Chemical structure of opioids
The presence of the tertiary nitrogen and its distance from the aromatic ring (4.55 Å for morphine and the related semisynthetic compounds) is considered to be essential for activity. The rigid pentacyclic (phenanthrene) frame of the morphine molecule has a three-dimensional structure in which rings A and B are coplanar and rings C and E are perpendicular to the AB plane. This configuration is common to many compounds with opioid activity. The flat benzene ring (A) lies against the flat part of the receptor, while the piperidine ring (E) fits into a groove in the receptor, to which it is attracted by virtue of its positively charged nitrogen atom.
Substitutions of functional groups on the rings or the core morphine molecule produce opioids with different pharmacological properties.
On the tertiary amine N-17, a short-chain alkyl substitution results in mixed agonist–antagonists such as buprenorphine and nalorphine. Hydroxylation of the C-14 in addition to this converts these into full antagonists. Naloxone is one such antagonist, produced by hydroxylation of nalorphine and modification of the C-6 hydroxyl group to double-bond oxygen. Removal of the methyl group (on the tertiary amine N-17) or replacing it with certain alkyl groups markedly reduces opioid agonist activity.
The phenolic group at C-3 probably interacts with the receptor, since the presence of a hydroxyl group maximises potency, while substituting it with a methyl to make codeine decreases potency 10 times. Longer alkyl side-chain substitutions produce even weaker agonists. Similar alkyl substitutions on C-1 also reduce potency.
C-6 substitutions with lipophilic groups increase activity. Oxidation of C-6 increases potency and the double acetyl substitution at C-3 and C-6 to produce diamorphine increases potency by increasing lipophilicity, despite the competing effects of the dual substitution.
Alterations to the pentacyclic structure of morphine produce the synthetic opioids. Removal of the oxide bridge between rings A and C produces the morphinans, which are generally more potent. Removal of the C ring from the morphinans creates the benzomorphan group. Removal of the methylene bridge from the benzomorphans produces the phenylpiperidine structure of which pethidine is the prototype. The molecule unfolds such that the tertiary nitrogen is now further from the aromatic ring. The phenylpiperidines are all developed from modifications to the structure of pethidine, with sufentanil being a thienyl derivative of fentanyl.
Opioid receptors
Opioid receptors are G-protein-coupled transmembrane receptors. These exist throughout the CNS, with particularly high concentrations in the periaqueductal grey area and the substantia gelatinosa of the spinal cord. They are also present outside the CNS, and this may account for some of the other opioid effects (gastrointestinal) and their postulated value in some peripheral anaesthetic techniques (such as intra-articular injection).
Classification
There have been a number of schemes used to define the various opioid receptor groups. These have in the main been merely new names for the same groups. Three main groups have been classified, to which a fourth (the nociceptin/orphanin receptor) has been added. The grouping has been defined by their response to different prototype agonists. The various classifications are shown in Figure 31.4. The current International Union of Basic and Clinical Pharmacology (IUPHAR) nomenclature for the opioid receptors is MOP (μ, OP3), KOP (κ, OP2), DOP (δ, OP1) and NOP (OP4). The OPx type classification can lead to confusion once the receptors are subdivided. Separate genes for each class of opioid receptor have now been identified.
Classification | Receptors | |||
---|---|---|---|---|
MOP | DOP | KOP | NOP | |
μ | δ | κ | ||
OP3 | OP1 | OP2 | OP4 | |
Subtypes | μ1, μ2 | δ1, δ2 | κ1, κ2, κ3 | |
Prototype agonist | morphine | Ala-leu-enkephalin | ketocyclazocine | |
Endogenous ligand | Leu-enkephalin | Leu-enkephalin | dynorphin | nociceptin |
Met-enkephalin | Met-enkephalin | β-endorphin | orphanin FQ peptide | |
β-endorphin | β-endorphin |
MOP
MOP receptors open potassium ion channels, causing hyperpolarisation and reduced neuronal firing. At the nerve terminal the action potential plateau shortens, thus reducing calcium ion influx and neurotransmitter release. MOP receptors are located in the:
Primary afferent neurones (pre- and postsynaptic)
Peripheral sensory neurones
Periaqueductal grey matter
Nucleus raphe magnus
Rostral ventral medulla
Thalamus
Cerebral cortex
DOP
DOP receptors also open potassium ion channels, causing hyperpolarisation and reduced neuronal firing. At the nerve terminal the action potential plateau shortens, thus reducing calcium ion influx and neurotransmitter release. DOP receptors are located in the:
Olfactory bulb
Cerebral cortex
Primary afferent neurones (presynaptic)
Motor integration areas
Nociception areas
KOP
KOP receptors, in contrast, directly close calcium channels, so that the end result is reduced neurotransmitter release. KOP receptors are found in the:
Hypothalamus
Nociception areas
NOP
NOP receptors directly close calcium channels, so that they too result in reduced neurotransmitter release. NOP receptors are located in the:
Nucleus raphe magnus
Primary afferent neurones
Sigma receptor
Following the original discovery of μ, δ and κ subtypes, a fourth variant, σ, was originally classified as an opioid receptor. The selective agonist N-allyl-normetazocine (SKF 10047) had been shown to produce mydriasis, tachypnoea, tachycardia and delirium after occupancy of this receptor. The σ receptor is no longer classified as an opioid receptor, as it does not meet the full criteria. Notably:
It has a high-affinity binding for phencyclidine and related compounds.
The σ-mediated effects are not reversed by naloxone.
σ receptors are stereospecific for dextrorotatory isomers.
The σ receptor is not affected by opioids but is bound to by phencyclidine and haloperidol.
Pharmacodynamics
All opioid agonists share a similar pharmacodynamic profile, and demonstrate a sigmoidal dose–effect relationship. In addition to the variety of exogenous opioid agonists, there are a number of endogenous agonist ligands which act on opioid receptors. Many of these agonists lack specificity for a particular subtype of receptor, while the mixed agonist–antagonists exhibit agonist, partial agonist and antagonist actions on the various receptor subtypes (Figure 31.5).
Actions | Receptors | ||
---|---|---|---|
MOP | DOP | KOP | |
OP3 (μ) | OP1 (δ) | OP2 (κ) | |
Analgesia: supraspinal | ++ | ++ | ++ |
Analgesia: spinal | ++ | ++ | ++ |
Respiratory depression | ++ | ++ | + |
Miosis | ++ | ++ | ++ |
Gastrointestinal motility decreased | ++ | ++ | 0 |
Smooth muscle | ++ | ++ | 0 |
Behaviour | Euphoria | Euphoria | Dysphoria |
Sedation | ++ | ++ | + |
Physical dependence | ++ | ++ | + |
Other | Diuresis |
Each of the opioid receptors arises from a separate gene. Opioid receptors belong to the superfamily of serpentine receptors, which contain seven membrane-spanning domains, with an extracellular N-terminus and an intracellular C-terminus. The transmembrane helices are arranged anticlockwise, forming a tight helical bundle. Importantly, the N-terminal and C-terminal tails, and the second and third extracellular loops, are responsible for ligand binding specificity, and for the differential regulation of and variation in coupling to effector systems. The four classes of opioid receptors all produce primary inhibitory effects following activation, via receptor coupling to inhibitory G proteins. This results in inhibition of adenylyl cyclase, with reduced formation of cyclic adenosine monophosphate (cAMP), closure of voltage-sensitive calcium channels, stimulation of inwardly rectifying K+ channel opening causing membrane hyperpolarisation, and stimulation of phospholipase Cβ. Any excitatory or facilitatory effects of opioids can be explained by the inhibition of inhibitory pathways. Figure 31.6 summarises opioid receptor classification and molecular coupling mechanisms.
Receptor | Subtypes | Location | Endogenous ligand | Clinical effect |
---|---|---|---|---|
MOP (μ) | μ1, μ2 | Pre- and postsynaptic on primary afferent neurones and peripheral sensory neurones Periaqueductal grey matter Nucleus raphe magnus Rostral ventral medulla Thalamus Cortex | β-endorphin Leu-enkephalin Met-enkephalin Dynorphin | Supraspinal analgesia (μ1) Sedation (μ1) Spinal analgesia (μ2) Respiratory depression (μ2) Bradycardia (μ2) Hypothermia (μ2) Miosis (μ2) Euphoria (μ2) Pruritis (μ2) Nausea and vomiting (μ2) Constipation (μ2) Urinary retention (μ2) Physical dependence |
DOP (δ) | δ1, δ2 | Olfactory bulb Cerebral cortex Pre-synaptic on primary afferent neurones, motor integration areas and nociception areas | Leu-enkephalin Met-enkephalin | Supraspinal analgesia (δ1) Spinal analgesia (δ1) Respiratory depression Constipation Physical dependence |
KOP (κ) | κ1, κ2, κ3 | Hypothalamus Nociception areas | Dynorphin | Supraspinal analgesia (κ3) Sedation Spinal analgesia (κ1) Dysphoria Psychotomimetic effects ↓ vasopressin + diuresis |
NOP | Nucleus raphe magnus Primary afferent neurones | Nociception/orphanin FQ peptide | Supraspinal anti-analgesia Spinal anti-analgesia and analgesia Urinary retention |
Stimulation of the NOP receptor may produce either a pro-nociceptive antanalgesic effect (supraspinal and spinal) or an antinociceptive effect (spinal – at high concentrations). The function of this receptor is thought to be in setting pain thresholds and in the formation of tolerance to opioid therapy, although tolerance also develops to its antiopioid effects. Importantly, NOP receptor antagonists produce analgesia, prevent the development of tolerance to opioid analgesia, and may be useful for reducing some of the side effects associated with opioid therapy.
Clinical effects
Opioid agonists cause a range of mainly depressant and some stimulant actions on the CNS via specific receptors. They have little capacity to produce amnesia, have no intrinsic anticonvulsant activity and do not alter seizure threshold.
In anaesthesia, opioids are used for the production of analgesia and the maintenance of haemodynamic stability. Systemically administered opioids produce analgesia through actions at two anatomically distinct regions: supraspinal and spinal sites. They reduce the intensity of pain and the associated fear. This is achieved by raising the pain threshold, altering the reaction to pain, and inducing sleep and hypercapnia. Their efficacy is greater for continuous dull pain rather than sharp intermittent pain. They are less effective for the treatment of neuropathic pain.
CNS effects
Figure 31.7 lists the various effects of opioids on the CNS. Opioids cause a reduction in level of consciousness and eventually produce sleep, with the loss of responsiveness to verbal command. They produce a dose-related decrease in the MAC for volatile anaesthetics, with a ceiling of 60–70% reduction in MAC. They also increase cerebral vasoconstriction in the presence of vasodilating agents such as volatile anaesthetics. They do not cause a loss of cerebral autoregulation or reactivity to CO2. A ceiling effect is reached on the EEG, with a slowing of EEG frequency and the production of high-voltage (δ) waves.
System | Effect | |
---|---|---|
Musculoskeletal | Gait | ↓ Physical performance Ataxia ↓ Spinal cord reflexes |
Rigidity | Rigidity occurs 60–90 seconds post-injection and abates spontaneously after 10–20 minutes Mainly thoracoabdominal and upper limb musculature, ↑ with ↑ age, ↑ speed of injection, ↑ dose, use of N2O Mediated via nucleus raphe magnus | |
Multifocal myoclonus | Non-seizure related, ↑ with pethidine | |
Neural | Central | Spectrum from abnormal eye movement, to contraction of extremities, to tonic–clonic movements Euphoria: especially for agents which cross the blood–brain barrier quickly Dysphoria in some individuals Subjective feelings of body warmth and heavy extremities Apathy ↓ Level of consciousness and eventually sleep ↓ Concentration and capacity for complex reasoning |
EEG | Effects vary between different opioids: slowing of frequency, production of high-voltage δ waves No capacity to produce EEG silence | |
Cerebrovascular | SSEPs | No effect on SSEPs |
ICP | No effect | |
CBF | No effect, but ↑ vasoconstriction with vasodilators | |
No loss of autoregulation or CO2 reactivity CMRO2 reduced by up to 10–25% | ||
Vision | Edinger–Westphal nucleus | Miosis (via a ↓ in inhibition to the nucleus) except pethidine. Reversed by hypoxia and atropine |
Thermoregulation | Peripheral effects | Promote hypothermia via ↓ BMR (10–20%), venodilatation, muscle relaxation |
Response | ↓ Thermoregulatory responses (as for volatile agents) |
Cardiovascular effects
Opioids preserve cardiovascular stability to a greater extent than most other anaesthetic drugs. In normovolaemic patients they produce little perturbation in haemodynamic parameters, with minimal cardiac depression, no baroreceptor inhibition and a modest reduction in preload or afterload. Haemodynamic compromise may, however, be observed in individuals whose cardiovascular integrity is dependent on a high level of sympathetic tone, because opioids reduce central sympathetic outflow when even small doses can cause hypotension or cardiovascular collapse. Morphine has the greatest effect on the vascular system, owing to its propensity to cause histamine release and subsequent indirect effects on catecholamine release. This may result in a tachycardia with a reduction in systemic vascular resistance (SVR) and mean arterial pressure (MAP). The risk can be attenuated by pretreatment with histamine receptor antagonists and volume loading.
Opioids generally produce a negative chronotropic effect via central vagal excitation. Pethidine, however, has a homology with atropine and can produce a tachycardia, and it is the only opioid to produce significant direct myocardial depression when used at high doses. Myocardial depression is not observed unless extraordinarily high doses of morphine or the fentanyl congeners are administered. Morphine has indirect positive inotropic effects at doses of 1–2 mg kg–1, and blocks neurally and hormonally mediated venoconstriction to reduce preload, making it useful in the treatment of acute congestive heart failure.
Effects on other organ systems
The effects of opioids on the cardiovascular system and other organ systems are summarised in Figure 31.8.
System | Effect | |
---|---|---|
Cardiovascular | Heart rate | Usually sinus bradycardia via central vagal excitation Occasionally sinus arrest Exacerbated by concomitant vagal stimuli (e.g. laryngoscopy) and β-blockers |
Mean arterial pressure | Usually no effect or a slight ↓ (unless significant bradycardia) Greater ↓ associated with histamine release | |
Vascular tree | No effect on SVR (unless histamine release) Mild venodilatation with a ↓ in preload (due to ↓ central sympathetic outflow | |
Myocardium | No effect on contractility (except for pethidine which is a depressant) No effect on metabolic rate Possible ischaemic preconditioning | |
Excitability | ↓ Myocardial conductivity, ↑ Refractory period, ↑ VF threshold | |
Respiratory | Mechanics | Equivalent ↓ in rate, tidal volume and minute ventilation at equianalgesic doses ↑ Pauses, irregular breathing and apnoeas |
Control | ↑ Apnoeic threshold ↓ CO2 sensitivity ↓ Carotid body chemoreception and hypoxic drive Voluntary control of respiration remains intact No effect on hypoxic pulmonary vasoconstriction | |
Airway reflexes | ↓ Airway reflexes with improved tolerance of ETT Antitussive via peripheral and central actions ↓ Mucociliary function Brief cough in up to 50% with bolus phenylpiperidines | |
Gastrointestinal | Stomach and intestines | ↓ Peristalsis and secretions, and ↑ tone causing dry stool and constipation ↓ Gastric acid ↓ Gastric emptying with ↑ antral tone and ↓ LOS tone promoting ↑ aspiration risk ↑ Tone of pyloric, ileocaecal, and anal sphincters |
Biliary tree | ↑ Bile duct pressure Sphincter of Oddi contraction (little clinical significance overall) | |
Chemoreceptor trigger zone | Nausea and vomiting (worse with first dose and ↑ dose interval) Worse postprandial due to concomitant ↑ gastric antral tone | |
Genitourinary | Kidney | Antidiuresis as a result of ↓ RBF and ↓ GFR (predominates) ↓ Vasopressin release in response to osmotic stimuli |
Bladder | ↑ Bladder and urethral tone Vesicular sphincter contraction | |
Immunity | Immune system | ↓ Immunoglobulin production (uncertain significance) Reactivation of herpes simplex virus 2–5 days after neuraxial opioid |
Opioids are the most efficacious of all anaesthetic drugs for attenuating the stress response associated with pain, laryngoscopy and airway manipulation. The plasma concentrations of stress hormones (catecholamines, cortisol, vasopressin, aldosterone and growth hormone) increase during anaesthesia and surgery in proportion to the degree of operative trauma. This contributes to increased myocardial work, tissue catabolism and hyperglycaemia – responses associated with increased perioperative morbidity and mortality. Opioids reduce nociception, inhibiting the pituitary–adrenal axis, reducing central sympathetic outflow and influencing centrally mediated neuroendocrine responses. Fentanyl and its congeners (particularly sufentanil) are the most efficacious, but no opioid is completely effective.
Side effects
The organ-specific adverse effects of opioids observed in an individual depend on age, extent of disease, presence of organ dysfunction, concurrent administration of certain drugs, prior opioid exposure and the route of drug administration. Not all adverse effects are deleterious. A number of opioid-induced side effects are produced by activation of opioid receptors either centrally or peripherally, or in both areas. Serious allergic reactions to opioids are exceedingly rare, although anaphylaxis has been reported.
At equianalgesic doses all opioids produce equivalent degrees of respiratory depression, with the elderly and neonates being at increased risk. Tolerance develops rapidly to this effect, and with chronic opioid exposure the risk of significant respiratory depression is reduced. Apnoea may occur in conscious patients, but is usually associated with other signs of CNS depression, and apnoeic patients can be instructed to breathe as voluntary control of ventilation remains intact. Sleep, or the concomitant use of other CNS depressants (except clonidine), potentiates the risk by further reducing sensitivity to CO2.
Opioid-induced depression of airway reflexes is usually regarded as an advantageous side effect, although the impairment of mucociliary function is not. All opioids possess antitussive activity at less than analgesic doses, acting via central and peripheral mechanisms (opioids that do not cross the blood–brain barrier also depress coughing, e.g. pholcodine).
The incidence of nausea associated with opioid use is 10–60%, and this is markedly increased in pain-free and ambulatory patients (via opioid sensitisation of the vestibular nucleus). The ability to produce nausea and vomiting varies between opioids, and also between patients, but tolerance develops rapidly. Substituting one opioid for another may decrease the incidence, as may switching to oral administration.
Constipation remains the most common side effect of chronic opioid administration, and toxic megacolon may occur in patients with ulcerative colitis. Tolerance to this and other smooth muscle effects develops very slowly. Loperamide is a synthetic opioid which does not cross the blood–brain barrier, and it is used specifically as an antimotility agent. All opioids increase bile duct pressure and cause contraction of the sphincter of Oddi in a dose-dependent manner. Pethidine has been incorrectly reported to cause less smooth muscle spasm than the other full agonists, and is equally spasmogenic at equianalgesic doses. Opioid effects on the biliary tree can be reversed by naloxone (except for pethidine, which produces any smooth muscle contraction via a direct action), nitroglycerine or glucagon.
Other smooth muscle effects involve the genitourinary system. Urinary retention and urgency occur frequently, and are more common in the elderly, and when opioids are administered neuraxially. This latter situation reflects a centrally mediated mechanism through receptors located in the sacral spinal cord.
There are a number of other centrally mediated opioid effects. The majority of these are of no clinical benefit and usually unpleasant. Opioids may cause pruritis with a spectrum of severity, and the mechanism and physiological significance are unknown. The pruritis predominantly affects the face and nose, and is independent of any histamine release. Substituting opioids may decrease the incidence, and it may be alleviated by low-dose naloxone therapy. Muscle rigidity begins at or just after the loss of consciousness, and may manifest as hoarseness in mild cases. Severe episodes can make ventilation almost impossible, even after intubation. It can be minimised by co-administration of induction agents and benzodiazepines, and may be prevented by pretreatment with muscle relaxants. It is aggravated by the use of nitrous oxide. It is seen more commonly with the potent phenylpiperidines than with morphine, the risk is increased with increased opioid dose, and it can be reversed by administration of naloxone.
Opioids decrease thermoregulatory thresholds to a similar degree to the potent volatile agents. Pethidine is unique in its ability to reduce shivering, probably via a KOP-mediated action, and has been effectively utilised for hypothermic, postoperative, blood transfusion-related and epidural-related shivering. Tramadol has also been demonstrated to be efficacious in this regard.
All alkaloids of opium cause a histamine weal when injected intradermally. Histamine release and associated hypotension are variable in incidence and severity, and are reduced by slow IV administration and ameliorated by intravascular fluid loading. The phenylpiperidines (except pethidine) are devoid of this side effect. The histamine release may be localised or generalised, and may cause subjective facial flushing and variable itch.
Opioid-specific effects
A number of important opioid-related therapeutic and adverse effects are specific to the individual opioid being used (e.g. codeine – antitussive and constipation). Pethidine is a unique opioid because of its non-opioid effects. It has a local anaesthetic effect of equivalent potency to cocaine, which gives it advantages when administered neuraxially, and it has a quinidine-like effect on cardiac muscle to reduce cardiac irritability and arrhythmias. Pethidine overdose produces the unique combination of cardiovascular collapse, mydriasis, hyperreflexia and convulsions in addition to respiratory depression.
The phenylpiperidine family (except remifentanil) has been associated with postoperative respiratory depression after high-dose administration, due to secondary peaks in plasma levels. This has been attributed to a number of possible aetiologies, but most probably results from the release of opioid from body stores, with increases in peripheral perfusion and shivering postoperatively.
Opioid metabolites
Some of the significant adverse effects of opioids relate to the production of toxic metabolites. Morphine-3-glucuronide (M3G) may be responsible for the neuroexcitatory effects observed with large-dose chronic morphine administration. Moreover, it may be an opioid antagonist and possibly associated with hyperalgesia (paradoxical pain) seen after morphine administration. Norpethidine has half the analgesic potency of pethidine, but 2–3 times the convulsant activity. Because of the long half-life of norpethidine, significant accumulation occurs within 48 hours and steady state is achieved within 3–6 days. Greater amounts of norpethidine are produced with oral administration. Norpethidine accumulation initially manifests as subtle mood alteration with anxiety, culminating in potentially fatal reactions at higher concentrations. Norpropoxyphene also has a weak opioid action, but causes central excitatory effects and cardiac conduction disturbances.
Not all opioid metabolites are inactive or toxic. In fact some are necessary for clinical efficacy. Examples include morphine and morphine-6-glucuronide (M6G), codeine and morphine, tramadol and its M1 metabolite. The main metabolite of naltrexone, 6-β-naltrexone, is active with a longer half-life than its parent, and is necessary for the efficacy of naltrexone.
Pharmacokinetics
Figure 31.9 shows pharmacokinetic data for some commonly used opioids.
Drug | Protein binding (%) | pKa | Octanol/water partition coefficient | Vd (steady state) L kg–1 | t1/2β (min) | Cl (mL kg–1 min–1) | HER |
---|---|---|---|---|---|---|---|
Alfentanil | 92 | 6.5 | 129 | 0.8 | 100 | 6 | 0.4 |
Buprenorphine | 96 | 8.6 | 3.0 | 330 | 19 | 0.8 | |
Codeine | 10 | 8.2 | 0.6 | 5.4 | 180 | 11 | 0.4 |
Fentanyl | 85 | 8.4 | 813 | 4.0 | 100 | 13 | 0.9 |
Morphine | 35 | 7.9 | 1.4 | 3.5 | 180 | 15 | 0.8 |
Naloxone | 45 | 7.9 | 34 | 2.0 | 70 | 25 | 0.9 |
Remifentanil | 70 | 7.1 | 18 | 0.35 | 15 | 50 | N/A |
Tramadol | 20 | 9.4 | 1.4 | 4.0 | 360 | 10 | 0.3 |
HER, hepatic extraction ratio; Vd, volume of distribution; t1/2, half-life
Administration
The choice of route of administration depends on the opioid being utilised, pain severity, the need for drug titration and any drug or patient contraindications to a particular route. The mode of administration may influence the onset of peak analgesia and side effects. For example, respiratory depression may occur 7 minutes after an IV dose of morphine, but not until ~30 minutes after IM or 6–10 hours after intrathecal dosing.
Certain routes confer advantages for specific opioids. Intrathecal administration may provide a greater quality and potentially a longer duration of pain relief, with a lower incidence of supraspinal effects. However, a higher incidence of specific side effects (nausea, urinary retention, pruritis) occurs. Hydrophilic opioids (morphine) and the active metabolites of diamorphine remain in the CSF for longer, where they produce prolonged analgesia. At higher doses they ascend to produce analgesia at higher segmental levels. An unfortunate and hazardous consequence of this migration is a dose-dependent risk of late respiratory depression (peak 6–10 hours after dose). The more lipophilic opioids bind rapidly to local sites, and from there are absorbed intravascularly before they can migrate cephalad.
In respect of epidural opioid pharmacokinetics, dural penetration is related to opioid molecular size and lipophilicity, with sufentanil, fentanyl, and morphine peak CSF concentrations occurring at 6, 20, and 60–240 minutes respectively. Only 3–5% of a morphine dose crosses the dura. Opioid lipophilicity is also important for uptake into peridural fat and epidural veins. Hence, plasma opioid concentrations of lipophilic opioids given epidurally are often equivalent to those levels achieved after IM administration.
No opioid agonist demonstrates dose-dependent pharmacokinetics. First-pass metabolism of orally administered opioids occurs in the liver and the intestinal wall (up to 50%). Opioids given IM or SC have 100% bioavailability, but peak plasma concentrations can vary up to fivefold depending on body temperature, site of administration and haemodynamic status. IV administration results in a much narrower range of plasma concentrations.
The lung exerts a significant first-pass effect on several highly lipid-soluble opioids. Prior administration of other lipophilic amines such as propranolol reduces pulmonary uptake, by saturating binding sites.

Full access? Get Clinical Tree
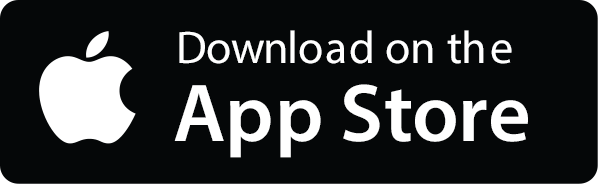
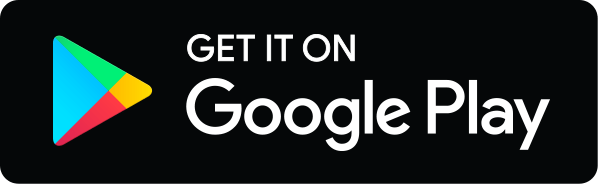