Understanding the anatomy and physiology of pain transmission systems is important for the pain management specialist because it informs diagnostic and treatment decisions for the common pain syndromes for which patients seek help (e.g., diabetic neuropathy and migraine headache). Interventions to provide the relief the patient seeks (nerve blocks, implantable devices) are available at distinct anatomic sites. As ongoing research reveals new modalities and pharmaceutical agents to provide relief, the provider with this knowledge base will better understand their mechanism and application. Designed as an overview to be read in one sitting, the clinician will find it an efficient way to review the large body of knowledge acquired in medical school and residency.
This chapter reviews the transmission of a nociceptive or pain impulse from the site of stimulus in the periphery to the central nervous system. The basic anatomic pathways of nociceptive transmission and of descending nociceptive modulations are described. While some basic physiology is discussed, the fundamentals of neuronal transduction, such as action potential propagation and the relationship of the cell body to the dendrites and axon, are not. While mention is made here of pathophysiology and disease states, the details follow in the subsequent chapters.
This chapter focuses on the neuronal components of the nervous system that transmit and modulate nociceptive stimuli. Although attention is focused on primary afferents and descending pathways, interneurons in the dorsal horn of the spinal cord and at higher levels in the central nervous system play important roles in signal processing, transmission, plasticity, and, ultimately, in the way pain is experienced, including its emotional components. The complexities of chronic pain syndromes, including such theories as chronic pain as a variant of depressive disorders,1,2 are discussed elsewhere.
Knowledge of nociceptive transmission has advanced considerably since Descartes outlined his concept of the nerve as tubes with delicate threads to convey a painful impulse.3 It is now widely believed that stimulation of a primary afferent neuron in the peripheral nervous system results in patterns of activation of neurons in the dorsal horn of the spinal cord and then in transmission(s) rostrally to the brain.
Sensory neurons, called primary afferents, have a cell body in the dorsal root ganglia (DRG) of the spinal cord or in the ganglia of the cranial nerves. Cranial nerves V, VII, IX, and X receive inputs from primary afferents and, thus, sensory information from the head, face, and throat. Nerve cells in the DRG are a heterogeneous population of size and function—a reflection of the heterogeneity of the sensory inputs processed by the central nervous system.4 Central nervous system pain processing is also influenced by immune and surrounding glial cells.5
The classic nomenclature of peripheral neurons relies on the size of the axon and presence or absence of myelin as distinguishing characteristics. This taxonomy is referred to as the Erlanger-Gasser classification.6 There are three major groups, A, B, and C, in order of diminishing axonal diameter. Group A is further divided into four subgroups: Aα, or primary muscle spindle and motor to skeletal muscles; Aβ, which are cutaneous touch and pressure afferents; Aγ, which are motor to muscle spindles; and Aδ, which are mechanoreceptors, nociceptors, thermoreceptors, and sympathetic postganglionic fibers. Group B is sympathetic preganglionic fibers. Group C refers to mechanoreceptors, nociceptors, and thermoreceptors, as well as sympathetic postganglionic fibers.
Classification of primary afferents on the basis of size and morphology has been refined.4 Most pain management practitioners are familiar with the traditional classification and are acquainted with the terms Aδ and C fibers as pain transmission fibers. An alternative classification based on size of axon cross-section—type Ia/b, II, III, and IV, from large myelinated to smaller and more slowly conducting fibers—is not used in this.
Thus, the sensory neurons of greatest interest to the pain clinician are the Aδ and C fibers have cell bodies in the DRG and project to the dorsal horn of the spinal cord (or the equivalent nuclei for the cranial nerves).7 The morphology of the peripheral termination of the axon is a reflection of the neuron’s function: Mechanoreceptors end in specialized structures, whereas nociceptors have free endings. Although the majority of Aδ fibers respond to low-intensity mechanical, chemical, or thermal stimuli, the free nerve endings of the Aδ and C-type neurons likely allow a less specialized response to hazardous, potentially dangerous, and damaging stimuli.8 As a nociceptive stimulus persists, cells along the transmission pathway undergo change, which can lead to the formation of a chronic pain state.9 This chapter focuses primarily on the anatomy and physiology of early acute pain before nervous system structure is altered by a persisting pain state.
The nociceptive primary afferents supply all the areas of the body: skin and subcutaneous tissue, muscle, joints, the periosteum, and the viscera. The skin, subcutaneous tissue, and fascia are supplied by mechanical nociceptors (Aδ high-threshold mechanoreceptors), polymodal nociceptors (C-fiber nociceptors), and myelinated mechanothermal nociceptors (Aδ heat nociceptors).8 The latter respond to both noxious heat and intense mechanical stimuli, and these Aδ fibers are likely responsible for the first pain perceived after heat stimulation. This is in contrast to the high-threshold Aδ mechanoreceptors, which respond to repeated stimuli and not to the initial noxious stimulus of heat or cold and may be the first nociceptors involved in peripheral sensitization.10 The C-polymodal nociceptors, which also respond to high-intensity stimuli, whether mechanical or heat, respond by sensitizing to heat but, interestingly, fatigue in response to repeated mechanical stimuli.11,12
A brief, noxious stimulus that results in an immediate but transient epicritic sensation that is mediated by Aδ fibers also evokes the slow onset of a protopathic burning pain sensation that is C-fiber dependent.13
Muscle, fascia, and tendons are innervated by Aδ and C fibers. Activation of these neurons by noxious stimulation produces diffuse, aching pain that is poorly localized. Ischemic contraction of muscle may result in C-fiber activation. The muscle nociceptors are also activated by chemical stimulation.14,15 Joints are innervated by Aδ and C fibers, which, when activated in a normal joint, result in deep aching pain. In an arthritic joint or during acute inflammation, however, even normal and minimal activities result in pain.16 There has long been interest in the pathogenesis of arthritis and the interaction between inflammatory changes and the development of chronic pain.17
The periosteum of bone is acutely sensitive to noxious stimuli and nociceptors capable of releasing substance P are present in both cortex and marrow.18 Teeth are innervated by nociceptive and mechanical afferents. Both the inner and outer aspects of the tooth are innervated.19 Immunohistochemical labeling demonstrates that most dental primary afferents are C nociceptors, that Aδ fibers are present, and that a quarter of the fibers appear to be low-threshold Aβ mechanoreceptors capable of the mechanical allodynia responsible for tooth pulp hypersensitivity.20 The Aδ neurons projecting to the trigeminal nucleus are stimulated by noxious thermal stimulation of tooth pulp and may contribute to peripheral sensitization in pulpitis.21
The viscera are innervated by nociceptors as well as parasympathetic and sympathetic fibers, some of which may also be nociceptors. In contrast to cutaneous innervation, visceral structures are sparsely innervated so that few fibers respond to stimuli over a large area.22 Clinically, patients with visceral conditions are likely to complain of diffuse discomfort or poorly localized pain except under conditions of extremes (e.g., appendicitis, pleurisy). Very often, even extreme perturbations are interpreted with the less common pain descriptors such as discomfort, pressure, crushing, and squeezing. These patients may even reject “pain” as a descriptor (e.g., acute myocardial infarction). Convergence of deep somatic (muscle, joints) and visceral nociceptive information at higher midbrain structures23 and convergence of cutaneous and visceral projections contribute to the poor localization of noxious stimuli in the viscera.
The heart has Aβ, Aδ fibers, and C fibers24 involved in cardiovascular reflex responsiveness and nociception. The classic pain of myocardial ischemia—angina—was postulated to be mediated via chemical sensitization of afferents by substances released by the damaged cells (potassium, hydrogen ions) as well as humoral factors (bradykinin and prostaglandins).9,12 Cardiac nociceptors project as vagal afferents into the nodose ganglia25 and as sympathetic afferents into the superior cervical ganglion and dorsal horn of the spinal cord.26
The lungs and bronchi are also innervated by Aδ and C fibers. These fibers play a diverse role, serving to warn of irritants in the airway that are either mechanical or chemical. Furthermore, such distinct stimuli as pulmonary edema, embolism, and changes in oxygen tension may give rise to the sensation of dyspnea.
The abdominal viscera include such diverse structures as the stomach and intestines, gallbladder, and urinary bladder. Direct activation of nociceptors may occur; for example, hypersensitivity is noted in acid-sensing nociceptors present in lamina propria in nonerosive esophagitis.27 Transmission of acid-injury–related visceral nociceptor signals into the CNS can be enhanced by descending pathways from midbrain structures.28 Two common clinical manifestations of visceral pain states are chronic pelvic pain and interstitial cystitis. Both syndromes may be disabling to patients and are frustratingly difficult to treat. Both myelinated and unmyelinated sensory fibers project from the bladder to the lumbar and sacral dorsal horn of the spinal cord, and immunohistologic studies have identified their possible role in pain transmission.29 Mechanoreceptors transmitting stretch/distention may also become sensitized by local bladder irritants, especially in the setting of erosive tissue damage.29
Nociceptors, sensitive to diverse extracellular signals and able to project with release of an array of molecular signals,30 are but the first in the highly integrated systems of neuronal, glial hormonal, and inflammatory cells that process their signals.
The primary afferents, which have their cell bodies in the DRG, extend from the periphery to terminate centrally in the dorsal horn. The fibers that enter the dorsal horn are segregated by size as they form small bands or rootlets and approach the dorsal horn. Lissauer observed that the smaller fibers are segregated laterally and then terminate in the first layers of the dorsal horn. This area has come to be known as Lissauer’s tract. There are clinical applications of knowledge of the anatomy of the dorsal horn and dorsal root entry zone. Neurosurgeons may perform a selective posterior rhizotomy in the hopes of ablating otherwise intractable, unrelenting chronic pain. These techniques are discussed further in the chapters on clinical pain management.
It is important to note that many cells in the DRG have more than one process.31 Thus, a fiber may receive input from two distinct areas, such as the dura mater and part of the face. The connections that appear to subserve sensory processing during migraine, for example, are complex.32 The terminal connections may also branch and innervate multiple spinal levels.33 There is also evidence that sensory fibers may travel in the ventral roots, as well. There are certainly small unmyelinated fibers in ventral tracts. Thus, the anatomy is more complex than a simple schema can represent. These complexities may explain why selective posterior rhizotomy is, clinically, rarely successful in providing complete relief of unrelenting pain. But, as understanding of the peripheral nervous system grows, so does an appreciation of its complexity. Injury to peripheral nerves may result in chronic changes. Initial injury and acute changes may develop into chronic ectopic inputs, which may induce a state of central sensitization and structural reorganization of dorsal horn synapse.10 Thus, there are profound physiologic reasons why a surgical anatomic approach to pain management may not be sufficient.
The majority of primary afferents terminate in the ipsilateral dorsal horn. The process of the spinal neurons bifurcates into an ascending and a descending branch, thus innervating the spinal cord over several spinal segments. Some processes travel dorsal to the central canal to end in the contralateral dorsal horn. The terminus of each primary afferent is the reflection of its function because the dorsal horn neurons are segregated by physiology. Rexed divided the gray matter of the spinal cord into ten laminae.34 This classic cytoarchitectural segregation yields six subdivisions of the dorsal horn (laminae I through VI) and three subdivisions of the ventral horn (laminae VII through IX.) Lamina X describes the column of cells around the central canal.
The dorsal laminae of the spinal cord run its entire length and, in the medulla, become the medullary dorsal horn. The marginal layer of the dorsal horn refers to lamina I. Lamina II, or the substantia gelatinosa, is subdivided into outer and inner areas (IIo and IIi). The substantia gelatinosa is of interest to clinicians and researchers.35 Laminae III through V are referred to as the nucleus proprius or the magnocellular layer.
Dorsal horn neurons, which process nociceptive information, are a heterogenous population. Laminae I and V have nociceptive specific neurons. There are two populations of nociceptive-specific neurons. One type receives inputs from Aδ fibers, both high-threshold mechanoreceptors and temperature-sensitive receptors and from polymodal C fibers. The second type of nociceptive-specific neuron appears to receive inputs only from high-threshold Aδ mechanoreceptors. The other neuron type that processes nociceptive input is the wide-dynamic-range (WDR) neuron. Found predominantly in lamina V (and to a lesser degree in lamina I), the WDR neuron receives input from Aδ fibers of both high-threshold mechanoreceptors and temperature-sensitive neurons and also from polymodal C fibers, as well as from Aβ low-threshold mechanoreceptors.32
The physiology of the dorsal horn explains the heterogeneity of function. Lamina I cell projection pathways have been shown to be concerned with long-latency, long-duration reactions to prolonged events, rather than to brief stimuli.33 Lamina I neurons, which project via the spinothalamic tract, are an integral component of the central representation of pain and temperature. These nociceptive-specific neurons, both the high-threshold mechanoreceptors and the temperature-sensitive polymodal C fibers, are inhibited by morphine in a dose-dependent manner.36,37 This suggests that opiate-modulation of nociceptive transmission is functionally organized. Both substance P and neurokinin A are released by neurons in the substantia gelatinosa when a noxious impulse is transmitted.38 Somatostatin is released following noxious thermal but not noxious mechanical stimuli, which implies some encoding of information by the dorsal horn.10
The fibers of greatest interest to pain clinicians are Aδ and C fibers. The Aδ nociceptors terminate in laminae I and IIo and have collateral branches that terminate in laminae V and X. Similarly, the C fibers, which enter the spinal cord via the lateral aspect of Lissauer’s tract, also terminate in laminae I and IIo, as well as lamina V. The termination of the large, myelinated Aβ fibers is in laminae III and IV, as well as lamina V.34
Following an injury, however, there may be marked alterations in the cytoarchitecture of the dorsal horn. Woolf reported that following nerve injury, large-diameter fibers may invade laminae I and IIo.10 This may provide a mechanism to explain the clinical finding of allodynia and the physiologic alterations in neuropathic pain syndromes.10,39,40 This subject is discussed in detail in subsequent chapters.
The information conveyed by the primary afferents travels first to the dorsal horn and then from the dorsal horn rostral via the ascending tracts.41 A variety of neurotransmitters are used to convey noxious information.37,42 There are distinct areas of dopamine-containing neurons.43 The peptide substance P is found in the dorsal horn.44,45 There is evidence that glutaminergic neurons are also involved, as well as neurons that respond to γ-aminobutyric acid (GABA), although these neurons are not as prevalent.46 A segmental chronic pain syndrome can be induced in rats by an intrathecal infusion of N-methyl-D-aspartate (NMDA)47 or by amputating large mylenated neurons at the dorsal root ganglia.48
New possibilities for targeting specific receptors to provide relief from pain continue to generate a great deal of excitement from clinicians. In 2013, Gohlke and colleagues performed data mining of the entire Medline/PubMed NBCI FTP database, using computational linguistics on titles and abstracts, sometimes on full texts, to identify possible receptors and ligands associated with pain transmission; built a web engine to link the human-reviewed results with molecular databases (BindingDB and PubChem); and designed a user-friendly graphical and text interface to search among 8700 molecules likely to increase or decrease pain or 100,000 that may possibly have an effect.49 The website and database, known as “SuperPain,”50 allows sharing and adapting content for noncommercial purposes.
The spinothalamic tract (STT) and the trigeminothalamic tract transmit primarily pain and temperature information.51,52 Neither tract, however, transmits exclusively noxious stimuli: The tracts are heterogenous and transmit some innocuous stimuli such as light touch, as well.53 Furthermore, it is now accepted that other ascending tracts also convey noxious information, as well.54–56
The lateral and ventral STTs travel in the anterior lateral quadrant of the spinal cord. The spinomesencephalic tract (SMT) is located in the anterior lateral quadrant and in the dorsolateral funiculus.52 The dorsal column postsynaptic spinomedullary system is a second-order dorsal column pathway that is located appropriately in the dorsal column.57,58 This propriospinal multisynaptic ascending system is a more complicated network of short chains of neurons, which may play a role in nociceptive transmission.57,59

Full access? Get Clinical Tree
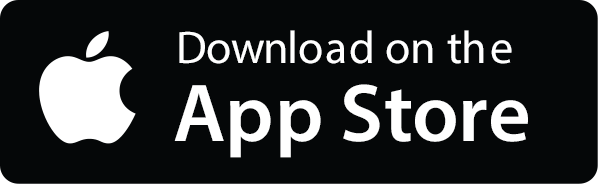
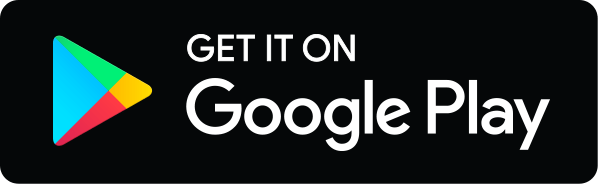