Standard 1: qualified anesthesia personnel shall be present in the room throughout the conduct of all general anesthetics, regional anesthetics and monitored anesthesia care
Standard 2: during all anesthetics, the patient’s oxygenation, ventilation, circulation, and temperature shall be continually evaluated (where “continuous” means without interruption and “continually” means repeated regularly and frequently)
Based on these principles, patients are monitored both by clinical observation (“look, listen, feel”) as well as by using specialized monitoring equipment (see Table 11.2). Most importantly, monitoring information of this kind can be useful in detecting various clinical problems. Some monitors (e.g., airway pressure) are usually built into the anesthesia machine. In addition, one should visually monitor the patient’s breathing pattern and color, look for signs of distress, etc.
Table 11.2
Monitoring equipment typically employed in general anesthesia cases
Electrocardiogram (provides information about rate, rhythm, ischemia (ST segments)) |
Blood pressure (manual, automatic, arterial catheter) |
Pulse oximeter (usually on fingertip or ear lobe) |
Capnograph (especially in patients with a supraglottic airway (e.g., LMA) or ETT) |
Oxygen analyzer (part of anesthesia machine) |
Anesthetic agent concentration analyzer |
Temperature (usually esophageal, nasopharyngeal or axillary) |
Precordial or esophageal stethoscope (listen to heart sounds, breath sounds) |
Gas flows/spirometry (part of anesthesia machine) |
Airway pressure monitor (part of anesthesia machine) |
Airway disconnect alarm (part of anesthesia machine) |
Peripheral nerve stimulator (where muscle relaxants have been used) |
Urometer (measure urine output – where appropriate) |
Blood Pressure Monitoring
Manual blood pressure monitoring is easily achieved via auscultation of Korotkoff sounds as learned by every medical student. However, automatic blood pressure monitoring is more practical and is generally achieved via a technique known as oscillometry . Here, the cuff is inflated to a high pressure, then deflated slowly. Oscillations in the cuff pressure begin to be detected when the cuff pressure first falls below systolic pressure. As deflation continues, the mean blood pressure is identified as the cuff pressure at which the amplitude of the oscillations is the greatest. The oscillations then vanish as the diastolic pressure is approached (see Fig. 11.1).


Figure 11.1
Sample blood pressure measurement using oscillometry. The peak oscillation corresponds to the mean arterial pressure (Reproduced with permission from Bronzino [15])
In most short cases, automatic blood pressure monitoring is done at least every 5 min. Many automatic blood pressure machines also have a “stat” mode where measurements are done immediately one after the other. Many anesthesiologists also put a manually operated blood pressure cuff on a second arm, in case the automatic blood pressure monitor fails to provide reasonable numbers, such may occur when the patient’s arms are tucked and the surgeon is leaning against the cuff. This second cuff is usually placed on the arm with the pulse oximeter; when it is inflated to the point that the pulse oximeter waveform is extinguished, the dial will indicate the patient’s systolic pressure. Alternatively, a second blood pressure cuff may be placed on a patient’s lower extremity.
In longer, complex cases or in very sick patients, invasive blood pressure monitoring via an arterial line is frequently utilized. Arterial lines are most often inserted into one of the radial arteries, although it may less commonly be placed in a brachial, femoral, ulnar, or dorsalis pedis artery. This method involves connecting an arterial catheter (usually a 20 gauge for adults) to a pressure transducer via a narrow fluid-filled tube (Fig. 11.2). This arrangement provides beat-to-beat pressure information that is helpful, for instance, in patients with poor ventricular function. In addition, since arterial blood gases are easily drawn from an arterial line, they can be particularly useful in patients with pulmonary disease or patients with acid–base disorders. Also, the ease with which blood samples can be sent for hemoglobin or glucose levels makes arterial lines useful in patients whose condition is changing rapidly over time or in which large blood losses are anticipated.


Figure 11.2
Arterial line waveform and its relationship to cardiac cycle (Reproduced with permission from www.rnceus.com/hemo/artline.htm)
Electrocardiographic Monitoring
All anesthetized patients undergo electrocardiographic monitoring. This provides the clinician with three types of information: (1) heart rate, (2) cardiac rhythm, and (3) information about possible myocardial ischemia (via ST segment analysis). In addition, ECG monitoring can help assess the function of a cardiac pacemaker.
The most common electrocardiographic system used during anesthesia is a five-electrode lead system. This arrangement (Fig. 11.3) allows for the recording of any of the six limb leads plus a single precordial (V) lead.


Figure 11.3
Location of the five electrodes used in a typical intraoperative electrocardiographic monitoring setup (Reproduced with permission from American Heart Association [16])
Pulse Oximetry
Pulse oximetry is a simple noninvasive method of monitoring arterial oxygen saturation (the percentage of hemoglobin (Hb) with oxygen molecules bound). The arterial saturation obtained in this manner is usually designated as SpO2. A pulse oximeter consists of a probe attached to the patient’s finger, toe, or ear lobe, which is in turn attached to the main unit (Fig. 11.4). It measures the red (e.g., 660-nm wavelength) and infrared light (e.g., 940-nm wavelength) transmitted through and/or reflected by a given tissue. In most units, an audible tone occurs with each heart beat which changes pitch with the saturation reading.


Figure 11.4
A typical pulse oximeter for operating room use, showing a SpO2 of 75 % and a heart rate of 60 beats/min. A waveform display allows one to ensure that a quality signal is present (Image courtesy Covidien)
A patient is generally said to be hypoxemic when the SpO2 falls below 90 %, a point usually corresponding to an arterial PO2 of 60 mmHg. An important advantage of using a pulse oximeter is that it can detect hypoxemia well before the patient becomes clinically cyanotic. Pulse oximetry is mandated in all patients undergoing anesthesia. However, it is important to realize that pulse oximeters give no information about the level of arterial CO2 and are useless in assessing adequacy of patent ventilation. Pulse oximeter units are now available for well under $1000. One design is even capable of providing hemoglobin information, a useful feature in surgical patients expected to undergo large blood losses.
Capnography and Ventilation Monitoring
Capnography (see Fig. 11.5a–e) is the continuous analysis and recording of carbon dioxide (CO2) concentrations in respiratory gases. Capnography is an ASA mandated monitor for both general anesthesia as well as for moderate sedation. A capnograph uses one of two types of analyzers: mainstream or sidestream. Mainstream units insert a sampling window into the breathing circuit for gas measurement, while the much more common sidestream units aspirate gas from the circuit and the analysis occurs away from the circuit. Capnographs may utilize infrared technology (most commonly), or other techniques such as mass spectroscopy, Raman scattering, or photoacoustic technology.


Figure 11.5
(a) Normal capnogram . The CO2 level at point D (end-tidal CO2) is normally around 40 mmHg. As shown above, the capnogram has four segments that correspond to phases of the respiratory cycle. The first phase (AB) is a flat part due to exhalation of dead space. It should always fall to zero; otherwise rebreathing is occurring. The second phase (BC) is the ascending segment from exhalation of mixed dead space and alveolar air. The third phase (CD) is the plateau portion that represents exhaled CO2 from the alveoli. The fourth phase (DE) represents the beginning of inspiration (Used with permission from Oridion Medical, Inc. www.oridion.com)(b) Hyperventilation . The end-tidal CO2 here is substantially less than 40 mmHg. Hyperventilation is sometimes used as a means to reduce intracranial pressure in head-injured patients (Used with permission from Oridion Medical, Inc.) (c) Hypoventilation . The end-tidal CO2 here is substantially greater than 40 mmHg. In this instance, this is due to a low respiratory rate, as might occur in a patient breathing spontaneously with opiate analgesics in use (Used with permission from Oridion Medical, Inc.) (d) Rebreathing . In this instance, the CO2 concentration never falls to zero. A common cause of this is exhausted CO2 absorbent (e.g., soda lime) in the anesthesia machine patient breathing circuit. This may be corrected by increasing the fresh-gas flow rate (Used with permission from Oridion Medical, Inc.) (e) A notch in the plateau (“a curare cleft”) is an indication of a spontaneous respiratory effort during mechanical ventilation
Capnography is useful in a number of important clinical situations:
Detecting when an anesthetic breathing circuit disconnects
Verification of endotracheal intubation (a sustained normal capnogram is not obtained when the endotracheal tube ends up in the esophagus)
Assisting in the detection of hypoventilation (raised end-tidal CO2 is often present) and hyperventilation (low end-tidal CO2 is often present)
Detecting rebreathing of CO2 (in which case the inspiratory CO2 level is nonzero)
Detecting capnograph tracings suggestive of COPD (where no plateau is present in the capnogram)
Monitoring CO2 elimination during cardiac arrest and CPR (the capnogram “improves” as pulmonary blood flow improves with adequate circulation)
Capnography is also important in monitoring ventilation in sedated or anesthetized patients, because mere observation of chest movement and (especially) skin color are often deceiving. Reliably estimating the degree of chest excursion by visual means is often difficult, and observation of cyanosis (dusky, bluish skin coloration) provides only a late warning of the presence of hypoxemia. By contrast, the use of capnography usually provides clinicians with reliable respiratory rate data and helps with the early detection of obstructed ventilation, hypoventilation, or apnea. Another point to remember is that since capnography measures ventilation, it will alert the caregiver to adverse ventilatory events well before a pulse oximeter signals an alarm. This is particularly true since patients receiving oxygen can have arterial saturation levels that are completely acceptable despite the patient having extreme hypercarbia.
Note that a sudden, severe decrease in end-tidal CO2 is sometimes due to a catastrophic cardiorespiratory event such as circulatory arrest, a large pulmonary embolus, or severe hypotension (such as from extreme blood loss or compression of the inferior vena cava).
In addition to capnography, mechanical ventilation can also be monitored through vigilant monitoring of tidal volumes (VT) and airway pressures. Tidal volumes may change as the patient’s condition changes – for example, when pressure-controlled ventilation mode is used, the VT is inversely related to total chest compliance (lung and chest wall). Nonpatient related factors, such as circuit leaks and partially inflated tube cuff, can cause a decrease in VT being delivered.
Airway pressures (peak and plateau) can also change secondary to patient factors. For example, when volume controlled ventilation is used, the airway pressures are inversely related to the total chest compliance. Nonpatient factors such as tube kinks and mucous plugs can cause an increase in airway pressures by increasing the resistance to gas flow.
Monitoring Muscle Relaxation
Muscle relaxation, or paralysis using neuromuscular blocking agents such as rocuronium is often required during surgery. For instance, muscle relaxation may be needed to facilitate tracheal intubation, to allow abdominal closure, or to ensure that no movement occurs during neurosurgery. In such settings, neuromuscular blockade monitoring or “ twitch monitoring ” is employed. This usually involves electrode placement at the ulnar or facial nerve, with use of a peripheral nerve stimulator (see Fig. 11.6). The nerve stimulators are usually used in one of two modes: A “train of four” (TOF) high-voltage stimulation pulses spread over 2 s (the more commonly used mode) or rapid tetanic stimulation at 50 (or sometimes 100) stimulation pulses per second. The observed “twitch” response to either stimulus sequence allows the anesthesiologist to determine the degree of muscle paralysis. In addition, neuromuscular blockade monitoring is usually used toward the end of the surgical procedure to assess both the suitability of the patient for reversal of muscle relaxation utilizing reversal agents such as neostigmine or sugammadex, as well as a few minutes later to access the degree to which reversal has been successful.


Figure 11.6
Proper electrode placement for stimulating the facial nerve (From O’Donnell and Nácul [17]. Used with permission)
Monitoring the Depth of Anesthesia
While monitoring muscle relaxation is easy using “twitch” monitors, measuring the degree of unconsciousness during general anesthesia is not. Some clinical techniques that anesthesiologists use to help gauge the depth of anesthesia include noting patient movements or grimacing, measuring the end-tidal anesthetic gas concentration, and following the blood pressure and heart rate trends. In addition to these classical methods, several electronic indices of brain function are available. Among these are the Bispectral Index , EEG Entropy, Patient State Index, and others. Among these, the Bispectral Index (BIS) (Fig. 11.7) is by far the best validated and most commonly used technique to monitor patient unconsciousness.


Figure 11.7
Bispectral index (BIS) monitoring system. Left: electrode assembly. Right: monitor showing a BIS score of 52 with the raw electroencephalogram shown on the upper right and the processed signal shown on the bottom

Full access? Get Clinical Tree
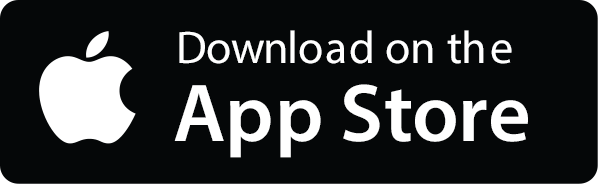
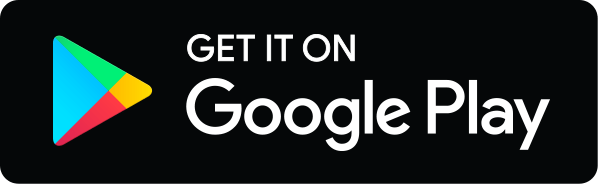
