Anemia in the Critical Care Setting
Marc S. Zumberg
Marc J. Kahn
Alice D. MA
General Principles
Anemia is common in the critical care setting. Recent studies have shown that 29% to 62% of patients have anemia at the time of admission to critical care units and 20% to 30% have moderate or severe anemia (hemoglobin < 9 g per dL) [1,2,3,4,5]. Anemia will develop in nearly all patients at some point during the course of a prolonged intensive care unit (ICU) stay, and as a result, the majority of patients admitted more than 7 days receive a red blood cell (RBC) transfusion [1,2,3,4,5].
Certain anemias may be encountered more frequently in patients who are admitted to critical care units than in other settings, including anemias arising from iatrogenic sources (e.g., mechanical hemolysis caused by ventricular assist devices or intra-aortic balloon pumps); those producing hemodynamic or systemic compromise that leads to a requirement for critical care (e.g., massive blood loss due to trauma, gastrointestinal lesions, or surgical invasion; thrombotic microangiopathies); and those arising in the context of prolonged critical illness (e.g., anemia of chronic disease/inflammation [ACD]). Losses from an enhanced frequency of phlebotomy for diagnostic testing in the critical care unit may contribute to the development or maintenance of anemia and have been estimated to account for 1 to 2 units lost during a typical hospital stay [5,6].
This chapter provides an overview of the evaluation and laboratory workup of anemia, with a focus on diagnoses that provoke the most clinical concern, are important to recognize quickly, and are the most likely to be encountered in the critical care setting. Accordingly, the hemolytic anemias, including the microangiopathic hemolytic anemias, autoimmune hemolytic anemia (AIHA), and sickle cell syndromes, will be covered in the most detail (Table 112.1). The ACD often develops in patients in the ICU and will also be a focus of this chapter. Anemia due to massive blood loss including trauma and gastrointestinal bleeding is essential to recognize, obtain proper consultation for, and treat appropriately, but the diagnosis is usually self-evident.
Initial Evaluation
The etiologies of anemia in the critical care setting are diverse, but the evaluation of anemia in a critical care patient initially should be approached in a manner similar to the noncritical care setting.
The patient’s volume status should be considered first, as an increase in the plasma volume may lead to a decrease in the measured hemoglobin or hematocrit that does not represent a decrease in the red cell mass or oxygen carrying capacity. This situation is known as dilutional or spurious anemia and is particularly common in ICU patients requiring fluid resuscitation [5]. Dilutional anemia does not require treatment.
To better come up with a differential diagnosis of the anemia, it should be determined whether the anemia predated the patient’s critical illness, developed in conjunction with the critical illness, or developed during the ICU stay (Table 112.2).
Table 112.1 Classification of the Hemolytic Anemias: Congenital Versus Acquired | |
---|---|
|
Laboratory Studies
Anemias can be classified by the size of the RBCs as reflected by the mean corpuscular volume (MCV): microcytic (MCV, < 80 fL), normocytic (80 to 100 fL), and macrocytic (> 100 fL). A finite number of diagnoses constitute each of these categories, allowing the practitioner to narrow the differential diagnosis (Table 112.3). One should take caution to review the MCV prior to the transfusion of RBCs, as donor RBCs may increase or decrease the MCV depending on the pretransfusion value.
Table 112.2 Sample Differential Diagnosis of Anemia Based on the Time Course of Anemia in Relation to the Critical Illness | |
---|---|
|
Table 112.3 Differential Diagnosis of Selected Anemias Based on Red Cell Mean Corpuscular Volume (MCV) | |
---|---|
|
Several additional tests may be helpful in the evaluation of anemia. The reticulocyte count, which is a measure of the bone marrow’s ability to produce new RBCs, should be the initial test performed. The reticulocyte count is typically elevated in hemolytic anemias, gastrointestinal bleeding, or after supplementation of a missing nutrient such as iron or vitamin B12. The reticulocyte count is typically low in primary bone marrow failure disorders, nutritional deficiencies, the anemia of chronic disease/inflammation, and any condition leading to the underproduction of or resistance to erythropoietin (e.g., renal disease). If a hemolytic anemia is suspected (i.e., due to consistently hemolyzed blood specimens, characteristic findings on physical examination [see later], or refractoriness to erythrocyte transfusion), measurement of total and unfractionated bilirubin (elevated), lactate dehydrogenase (LDH) (elevated), and haptoglobin (decreased) may be useful, although the results are not specific to hemolysis and may be similar in patients with advanced liver disease.
The blood smear itself may help to narrow the diagnosis and quickly identify anemias due to causes that require expeditious, specialized management (e.g., thrombotic microangiopathies). Examples of erythrocyte abnormalities include schistocytes (Fig. 112.1), sickle cells (Fig. 112.2), bite cells (Fig. 112.3), or spherocytes (Fig. 112.4) and identification of these aberrant forms is critical in making the correct diagnosis (Table 112.4).
Further laboratory testing should be guided by the results of the MCV, reticulocyte count, review of the blood smear, and any clinical suspicion of likely diagnoses (Table 112.5).
Therapeutic Red Cell Transfusion
Clinicians caring for patients in critical care settings are often confronted with the decision to transfuse RBCs even before results of laboratory testing or other evaluation has elucidated
the cause of the anemia. Erythrocyte transfusion in this setting may be guided by hemodynamic considerations, rather than a finite transfusion trigger [7]. Because of the (albeit low) risk of transmission of infectious pathogens and the potential for transfusion reactions and immunomodulation, and in light of increasing evidence from randomized trials that anemia is well tolerated in individuals without cardiopulmonary compromise, more restrictive transfusion policies are becoming more common [8,9,10,11,12]. Principles of transfusion are discussed in greater detail in Chapter 114.
the cause of the anemia. Erythrocyte transfusion in this setting may be guided by hemodynamic considerations, rather than a finite transfusion trigger [7]. Because of the (albeit low) risk of transmission of infectious pathogens and the potential for transfusion reactions and immunomodulation, and in light of increasing evidence from randomized trials that anemia is well tolerated in individuals without cardiopulmonary compromise, more restrictive transfusion policies are becoming more common [8,9,10,11,12]. Principles of transfusion are discussed in greater detail in Chapter 114.
Use of Erythropoiesis-Stimulating Agents
In multiple randomized clinical studies, use of erythropoiesis-stimulating agents (ESAs) in critically ill patients as compared with placebo or no intervention had no statistically significant effect on overall mortality, length of hospital stay, ICU stay, or duration of mechanical ventilation [13,14]. A recent meta-analysis, however, has shown that use of ESAs reduced the odds of a patient receiving at least one transfusion and modestly decreased the mean number of units of blood transfused by 0.41 units [13]. The optimal dosing and schedule of erythropoietin remains to be determined [13,15,16,17], and the need for concomitant supplemental intravenous iron, which may be considered when the serum ferritin drops below 100 to 200 ng per mL or iron saturation drops below 20% [2,15], still is debated. In a recent U.S. multicenter, retrospective, observational study of ESA utilization in anemic critically ill patients admitted to the ICU, practice patterns were highly variable [18]. Thus, at the present time, there remains insufficient evidence to recommend the routine use of ESAs in critically ill anemic patients [13].
![]() Figure 112.3. The RBC deformity (arrow) shown in this image is referred to as a “bite” cell. [Reused with permission from Lazarchick J. ASH Image Bank 2008;2008:8-00151.] |
![]() Figure 112.4. Spherocytes lack central pallor and may appear smaller than typical red cells. [Reused with permission from Maslak P. ASH Image Bank 2008;2008:8-00103.] |
Table 112.4 Selected Blood Smear Morphologic Findings Useful in the Evaluation of Hemolytic Anemia | ||||||||||||||||||||||
---|---|---|---|---|---|---|---|---|---|---|---|---|---|---|---|---|---|---|---|---|---|---|
|
Hematology Consultation
If the etiology of the anemia is not apparent despite the above evaluation or if treatment options remain uncertain, hematology consultation should be initiated. A bone marrow aspirate and biopsy may be useful if the diagnosis remains in question or if a primary bone marrow disorder is suspected due to unexplained abnormalities (morphologic or quantitative) in other blood cell lineages.
Hemolytic Anemias
The hemolytic anemias are characterized by a decreased red cell life span. The physiologic sequelae of these disorders, in addition to the ability of the hemolytic process to cause a decrease in hemoglobin and oxygen carrying capacity in a short period of time, may lead to a requirement for critical care. The patient with hemolysis may be very or only minimally symptomatic, depending on the rate of red cell destruction and the degree of compensation by the bone marrow, which produces young red cells (reticulocytes) in response to the decreased hemoglobin.
Overview of Laboratory Features
Pathologic features of hemolysis differ greatly depending on whether the red cell destruction is primarily intravascular or extravascular. Biochemical evidence for intravascular hemolysis includes elevated levels of LDH and unconjugated bilirubin and decreased levels of haptoglobin, which is cleared from the
circulation after binding free hemoglobin. Hemoglobinuria results when free hemoglobin is filtered through the glomerulus and is released into the urine, imparting a reddish color. Some hemoglobin in the urine is taken up by tubular cells and is converted to hemosiderin. This can be detected by checking for intracellular iron in the urine by staining the urine with Prussian blue stain. Extravascular hemolysis may be evidenced by only a declining hemoglobin level, although cases of brisk destruction of red cells may show elevations in LDH and unconjugated bilirubin.
circulation after binding free hemoglobin. Hemoglobinuria results when free hemoglobin is filtered through the glomerulus and is released into the urine, imparting a reddish color. Some hemoglobin in the urine is taken up by tubular cells and is converted to hemosiderin. This can be detected by checking for intracellular iron in the urine by staining the urine with Prussian blue stain. Extravascular hemolysis may be evidenced by only a declining hemoglobin level, although cases of brisk destruction of red cells may show elevations in LDH and unconjugated bilirubin.
Table 112.5 Suggested Initial Sample Laboratory Evaluation Based on the MCV and Reticulocyte Count | ||||||||||||||||||||||||
---|---|---|---|---|---|---|---|---|---|---|---|---|---|---|---|---|---|---|---|---|---|---|---|---|
|
Increased red cell production is evidenced by an increase in the number of circulating reticulocytes, which are young red cells whose large size typically results in an elevated red cell MCV and red cell distribution width. Circulating nucleated red blood cells (NRBCs) may be seen in cases of brisk hemolysis. Morphologic evidence of red cell destruction may be evident on the blood smear (see following sections and Figs. 112.1 to 112.4).
Immune-Mediated Hemolysis
The pathophysiology of immune-mediated hemolysis involves antibodies binding to red cells, with or without the activation of complement, leading to red cell destruction. If the antibody on the red cell surface is immunoglobulin G (IgG), then red cell destruction is mediated via Fc receptors on macrophages within the reticuloendothelial (RE) system. Complete or partial phagocytosis occurs causing the red cells to take a spherocytic shape as opposed to the normal, more pliable, biconcave disc shape.
Antibodies which lead to hemolysis can be divided into two categories: warm and cold, referring to the temperature at which the antibody optimally reacts with the red cell. Warm antibodies react with red cells best at temperatures 37°C and typically do not agglutinate red cells [19]. Cold antibodies typically react best at temperatures less than 32°C, with maximal reactivity at 4°C and lead to red cell agglutination [20]. The hallmark of AIHA is a positive direct Coombs test, which will detect the presence of either IgG or C3 bound to red cells (Table 112.6).
Warm Autoimmune Hemolytic Anemia
In warm autoimmune hemolytic anemia (WAIHA), IgG antibodies are directed against red cell surface membrane antigens [19]. Most commonly, these antibodies are directed against members of the Rh blood group, but the specificity of the pathogenic IgG antibodies is not always identified. The IgG antibodies coat the red cells and may or may not fix complement (C3). IgG-coated red cell membrane fragments are engulfed by macrophages in the RE system (usually the spleen) [19,21]. As the red cell loses surface area, it loses the ability to retain its biconcave disc shape. Since the shape with the smallest surface area-to-volume ratio is a sphere, the red cell becomes progressively more spherocytic with each pass through the splenic circulation [19].
Table 112.6 Interpretation of the Coombs Test and Differential Diagnosis | ||||||||||||||||||
---|---|---|---|---|---|---|---|---|---|---|---|---|---|---|---|---|---|---|
|
Table 112.7 Causes of Immune Hemolytic Anemias | |
---|---|
|
WAIHA can manifest as a primary disorder, or alternatively, it can be secondary to an underlying disorder, such as collagen vascular disease (e.g., lupus) or a lymphoproliferative disorder (e.g., lymphoma). Approximately 30% of patients with chronic lymphocytic leukemia have a positive Coombs test, although a much lower proportion develops hemolysis [22]. AIHA may be associated with immune thrombocytopenia, a condition called Evans syndrome. AIHA can also be provoked by infection or can be induced by various drugs. Causes of WAIHA are listed in Table 112.7.
Clinical Features
Almost all patients present with worsening and often debilitating fatigue. Older patients, and those with rapid hemolysis and ensuing severe anemia, may present with evidence of organ compromise such as dyspnea, angina, or syncope and can suffer myocardial ischemia, hypotension, and/or renal failure. Physical findings can include pallor, jaundice, and splenomegaly. Laboratory findings include an increased reticulocyte count, increased bilirubin (total and indirect), and increased LDH. The direct Coombs test should be positive (Table 112.6), and typically spherocytes, microspherocytes, NRBCs, and/or anisocytosis are seen on the blood smear.
Transfusion in Patients with Warm Autoimmune Hemolytic Anemia
If the patient has heart failure, angina, shock, or evidence of hypoperfusion to vital organs, or if compensatory erythrocytosis is absent or inadequate due to an underlying illness that suppresses the bone marrow, such as leukemia, prior chemotherapy, or renal failure, then red cell transfusion should be performed [19]. The anti-erythrocyte autoantibody itself also occasionally can be directed against red cell precursors in the marrow, leading to an inappropriately low reticulocyte count [19,23]. Any transfusion in patients with WAIHA needs to be coordinated closely with the blood bank or transfusion service. The offending antibody will frequently interfere with performing a crossmatch to identify compatible blood for transfusion. It is critical to obtain a thorough transfusion and pregnancy history to determine the likelihood of an underlying alloantibody which may be masked by the autoantibody; testing a red cell eluate may be helpful in this regard. Crossmatching can be done using low ionic strength solution (LISS) which
will minimize nonspecific interactions, allowing the stronger alloantibody interactions to appear. If time allows, phenotyping can be performed to identify any antigens on the patient’s red cells that may be likely to engender an immune reaction when exposed to transfused blood; such a maneuver may help to minimize the risk of a delayed hemolytic transfusion reaction (DHTR). If crossmatched units are not available, phenotypically matched red cells are preferred. If not available, due to time constraints or the patient’s condition, then ABO and Rh type-specific, noncrossmatched, or “least incompatible” units should be used. Each unit should be transfused slowly, while the patient’s clinical status is closely assessed for evidence of worsening hemolysis. The blood bank may require that samples of the patient’s blood be drawn soon after the transfusion begins to record any evidence of hemolysis. This is termed an in vivo crossmatch.
will minimize nonspecific interactions, allowing the stronger alloantibody interactions to appear. If time allows, phenotyping can be performed to identify any antigens on the patient’s red cells that may be likely to engender an immune reaction when exposed to transfused blood; such a maneuver may help to minimize the risk of a delayed hemolytic transfusion reaction (DHTR). If crossmatched units are not available, phenotypically matched red cells are preferred. If not available, due to time constraints or the patient’s condition, then ABO and Rh type-specific, noncrossmatched, or “least incompatible” units should be used. Each unit should be transfused slowly, while the patient’s clinical status is closely assessed for evidence of worsening hemolysis. The blood bank may require that samples of the patient’s blood be drawn soon after the transfusion begins to record any evidence of hemolysis. This is termed an in vivo crossmatch.
Treatment
After hemostatic instability has been addressed through transfusion of RBCs, the initial treatment of WAIHA consists of immunosuppression which, if successful, may attenuate antibody production and allow the patient’s RBCs to survive normally in the circulation. First-line therapy consists of glucocorticosteroids, either intravenously such as methylprednisolone or oral prednisone, typically at 1 to 2 mg per kg daily [19]. Intravenous immunoglobulin (IVIG) has also been used but is less effective than in immune thrombocytopenic purpura (ITP) [19,24]. If steroids are ineffective, or if relapse occurs, then alternative immunosuppression should be considered. Agents which have been reported to be useful in WAIHA include rituximab, cyclophosphamide, mycophenolate mofetil, and azathioprine [19,25,26,27]. Splenectomy should also be considered as a reasonable second-line treatment option in eligible patients [19]. As with all hemolytic anemias, the administration of folic acid 1 to 5 mg per day, at least as long as hemolysis is ongoing, is recommended. The reticulocyte count and complete blood cell count (CBC) should be followed closely to monitor the effectiveness of therapy. The amount of blood drawn may be minimized by using pediatric tubes or “bullet” tubes, if available.
Cold Agglutinin Disease
In cold agglutinin disease (CAD), immunoglobulin M (IgM) antibodies target red cell surface antigens, typically with specificity to either “I” or “i.” These IgM antibodies optimally bind to red cells at “cold” temperatures (typically < 32°C and most strongly at 4°C) [20], and, given their ability to bind more than one RBC simultaneously, lead to the agglutination and clumping of RBCs in the distal microvasculature. IgM anti-erythrocyte antibodies fix complement to the red cell, leading to either intravascular or extravascular hemolysis. CAD may be primary or secondary due to disorders such as lymphoproliferation or infection [28,29].
Clinical Features
In most patients, CAD is a chronic condition characterized by mild to moderate hemolysis and episodic cyanosis and ischemia of the ears, tip of the nose, and digits [29]. When episodic, cold-induced hemolytic episodes occur, intravascular hemolysis may be associated with shock, rigors, back pain, and renal failure.
Laboratory Evaluation
Cold-agglutinin titers can be measured. On Coombs testing, complement (C3) is typically positive while IgG is negative, reflecting the underlying IgM autoantibody which more efficiently fixes complement (Table 112.6). The thermal amplitude of the autoantibody, not the antibody titer, however, best determines the severity of clinical symptoms. If binding occurs only at 4°C to 30°C, it is less clinically important than if significant binding occurs at temperatures more than 34°C, approximating more physiologic conditions. In fact, many normal individuals will have cold agglutinins detected at 4°C but have no clinical symptoms.
Treatment
In patients with chronic, mild CAD, the mainstay of treatment is avoidance of cold temperatures. Corticosteroids and splenectomy are typically ineffective in CAD as compared with WAIHA. Other agents, such as chlorambucil, cyclophosphamide, and rituximab, have been used successfully [20,30]. In patients who present with impending or actual end-organ damage such as myocardial ischemia or stroke, plasmapheresis may be effective because IgM remains primarily intravascular and can be efficiently removed. Plasmapheresis may need to be performed preoperatively in surgeries requiring cardiopulmonary bypass or cardioplegia [20,31]. In all patients, care must be taken to keep the extracorporeal tubing warm and to warm intravenous fluids and blood products, or hemolysis may worsen. Folic acid repletion is recommended in all patients.
Paroxysmal Cold Hemoglobinuria
IgG is the pathogenic antibody in this rare condition. Similar to IgM antibodies in CAD, the IgG antibody in paroxysmal cold hemoglobinuria (PCH) binds to red cells only at cold temperatures where it fixes complement. Unlike the antibody in CAD, however, it is activated at warmer temperatures and does not agglutinate red cells. This antibody is called the Donath-Landsteiner antibody and is directed against the “P” red cell antigen [20]. Red cell destruction occurs primarily via activation of the complement cascade and leads to subsequent intravascular hemolysis. In the past, PCH was primarily a disease associated with tertiary syphilis and, therefore, has become much less common in the penicillin era. Currently, PCH is primarily a pediatric disorder (often following a viral infection), only rarely affecting adults. Patients suffer episodic, cold-induced hemolysis. There is no cold-induced digital ischemia.
The diagnosis is made by detection of the Donath-Landsteiner antibody. The Coombs test is typically negative for IgG and positive for C3 (Table 112.6). The blood bank should be alerted to the possibility of this diagnosis, as special considerations are required for detection. Serum is collected from the patient and kept at 37°C. Patient serum and normal red cells are next chilled to 4°C then warmed to 37°C. The presence of lysis is revealed by detection of free hemoglobin in the sample. Controls must be performed where red cells and serum are incubated at 37°C and in a separate test tube at 4°C. In both of these scenarios, there should be no lysis detected [32], as a positive test requires the extremes of temperature.
Drug-Induced Hemolytic Anemias
More than 130 drugs have been reported to cause immune-mediated hemolytic anemia [33]. Drugs can induce hemolytic anemia by three general mechanisms: the innocent bystander mechanism, hapten mechanism, and a true autoimmune mechanism [34,35]. These are described in Table 112.8. It should be noted that many drugs may lead to a positive direct Coombs test in the absence of overt hemolysis. Thus, a positive direct Coombs test should not be inferred to represent hemolysis unless there is worsening anemia in conjunction with consistent laboratory evaluation.
Other drugs may cause hemolysis by alternative mechanisms. Oxidant agents such as dapsone and other sulfa drugs may cause hemolysis in a dose-dependent fashion, especially in individuals with glucose 6-phosphate dehydrogenase (G6PD) deficiency who are impaired in their ability to detoxify the oxidant damage to hemoglobin (see “Glucose 6-Phosphate Dehydrogenase Deficiency” section later in the chapter). Ribavirin,
used to treat hepatitis C, causes hemolysis in a dose-dependent fashion. Its mechanism of red cell damage is unclear, but it may relate to nucleotide depletion. Other agents such as cyclosporine and tacrolimus may cause a microangiopathic hemolytic anemia due to endothelial damage (see section “Microangiopathic Hemolytic Anemia”).
used to treat hepatitis C, causes hemolysis in a dose-dependent fashion. Its mechanism of red cell damage is unclear, but it may relate to nucleotide depletion. Other agents such as cyclosporine and tacrolimus may cause a microangiopathic hemolytic anemia due to endothelial damage (see section “Microangiopathic Hemolytic Anemia”).
Table 112.8 Mechanisms of Drug-Induced Hemolysis | |||||||||||||||
---|---|---|---|---|---|---|---|---|---|---|---|---|---|---|---|
|

Full access? Get Clinical Tree
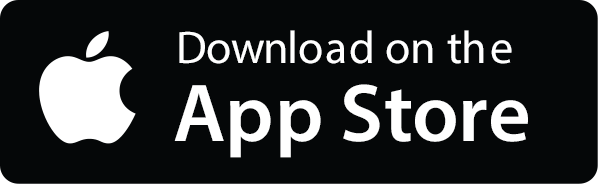
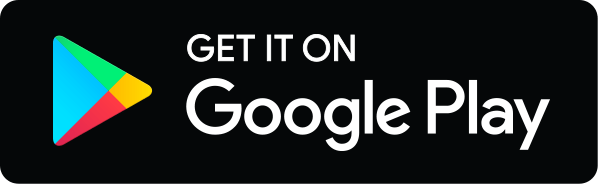
