux of the heart, the area where the interventricular septum (IVS) meets the atrioventricular groove. In 84% of cases, it terminates as the posterior descending artery (PDA), which is its most important branch, being the sole supply to the posterior-superior IVS. Other important branches are those to the sinus node in 60% of patients and the atrioventricular node in approximately 85% of patients. Anatomists consider the RCA to be dominant when it crosses the crux of the heart and continues in the atrioventricular groove regardless of the origin of the PDA. Angiographers, however, ascribe dominance to the artery, right coronary or left coronary (circumflex [Cx]), that creates the PDA.
The vertical and superior orientation of the RCA ostium allows easy passage of air bubbles during aortic cannulation, CPB, or open valve surgery. In sufficient concentration (e.g., coronary air embolus), myocardial ischemia involving the inferior LV wall segments and the right ventricle (RV) may occur (Figure 18-5). In contrast, the near-perpendicular orientation of the left main coronary ostium makes air embolization much less common.
The left coronary artery arises from the left sinus of Valsalva as the left main coronary artery. This is best seen in a shallow right anterior oblique projection (see Figure 18-3). The left main coronary artery courses anteriorly and to the left, where it divides in a space between the aorta and pulmonary artery. Its branches are the left anterior descending (LAD) and Cx arteries. The LAD passes along the anterior intraventricular groove. It may reach only two thirds of the distance to the apex or extend around the apex to the diaphragmatic portion of the left ventricle. Major branches of the LAD are the diagonal branches, which supply the free wall of the left ventricle, and septal branches, which course posteriorly to supply the major portion of the IVS. Although there may be many diagonal and septal branches, the first diagonal and first septal branches serve as important landmarks in the descriptions of lesions of the LAD (see Figure 18-4).
The Cx arises at a sharp angle from the left main coronary artery and courses toward the crux of the heart in the atrioventricular groove. When the Cx gives rise to the PDA, the circulation is left dominant, and the left coronary circulation supplies the entire IVS and the atrioventricular node. In approximately 40% of patients, the Cx supplies the branch to the SA node. Up to four obtuse marginal arteries arise from the Cx and supply the lateral wall of the left ventricle (see Figure 18-4). All of the previously described epicardial branches create small vessels that supply the outer third of the myocardium and penetrating vessels that anastomose with the subendocardial plexus. This capillary plexus is unique in that it functions as an end-arterial system. Each epicardial arteriole supplies a capillary plexus that forms an end loop rather than anastomosing with an adjacent capillary from another epicardial artery.8 Significant collateral circulation does not exist at the microcirculatory level. This capillary anatomy explains the distinct areas of myocardial ischemia or infarction that can be related to disease in a discrete epicardial artery (see Chapters 3 and 6).
CAD most commonly affects the epicardial muscular arteries with rare intramyocardial lesions (with the exception of the transplanted heart). However, severe disorders of the microcirculation and primary impairment of coronary vascular reserve in normal coronary arteries have been described, especially in diabetics, female patients, and those with variant angina.9–11 Atherosclerosis in all organs is most common at the outer edges of vessel bifurcations because in these regions blood flow is slower and changes direction during the cardiac cycle, resulting in less net shear stress (i.e., frictional force per unit area) than in other regions with more steady blood flow and higher shear stress.12 Low shear stress has been shown to stimulate an atherogenic phenotype in the endothelium. Epicardial lesions can be single but are more often multiple. A combined lesion of the RCA and both branches of the left coronary artery is referred to as triple-vessel disease. The left coronary artery supplies the thickest portions of the LV, at least the exterior two thirds of the IVS, and the greater part of the atria. Most bypass grafts are done on the left coronary system.
Venous drainage of the myocardium is primarily to the coronary sinus, which drains 96% of the LV free wall and septum, and the remainder of the venous return goes directly into the right atrium.7 A small fraction may enter other cardiac chambers directly through the anterior-sinusoidal, anterior-luminal, and thebesian veins.13

Myocardial Ischemia and Infarction
In patients with CAD, myocardial ischemia usually results from increases in myocardial oxygen demand that exceed the capacity of the stenosed coronary arteries to increase their oxygen supply (Figure 18-6). However, the determinants of myocardial oxygen balance are complex, and alterations may have several effects. For example, an increase in blood pressure (i.e., increased afterload) increases wall tension and oxygen demand while also increasing coronary blood flow (CBF). Myocardial ischemia may occur without changes in systemic hemodynamics and in awake patients may occur in the absence of chest pain (i.e., silent ischemia), particularly in patients with diabetes.
In atherosclerotic heart disease, the fundamental lesion is an intimal lipid plaque that causes chronic stenosis and episodic thrombosis, occurring most often in an epicardial coronary artery (Figure 18-7), thereby reducing myocardial blood supply. Characteristics of the vulnerable plaque include high lipid content, a thin fibrous cap, a reduced number of smooth muscle cells, and increased macrophage activity.14 The lipid core is the most thrombogenic component of the plaque.

Figure 18-7 Lipoid plaque lesion of a coronary artery.
(From Davies MJ: A macro and micro view of coronary vascular insult in ischemic heart disease. Circulation 82(Suppl II):II-38, 1990, by permission of American Heart Association.)
Fuster et al15 described five phases in the progression of CAD by plaque morphology. Phase 1 is a small plaque present in many people younger than 30 years and usually progresses very slowly, depending on the presence of risk factors associated with CAD (i.e., increased low-density lipoprotein cholesterol). Phase 2 is a plaque with a high lipid content that has the potential to rupture. If it ruptures, it will lead to thrombosis and increased stenosis (phase 5), possibly producing unstable angina or an acute coronary syndrome. The phase 2 plaque usually does not rupture; it instead progresses into phases 3 and 4, with enlargement and fibrous tissue organization, which ultimately may produce an occlusive plaque at phase 5.
Acute coronary syndrome is produced by a sudden decrease in CBF. In unstable angina, a relatively small fissure in a plaque may produce a temporary thrombotic occlusion of a vessel.16 Release of vasoactive substances from platelets and white blood cells, as well as dysfunction of the endothelium, may lead to vasoconstriction and reduction of CBF.17,18 Reduced vasoconstriction, spontaneous thrombolysis, or the opening of collateral channels may limit the duration of myocardial ischemia. A larger plaque disruption and prolonged thrombosis will produce a Q-wave infarction with transmural myocardial necrosis. The potential lesions seen with plaque fissure are shown in Figure 18-8. Several studies have found that the coronary artery responsible for an acute infarction is often only moderately obstructed.19,20 It is the extent of plaque rupture and thrombosis that determines the size and extent of an infarction, rather than the degree of stenosis. Patients with severely obstructed coronary arteries often have extensive collateral circulations that protect them from infarction. Similar findings have been reported in patients developing postoperative myocardial infarction after noncardiac surgery.21–23

Figure 18-8 Possible outcomes of intimal plaque rupture.
(From Davies MJ: A macro and micro view of coronary vascular insult in ischemic heart disease. Circulation 82(Suppl II):II-38, 1990, by permission of American Heart Association.)
The physiology of the coronary circulation is reviewed in Chapter 6. CBF in the normal individual is independent of perfusion pressure, but it is related to tissue oxygen demand.7 This phenomenon is referred to as autoregulation. Autoregulation affects all layers of the ventricular wall, maintaining essentially equal flows from the epicardium to the endocardium.24,25 The difference between autoregulated flow and maximal flow constitutes the coronary vascular reserve. As an obstruction of a coronary artery increases in size, dilation of the capillary bed occurs, with maintenance of adequate blood supply as the result. Coronary flow reserve, however, is diminished and eventually exhausted, and autoregulation begins to fail. Autoregulatory failure is loss of metabolic control of CBF and the sole dependence on pressure gradients to determine flow. Autoregulation fails first in the subendocardium, where blood flow fails to match demand, resulting in subendocardial ischemia and dysfunction.11,26
Autoregulation is pressure dependent, in that as perfusion pressure declines below a critical value, autoregulation begins to fail. In conscious animals, the pressure at which autoregulation begins to fail is very low (mean arterial pressure [MAP] of 38 to 40 mm Hg).27 Because CBF reserve is diminished in CAD, the pressure at which subendocardial autoregulation fails is increased. Heart rate (HR) also appears to have an effect on autoregulation. In an unanesthetized animal model, when the HR was doubled, the perfusion pressure that produced failure of subendocardial autoregulation was increased from a mean of 38 mm Hg at the normal HR to a mean of 61 mm Hg at the doubled rate.28 This pressure is not that much less than normal perfusion pressures. This effect of HR on subendocardial autoregulation is related to the increased myocardial oxygen consumption and flow caused by tachycardia and to the reduction in diastolic time and, therefore, perfusion. The net effect is a reduction in coronary vascular reserve and earlier failure of autoregulation, with resultant subendocardial ischemia. Tachycardia, because of its effects on demand and supply (reduction in diastolic perfusion time), is especially deleterious in the presence of CAD, in which perfusion pressures beyond an epicardial arterial obstruction are unknown.29 Avoidance of hypotension and tachycardia has been the basic tenet of anesthetic practice in patients with CAD for many years.
A coronary arterial stenosis may be rigid or, more commonly (70%), compliant in nature.30 When the pressure in the coronary circulation distal to a fixed stenosis is decreased, flow across the stenotic area decreases. Conversely, when the pressure distal to a compliant obstruction is increased, flow across the lesion is increased.31 The clinical implication is that decreased blood pressure to an area of myocardium supplied by a vessel with a variable stenosis will decrease blood supply to the myocardium by two mechanisms: loss of collateral flow and decreased flow across the compliant stenosis. Collateral vessels exist in normal hearts, but in the presence of CAD, they are increased in size and number.32 When a coronary vessel has a high-grade stenosis, the microvasculature distal to it may be maximally dilated even at rest and subject to ischemia. Collaterals may develop between this ischemic zone and an adjacent nonischemic area supplied by a different vessel. When vasodilation of the microcirculation is induced by exercise or drugs, perfusion of the ischemic bed by the collateral circulation may be decreased or cut off, particularly when the collateral vessels are poorly developed, because these present a greater degree of resistance than collaterals that are well developed. When vasodilation occurs in the nonischemic bed, pressure within it is decreased, and flow across collateral vessels with high resistance is reduced. An increase in myocardial blood flow in one region that reduces flow in another is referred to as a coronary steal.33 In experimental models, a partial lesion of the epicardial artery supplying the collateral vessel is required to produce a coronary steal.34 This anatomic configuration, called steal-prone anatomy, occurred in 23% of patients with symptomatic CAD in one large registry of coronary angiograms.35
The hallmark symptom of myocardial ischemia is pain. It has been found, however, that significant ischemia may occur without pain (“silent ischemia”).36,37 Early research using ambulatory electrocardiographic (ECG) monitoring reported that these silent episodes were frequent and occurred at lower HRs and activity levels when compared with exercise stress test data for the same individuals.38 Silent ischemic episodes are not considered to be an electrical anomaly but are due to myocardial perfusion impairment resulting in reduced regional function.39 A study of patients presenting for elective CABG confirmed these findings in the perioperative period: 42% of 50 patients studied had ischemic episodes before surgery, 87% of these episodes were clinically silent, and few were precipitated by adverse hemodynamics.40 In summary, there is no doubt that primary reductions in myocardial oxygen supply because of hypotension, anemia, or coronary vasoconstriction are important mechanisms in the development of ischemia, particularly in the perioperative period.
Risk assessment in patients scheduled for coronary artery bypass grafting surgery
Operative mortality (usually defined as death within 30 days of surgery), as expected, varies widely with patient risk, acuity, and previous cardiac surgery. On average, however, it appears that mortality has declined progressively despite increases in patient risk (Figure 18-9).41 The rapid growth of PCI has led to the perception of a shunting of healthier patients away from CABG, leaving a greater percentage of older and sicker patients not studied in the original CABG efficacy trials (e.g., the seminal coronary artery surgery study [CASS] trial excluded patients with ejection fractions [EFs] less than 35%). Publications since 1980 addressing whether patients presenting for CABG are sicker or at greater risk than they were previously have uniformly answered this affirmatively, noting older patients, worse ventricular function, and more emergent cases.41 Despite these findings, however, operative mortality continues to decline. Davierwala et al42 observed that LV dysfunction and reoperative status have decreased in significance as predictors of mortality in a large cohort of Canadian patients, whereas emergency surgery has increased in importance in predicting adverse outcome.
Preoperative risk assessment for patients undergoing CABG has evolved dramatically since 1990, driven by a variety of diverse factors. Institution of a federally mandated accounting of surgical outcomes for cardiac surgery in the Department of Veterans Affairs in the 1970s led to the establishment of what is considered the first large-scale, multicenter surgical outcomes database applying rigorous statistical methodology for comparing outcomes between centers.43,44 This group and others have pioneered methodology for adjusting for different severities of illness between patients (i.e., risk adjustment) using multiple preoperative and perioperative variables thought to be of intrinsic value (usually by expert consensus) that easily could be captured and have high consistency of definition. Entering these variables into logistic regression models of mortality (considering all of the patients operated on in the particular time frame in which the variables were collected) allows determination of an “expected” mortality based on coefficients of the regression equation most correlated with outcome. By simple comparison of the “observed” to “expected” (i.e., the value calculated by entering a unique patient’s coefficients into the general population mortality model) mortality ratio (O/E ratio), hospitals in a particular system can be ranked from best (low O/E ratio) to worst (high O/E ratio).45 However, as observed by a variety of experts, caution is advised in use of such adjectives given inherent assumptions and controversies in the statistical approaches for determining thresholds for “quality” and problems in “gaming” of the system by exploiting variables with imprecise definitions.46
Nonetheless, this methodology and its variants have been adopted widely by many organizations as a measure of quality of care.47 The Society of Thoracic Surgeons (STS) instituted a voluntary clinical database system with this approach in the early 1990s, which has continued to grow rapidly as cardiac surgical groups are increasingly interested in benchmarking their practices against others.48,49 Many states have established and maintain risk-adjusted mandatory reporting systems for hospital and individual surgeon performance.50 A natural offshoot of this approach has been the exploration of surgeon and hospital volumes as predictors of outcome, a topic that has generated substantial controversy in the literature given conflicting findings (i.e., low-volume centers in which “high-volume” surgeons operate appear to do as well as high-volume centers, and low-risk patients do better relative to high-risk patients at high-volume centers).51,52 This is a hotly contested topic given the high stakes involved in federal and private sector initiatives to regionalize cardiac surgery care into “centers of excellence.”53 The EuroSCORE scoring system is based on outcomes in 128 centers in 8 European countries and has received increasing attention. It appears to compare favorably with the STS model in North American patients.54,55 It is freely accessible by means of an interactive Web-based calculator (www.euroscore.org) and is decidedly simpler and faster to use than the STS’s scoring system, which is now also freely accessible to the public (http://www.sts.org/sections/stsnationaldatabase/riskcalculator/index.html).
Given the mass of literature on this topic and the large number of risk models available, some of which are easily calculated (e.g., a simple additive score), whereas others require manipulation of a logistic regression equation on an advanced calculator or microcomputer, considerable debate exists about how they are best applied. The American College of Cardiology/American Heart Association CABG guidelines group accords a Class IIa recommendation (level of evidence C) to their use for predicting hospital mortality.56 However, they caution that risk scores should be individually calibrated for regional mortality rates (a difficult task) and updated periodically to maintain accuracy (many have not been, with the exception of the Department of Veterans Affairs program and the STS).
Table 18-1 presents an evaluation of the importance of risk factors in several major risk models and large-scale outcome analyses grouped by the classification that Jones et al57–63 advocated.
The major database models only predict perioperative mortality (usually defined as death within 30 days of surgery, although some use any death directly related to a perioperative complication regardless of the time interval, a factor further complicating comparison of models). As such, these models are often of less practical importance to the cardiac anesthesiologist. Predicting which patients are most likely to be difficult to wean from CPB because of development of a low cardiac output syndrome (LCOS) in the operating room or early postoperative period, or who may acquire postoperative complications, is of greater interest to the anesthesiologist. Despite publication of several studies, difficulty in standardization of what constitutes difficult weaning or LCOS has limited generalization of findings. However, given that most CABG mortality is related to difficulty in revascularization and/or myocardial protection, the risk factors are quite similar to those reported for overall mortality.64,65 An analysis of 1009 patients undergoing CABG at Duke University, all of whom were monitored with transesophageal echocardiography (TEE), found six independent predictors of inotrope support, which was required in 39% of the cohort during weaning from CPB (Table 18-2 and Figure 18-1066; see Box 18-1).
BOX 18-1 Preoperative Evaluation and Management
The introduction and rapid growth of OPCAB have complicated matters because large-scale, well-calibrated outcome models are not yet available for this procedure. Initial publications have addressed this topic.67,68
Considerable data have been reported on the potential impact of various anesthetic techniques on outcomes, particularly effects on pre-CPB myocardial ischemia and postoperative myocardial infarction. However, much of these data suffer from inadequate statistical power, use of single centers, and difficulties in standardization of definitions or reporting of surrogate (otherwise called soft) outcomes. Earlier reports supported a lack of effect of the anesthetic technique, suggesting that hemodynamic control was more important (e.g., “It’s not what you use, but how you use it.”), particularly for prevention of ischemia.69,70 However, newer data support various degrees of efficacy with different approaches for preserving ventricular function with volatile anesthetics on weaning from CPB,71,72 or high sympathetic blockade relative to intravenous techniques.73 Some of these have been linked to improved recovery or shorter lengths of stay. However, the advantages appear to be modest, and demonstration of overt reductions in serious morbidity or mortality in large-scale, multicenter cohorts has not yet been reported. Similar data suggest that although use of a specific anesthetic agent (e.g., opioid or neuromuscular agent) may facilitate earlier extubation, the small magnitude of effect in most instances does not appear to reduce overall length of hospital stay, have significant effects on recovery, or substantially reduce cost.74,75 Nonetheless, nearly all anesthesiologists continue to strive for the “perfect technique.”
Several publications have attempted to more precisely delineate the association of perioperative hemodynamics with outcomes in larger cohorts of patients than have been previously reported in the older randomized trials of anesthetic techniques. Reich et al76 merged computerized anesthesia record data from 2149 CABG patients (with CPB) at two New York hospitals from 1993 to 1995 with outcome data from the state’s mandatory reporting database. Four independent predictors of mortality were identified: high mean pulmonary arterial pressure (PAP) before CPB (>30 mm Hg; odds ratio [OR] = 2.1), low MAP during CPB (40 to 49 mm Hg; OR =1.3), tachycardia (HR > 120 beats/min; OR = 3.1), and high diastolic PAP (>20 mmHg; OR =1.2) after CPB. The investigators observed that three of the four are markers of severe LV dysfunction.
Morbidity and mortality also are influenced by events in the operating room, including time on CPB and aortic cross-clamping, adequacy of revascularization, and complications such as cardiovascular decompensation or bleeding. Reevaluation of the patient’s risk can be performed on arrival in the intensive care unit (ICU) at the start of the postoperative period. The APACHE (Acute Physiology and Chronic Health Evaluation) III system has been modified for CABG patients, and Becker et al77 found that predictors of outcome included the risk score, age, reoperative status, and number of grafts. Higgins et al78 devised the Cleveland Clinic ICU admission score, which includes preoperative and physiologic factors present on admission to the ICU. Morbidity rate was 3% with scores of 5 or less, and the rate increased to 83% with scores greater than 20.
The SYNTAX (Synergy between PCI with TAXUS drug-eluting stent and cardiac surgery) trial, a prospective, randomized, multicenter trial, originally was designed to evaluate current practice patterns and to evaluate optimal revascularization strategies in patients with three-vessel and left main CAD in Europe and the United States.79 For the purpose of risk stratification in this trial, the SYNTAX score was developed, characterizing the complexity of coronary pathology. The SYNTAX score is based on already existing classifications and takes number, location, complexity, and functional impact of the coronary lesions into consideration.80 Patients with more complex disease and potentially worse prognosis have greater SYNTAX scores. The initial analysis showed that there was no difference in outcome (major adverse cardiovascular and cerebrovascular events) among patients randomized to surgery between those who had low, intermediate, and high scores (major adverse, cardiovascular, and cerebrovascular events 14.4%, 11.7%, 10.7%, respectively).81 In the patients randomized to PCI, however, the SYNTAX score was able to predict adverse outcome between the earlier mentioned risk groups at 12 months (13.5%, 16.6%, 23.3%, respectively). Based on these findings, it was concluded that patients with a low-risk SYNTAX score constellation of three-vessel and/or left main disease can be treated with surgery, PCI, or both, whereas intermediate-and high-risk groups should be referred for surgery. Further analysis of the SYNTAX trial and associated risk score will be performed at medium and long-term time points.
Anesthesia for coronary artery bypass grafting

Role of Central Neuraxial Blockade
A wide variety of techniques have been used for anesthetic induction and maintenance for CABG. A balanced general anesthetic, however, is still the most commonly used anesthetic technique in patients undergoing CABG surgery. Nevertheless, the interest in the use of thoracic epidural anesthesia (TEA) for cardiac surgery has increased steadily since 1990. It has been long appreciated that thoracic sympathectomy has favorable effects on the heart and coronary circulation.82 Its coronary vasodilating effects have been well documented, and it has been used to treat unstable angina for many years, albeit infrequently because of logistics and the contemporary standard of use of potent antiplatelet agents. There has been increased interest in TEA including reports of using chronically implanted, patient-controlled catheters83–87 and its use as a supplement to general anesthesia for cardiac surgery, particularly in Europe and Asia (Figure 18-11).88–90
TEA in conscious patients appears to be increasingly used for OPCAB or minimally invasive direct coronary artery bypass approaches with recent reports from diverse settings (e.g., Canada, Germany, Turkey, India), and has been designated as conscious OPCAB.91–96 Although most reports were of relatively small patient cohorts (15 to 30), Karagoz et al97 described 137 patients, of whom 97% were successfully managed. In the reported series, most grafts were single-vessel left internal mammary artery (LIMA)-to-LAD grafts, although two-vessel and even a small group of three-vessel procedures were performed successfully. In most series, 2% to 3% of catheters were unable to be placed in potential candidates, and 2% to 3% of patients were converted to general anesthesia because of a large pneumothorax or incomplete analgesia. Patients were fast-tracked, an ICU stay was not used, and some were discharged from the hospital the day of surgery. Patient acceptance appeared to be quite high. No complications related to TEA were observed. This is clearly an area of growing interest and one that has potential advantages, particularly for countries with different health care systems, resource constraints, and sociocultural differences.
Given the fact that most conscious OPCABs are performed mostly for single-vessel LAD lesions, the sophistication and aggressiveness of the interventional cardiologists at a particular institution are major variables. Noiseux et al98 recently published a series of 15 patients undergoing conscious OPCAB surgery combining a high TEA with a femoral nerve block for venous graft harvesting. Three patients needed conversion to general anesthesia. The authors concluded that even though this technique is feasible, technical limitations still exist that need to be overcome.
When neuraxial techniques are compared with general anesthesia alone, or as a combined technique, no significant difference in measured major outcome parameters such as perioperative mortality and major morbidity were found in most studies.99–101 Differences in minor outcome findings such as quality of analgesia and time to extubation were reported in others. In a prospective study, Scott et al102 randomized 420 patients undergoing CABG surgery under general anesthesia to either TEA or intravenous narcotic analgesia. No neurologic complications were associated with TEA. Many of the examined outcome parameters such as time to extubation, pulmonary function, atrial fibrillation, and renal function were significantly better in the TEA group. However, this study was widely criticized for design flaws that may have impacted the reported findings.
Priestley et al103 consequently conducted another prospective, randomized study and did not find improved pulmonary or cardiac function with TEA or decreased length of hospital stay, despite improved analgesia and earlier extubation. In 2006, Hansdottir et al104 randomized patients who all had a general anesthesia for cardiac surgery to either TEA (inserted before surgery) or intravenous morphine for postoperative analgesia. Again, even though time to extubation was shorter in the TEA group, none of the other examined outcome parameters was significantly different between the two groups. The authors concluded that TEA combined with general anesthesia offers no major advantage when compared with general anesthesia alone. Bracco et al105 found fewer postoperative complications such as delirium, pneumonia, acute renal failure, and myocardial function in patients undergoing cardiac surgery who had TEA in addition to general anesthesia, compared with general anesthesia alone. Based on shorter ICU and mechanical ventilator times in patients with TEA, they calculated $8800 cost savings per person if TEA was used. A recently published randomized trial in obese patients (body mass index > 30 kg/m2) undergoing OPCAB surgery showed better analgesia, improved lung function tests, and shorter time to extubation and length of ICU stay in patients who had TEA in addition to general anesthesia.106 Liu et al107 reported a meta-analysis of 15 randomized trials of TEA in 1178 patients. In contrast with an earlier mixed meta-analysis (i.e., cardiac and noncardiac surgery, observational and randomized),108 there were no effects on postoperative myocardial infarction or mortality. However, significant favorable effects were observed for arrhythmias, pulmonary complications, time to extubation, and reduction in visual analog pain scales.
In a propensity-matched, retrospective study, Salvi et al109 compared high TEA combined with general anesthesia with a total intravenous anesthesia technique (without TEA) in 1473 patients undergoing CABG surgery. There were no major differences in measured early outcome parameters (postoperative mortality, myocardial infarction, stroke, acute renal failure, ICU stay) between the two techniques. Patients with high TEA had shorter time to extubation.
The potential advantages of TEA on cardiac function were investigated specifically in the following studies. Berendes et al110 reported improved regional LV function (by wall motion score index) with lower troponin I and atrial and brain natriuretic peptide levels. However, the control for this study was a total intravenous anesthetic technique, and it is unclear whether a volatile anesthetic would have similar effects, as demonstrated by several investigators. In a prospective, controlled study, Barrington et al111 randomized 120 patients to general anesthesia with or without high TEA. Even though postoperative analgesia was improved in the TEA group and led to earlier extubation, there was no significant difference seen with troponin levels between the two groups. Crescenzi et al112 evaluated the effect of TEA on N-terminal-protein-B-natriuretic peptide levels in elderly patients undergoing CABG surgery. TEA, in addition to general anesthesia, significantly attenuated N-terminal-protein-B-natriuretic peptide release. Lee et al113 addressed a slightly different question, using total spinal sympathectomy (bupivacaine, 37.5 mg) before induction of general anesthesia. Patients randomized to bupivacaine had less β-receptor dysfunction in response to CPB of more than 1 hour, with lower catecholamine levels, a greater cardiac index, and a lower pulmonary vascular resistance index in the post-CPB period.
Safety concerns are a major consideration in use of neuraxial techniques in patients undergoing cardiac surgery given chronic use of antiplatelet agents, use of systemic anticoagulation and platelet inhibition for acute therapy of unstable angina, and high-dose systemic anticoagulation and potential coagulopathy induced by CPB. The true incidence of serious complications (particularly epidural hematoma) is unknown. The most recent and widely quoted estimation of the risk for epidural hematoma with TEA in patients undergoing cardiac surgery is 1 in 12,000, with 95% confidence intervals of 1:2,100 to 1:68,000, and 1 in 1000 with 99% confidence.114 Intrathecal risks from a different older source of risk assessment are quoted as 1 in 3610 and 1 in 2400, respectively.115 Even when under-reporting of such complications is assumed,116 there is increasing evidence that neuraxial anesthesia can be performed safely even in patients undergoing cardiac surgery with full heparinization.
Chakravarthy et al117 presented an audit of 2113 cardiac surgery TEA cases over a 13-year period with no permanent neurologic deficits, a 0.9% dural puncture rate, and 0.2% transient neurologic deficits. Jack et al published their experience of thoracic epidural catheter placement in 2837 patients undergoing cardiac surgery.118 No epidural hematoma was seen in this series. Similar results were reported by Royse et al,119 who reviewed 874 cardiac surgery cases involving epidural anesthesia over a 7-year period with no complications attributable to epidural catheter use. Pastor et al120 reported 714 uneventful cases over a 7-year period, emphasizing their use of safety guidelines in which antiplatelet drugs were discontinued 7 days before surgery, and routine coagulation tests and neurologic examinations were performed after surgery.
Careful attention to the most recent guidelines on neuraxial anesthesia in the setting of anticoagulant and antiplatelet agents is of paramount importance. The American Society of Regional Anesthesia and Pain Medicine published Consensus Statements on Neuraxial Anesthesia and Anticoagulation.121 These include recommendations for appropriate withdrawal of anticoagulant and antiplatelet therapy before neuraxial anesthesia (these recommendations can be found online at: www.asra.com). Chaney122 also has published an extensive review on the use of intrathecal and epidural anesthesia and analgesia for patients undergoing cardiac surgery.

Premedication
Anxiolysis, Amnesia, and Analgesia
The sedative and anesthetic-sparing actions of α2-adrenergic agonists (e.g., clonidine, dexmedetomidine) have been evaluated for their efficacy in several studies of CABG patients alone or in combination with a benzodiazepine.123–125 The use of α2-adrenergic agonists reduced stress response and anesthetic requirements compared with conventional regimens. A meta-analysis reported reductions in mortality and postoperative myocardial infarction with their use in cardiac surgery (started before surgery and often continuing until CPB).126 Nevertheless, some of the hemodynamic side effects of α2-agonists warrant caution in certain patient populations. Decreased HR, MAP, cardiac output (CO), contractility, and transiently increased systemic vascular resistance (SVR) seen with the less-selective α2-blocker clonidine, as well as an increased risk for hypotension during anesthesia induction, have been reported in general and cardiac surgery patients who were premedicated with α2-agonists.127,128 Consequently, α2-agonists should be used with caution in patients with preexisting severe bradycardia, conduction problems such as second- or third-degree heart block, hypovolemia, or hypotension.
Management of Antianginal and Antihypertensive Medications
The use of β-blocking agents in patients with poor ventricular function has been evaluated. Contrary to earlier reports,129 Kaplan et al130,131 reported in the mid-1970s and long before the ongoing discussion of perioperative β-blockade in noncardiac surgical patients that it was safe to continue β-blockade in patients presenting for cardiac and noncardiac surgery. Slogoff et al132 performed a randomized trial evaluating the safety of administration of propranolol within 12 hours of surgery. Based on a significantly greater increase in the incidence of pre-CPB ischemia in patients withdrawn from propranolol (within 24 to 72 hours), they also recommended continuation of therapy up until the time of surgery. Further work by these and other investigators in the 1980s documented the efficacy of β-blocker continuation through CABG surgery with regard to reducing pre-CPB ischemia and their superior efficacy over the increasingly popular calcium channel blockers133,134 (Box 18-2).
BOX 18-2 Preoperative Medication Management
The earlier mentioned studies were instrumental in laying the groundwork for the subsequent noncardiac surgery studies in the late 1980s.135–137 They led to the contemporary randomized trials of Poldermans et al138 and Mangano et al,139 which rallied support for routine perioperative β-blocker use; and the most recent conclusions drawn from the POISE trial140 that questioned parts of this practice. These trials resulted in the latest recommendations of β-blockade in patients undergoing noncardiac surgery.141 There is little doubt that β-blockers are beneficial for most CABG patients, particularly if HR is increased. When acutely administered in adequate dose, they significantly reduce myocardial oxygen demand and the incidence of atrial and ventricular arrhythmias. Several observational studies have documented associations of β-blocker therapy with reduction in perioperative mortality in CABG patients.142,143 The largest of these by Ferguson et al144 considered 629,877 patients in the STS database (1996 to 1999) in which a modest but statistically significant reduction in 30-day risk-adjusted mortality was reported. This treatment effect was observed in many high-risk subgroups, although a trend toward increased mortality was seen in patients with EF less than 30%. In a meta-analysis, Wiesbauer et al145 found that perioperative β-blockers reduced perioperative arrhythmias after cardiac surgery, but they had no effect on myocardial infarction or mortality. Considerable efforts are being expended by major organizations (STS, American College of Cardiology) in increasing compliance with existing guidelines for use of β-blockers at the time of hospital discharge (together with use of aspirin, statins, and angiotensin-converting enzyme [ACE] inhibitors).146,147
An increasing number of patients are presenting for CABG surgery while being treated with platelet inhibitors. Aspirin is a well-recognized component of primary and secondary prevention strategies for all patients with ischemic heart disease.148,149 Treatment with clopidogrel is required for coronary artery stent placement, has been shown to improve outcome, and is now recommended in combination with aspirin after acute coronary syndrome.150,151 Antiplatelet therapy is also used after CABG to reduce ischemic complications. Aspirin (and other platelet inhibitors such as dipyridamole) have long been recognized to have strong efficacy in the prevention of early graft thrombosis after CABG.152 Mangano et al,153 in a large observational analysis, reported substantial reduction in overall mortality rate (1.3% vs. 4.0%) and ischemic complications of the heart, brain, kidneys, and gastrointestinal tract in 5065 patients at 70 hospitals when aspirin was administered within 48 hours after surgery.
The combination of aspirin and clopidogrel after CABG may be even more effective.154 However, great controversy exists in regard to preoperative antiplatelet therapy. The risk for hemorrhagic complications needs to be weighed against the potential benefits of antiplatelet therapy. In a recent meta-analysis, patients receiving aspirin immediately before surgery had more mediastinal bleeding and received more blood products.155 Several retrospective studies have reported that CABG surgery in patients receiving clopidogrel is associated with increased bleeding and transfusion requirements.156 Filsoufi et al157 also reported longer ICU and hospital length of stay (LOS) and increased all-cause morbidity and mortality in clopidogrel-treated patients. In a multicenter, retrospective study, Berger et al158 reported that clopidogrel-treated patients were at increased risk for reoperation, major bleeding, and increased LOS.

Full access? Get Clinical Tree
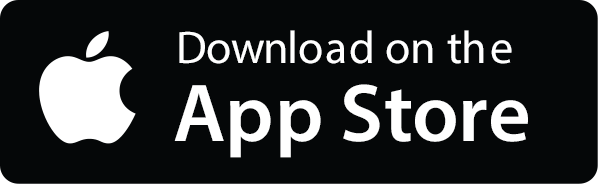
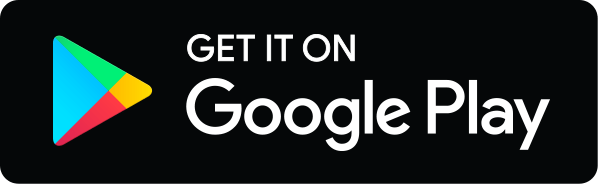