FIGURE 18.1 A historic paradigm was created to depict immune activation after injury or sepsis. It was thought that initial innate inflammatory response was followed by a compensatory anti-inflammatory adaptive immune response, which was proportional to the initial innate activation. Under physiologic circumstances, both the inflammatory and anti-inflammatory responses were self-contained (blue line). However, an excessive maladaptive inflammatory response would lead to self-injury, followed by increased susceptibility to infection from an equally maladaptive anti-inflammatory response (red line). This paradigm, dominant among investigators, led to the development of multiple clinical trials aimed at curtailing innate responses and/or enhancing adaptive immunity.
In contrast to innate immunity, the adaptive immune response is highly specific and is activated by exposure to infectious agents or molecules. The defining characteristics of adaptive immunity are exquisite specificity for distinct molecules and an ability to “memorize” and respond more vigorously to repeated exposure. Because of its specificity for a particular antigen, adaptive immunity is also referred to as antigen-specific immunity. Memory increases the rapidity, magnitude, and defensive capabilities with each successive exposure to a particular molecule.
Cellular Components of the Immune Response
Two cell families compose the cells of the immune response; myeloid cells and lymphocytes. Myeloid cells constitute the most abundant nucleated hematopoietic cell lineage in humans. Classic ontogenic studies describe common myeloid progenitors (CMPs) eventually differentiate into three distinct mature cell lines: macrophages (derived from monocytes), dendritic cells (DCs), and granulocytes. Each of these cell lines play classic roles during immune activation directly functioning as effectors—such as in bacterial killing—while modifying immune and nonimmune physiologic functions.
TABLE 18.1 Structural Division of Immune Responses |
![]() |

FIGURE 18.2 The possibility of studying the genomic response using new technologies has allowed a better understanding of the complexity of immune activation. A gargantuan effort performed by multiple centers studying the genomic response in different peripheral blood immune cells from both patients after injury and burns, as well as in normal volunteers receiving endotoxin. A virtual “genomic storm” was reported by Xiao and colleagues (2). Both innate inflammatory and adaptive anti-inflammatory responses occur simultaneously. Physiologic responses are self-contained and lead to resolution of illness (blue lines). On the other hand maladaptive responses lead to a persistent inflammatory and immunosuppressive syndrome that is also associated with severe muscle wasting and progression to malnutrition (red lines).
Recently, an important group of mostly immature myeloid cell subpopulations have been identified playing key roles in immune responses in healthy individuals and during illness. These immature myeloid cells suppress lymphocyte function through two metabolic pathways involving the metabolism of arginine. Due to their suppressive functions, these cells are now called myeloid-derived suppressor cells (MDSCs). There are at least two subpopulations of MDSC, but it is possible there are more. MDSCs accumulate during pregnancy and appear to play an important role in preventing T-cell activation against the growing fetus. MDSCs also accumulate in certain cancers, during infection, and after physical injury (surgery or trauma) where they can cause severe immune suppression, worsening overall prognosis (4).
The main cells of adaptive immunity are lymphocytes; the two main subpopulations of which are designated B and T lymphocytes, which refer to the organs in which those cells are found to mature, bursa of Fabricius in birds (equivalent to bone marrow in mammals) and thymus, respectively. Lymphocyte responses are aided and modified in their provision of physiologic immunity by other cells, including myeloid cells (DCs, macrophages, monocytes, and others). Substances and molecules that induce specific immune responses, or are the targets of such responses, are termed antigens. There are two types of adaptive response: humoral immunity mediated by antibodies and B lymphocytes, and cell-mediated immunity, which involves T lymphocytes. The cardinal features of adaptive immune responses, besides specificity and memory, are diversity, which is the ability to respond to a large variety of antigens; specialization, considered as the optimal response for a particular antigen; self-limitation, allowing a regulated and finite immune response also known as immune homeostasis; and self-tolerance, a nonreactivity to self.
Both innate and adaptive immune responses can be divided into distinct phases: recognition of antigen, activation, and the effector phase of antigen elimination, followed by the return to homeostasis; and in the case of adaptive response, the maintenance of memory.
Lymphoid tissues are classified as generative or primary lymphoid organs, and as peripheral or secondary lymphoid organs. Primary lymphoid organs are bone marrow, where all lymphocytes arise and also where B lymphocytes mature, and the thymus, where T lymphocytes mature and reach a stage of functional competence. The peripheral lymphoid organs and tissues include lymph nodes, spleen, and the cutaneous and mucosal immune system. Specialized microenvironments within primary immune organs support immune cell growth and maturation, while secondary lymphoid organs are sites in which optimal adaptive immune responses are initiated and developed. Lymph nodes are sites of immune response to lymph-borne antigens, and the spleen is the major site of immune response to blood-borne antigens. Similarly, cutaneous and mucosal immune systems are specialized for the best response to potential antigens coming through skin and mucosal surfaces, respectively. Although some cells are permanently resident in one tissue, lymphocytes continuously “traffic” through the bloodstream and lymphatic system, from one peripheral (secondary) lymphoid tissue to another. Lymphocyte recirculation and migration to particular tissues are tightly regulated and mediated by adhesion molecules, chemokines, and their receptors, and depend on the cell maturation and activation stage.
The main cells of the immune system involved in the adaptive immune response are: antigen-specific lymphocytes, specialized antigen-presenting cells (APCs) that display antigens activate lymphocytes, and effector cells that function to eliminate antigens (microbes). Lymphocytes are exclusively capable of specifically recognizing and distinguishing different antigens; no other cell types are able to do so in our body. Lymphocytes consist of subsets that are different in their function, but are morphologically indistinguishable.
B lymphocytes are the only cells capable of producing antibodies. They recognize extracellular (soluble or cell surface) antigens and differentiate into antibody-secreting cells, thus functioning as the mediators of humoral immunity. T lymphocytes, the mediators of cellular immunity, consist of functionally distinct subpopulations. A growing complexity in the number of T-lymphocyte subpopulations is now recognized playing key distinct roles during health and disease. Attempts at understanding the role of T-lymphocyte subpopulations include identification of a functional state (naïve, memory, effector cells) and phenotypic differentiation based on the presence of specific receptors. Yet other mechanisms of differentiation of T-cell subsets are that of the production of specific cytokines and telomere length. There is a significant amount of controversy in the attempts to determine the biologic roles of T-lymphocyte subsets during health and disease. Furthermore, this area of knowledge is changing rapidly as new receptors, cytokines, and functional roles are identified. To complicate things further there are significant interspecies differences including different nomenclatures.
It has emerged that there is a significant overlap between the different T-cell subpopulations suggesting a continuum of differentiation. Nevertheless, it is important to understand the historically established subpopulations, how they are identified, and the biologic roles that these can play under different circumstances. The classic classification of T cells, divides them into helper T cells, cytolytic or cytotoxic T cells (CTLs), and regulatory T cells. NK cells are a third population of lymphocytes with receptors different from those of B and T cells and with major function involving innate immunity.
The use of monoclonal antibodies has allowed the identification of unique surface proteins, which are present only in a particular cell population. These surface proteins have thus been used as their characteristic identification marker(s). The standard nomenclature for these proteins is the CD (cluster of differentiation) numerical designation that currently consists of over 350 different molecules. The majority of characterized molecules, however, are present on more than one cell population, where their presence defines maturation stage or particular effector function. The classification of lymphocytes by CD antigen expression is now widely used in clinical medicine and experimental immunology. T lymphocytes express the CD3 receptor on the cell membranes. According to the CD classification, helper T cells are defined as CD3+ and CD4+; most CTLs are CD3+ and CD8+, while regulatory T cells are a subgroup of helper cells with an additional low expression of the CD25 activation marker—the α-chain of the surface receptor for interleukin-2 (IL-2Rα)—and are defined as CD3+, CD4+, and CD25+. B cells are characterized with the expression of CD19, while NK cells express the CD56 molecule (1).
APCs are a cell population that are specialized to capture microbial and other antigens, process and present the antigens to lymphocytes, and provide signals that stimulate the proliferation and differentiation of lymphocytes. The major type of APC is the DC, which is found under the epithelia and in most organs, where it is poised to capture antigens and transport them to peripheral lymphoid organs. There are two major subtypes of DCs: myeloid and plasmacytoid. DCs are the most potent APCs capable of stimulating “naïve” T cells as they encounter antigens for the first time. Mature mononuclear phagocytes, tissue macrophages, also function as APCs in a T cell–mediated, adaptive immune response. Macrophages that have ingested microbes may activate “naïve” T cells while, in turn, effector T cells may stimulate the macrophages to more efficiently kill ingested pathogens. Follicular dendritic cells (FDCs) are cells present in the lymphoid tissue that trap antigens in the complex with antibodies or complement products and display those antigens for recognition by B lymphocytes.
After being stimulated by APCs, lymphocytes differentiate into effector cells. Differentiated effector helper T cells secrete cytokines (see below: Cytokines) and interact with and activate macrophages and B lymphocytes. Effector CTLs develop granules containing proteins that kill virus-infected and transformed host (tumor) cells; B cells differentiate into plasma cells that actively synthesize and secrete antibodies. Some antigen-stimulated B and T lymphocytes differentiate into memory cells whose function is to mediate rapid and enhanced responses to second and subsequent exposures to antigens.
Cytokines
These are proteins secreted by the cells of innate and adaptive immunity that mediate many of the functions of those cells. Cytokines are produced in response to microbes and other antigens, and different cytokines stimulate diverse responses of cells involved in immunity and inflammation. In the activation phase of the adaptive immune response, cytokines stimulate growth and differentiation of lymphocytes; in the effector phase, they modulate different cells for a variety of functions including proliferation, cytotoxicity or, alternatively, downregulation.
The number of cytokines, like T-lymphocyte subpopulations, has grown significantly. In some cases, the production of a specific cytokine is characteristic of particular T-lymphocyte subsets. Thus, for example, the production of interferon gamma (IFN-γ) is characteristically generated by NK and TH1 (helper T1) T cells upon activation. In contrast, the production of IL-4 is produced by TH2 (helper T2) cells. Cytokines exhibit multiple potent biologic activities including mediating innate immunity (IL-1, IL-6, and TNF-α); cytokines that control (either stimulate or modulate) adaptive immunity (IL-2, IL-4, IL-5, and IFN-γ); and those that stimulate hematopoiesis (IL-3, IL-7, and some growth factors) among others. Although different cells produce cytokines of innate and adaptive immunity, and those cytokines act on different target cells, this distinction is not absolute because cytokines produced during such reactions often have overlapping actions (1).
Antigen Recognition and Processing
Antigen recognition is the first phase of the adaptive immune response. Antibodies, major histocompatibility complex (MHC), and T-cell antigen receptors (TCRs) are the three classes of molecules used in adaptive immunity to recognize antigens. Antibodies produced in a membrane-attached form function as B-cell receptors for antigen recognition. The interaction of antigen with membrane antibodies initiates B-cell activation and, thus, constitutes the recognition phase of the humoral immune response. B-lymphocyte differentiation, upon activation, proceeds along two pathways: one that requires stimulation by helper T lymphocytes, the T cell–dependent pathway, or a second T cell–independent pathway. The antigens recognized by B cells may be in their native, nondegraded form and not require prior processing of the antigen by other immune system cells. In order to get help from T cells, however, B cells need to internalize the membrane antibody–antigen complex, degrade protein, and display it back on the cell surface membrane in complex with the class II MHC molecule. As explained below, T cells can recognize antigens only if they are processed and presented on the membrane surface of APCs in complex with the MHC molecules. Antibodies are also produced in a secreted form by activated B cells. In the effector phase of the humoral immune response, secreted antibody binds to antigens and triggers several effector mechanisms that eliminate the antigens. Although of the same antigen specificity, membrane-bound antibodies are involved in antigen recognition and B-cell activation, while secreted antibodies are responsible for triggering the effector phase of the humoral immune response and antigen clearance.
In contrast to B cells and their secreted antibodies that can recognize soluble as well as cell-associated antigens in their native form, T cells can only recognize antigens that are displayed on other cell surfaces and are degraded into fragments by the body’s various APCs. The task of displaying (presenting) cell-associated antigens for recognition by T cells is performed by specialized proteins that are encoded by genes in a locus called the major histocompatibility complex. MHC molecules are integral components of the ligands that most T cells recognize, because the antigen receptors of T cells are actually specific for the complex of (foreign) peptide antigens and (self) MHC molecules.
MHC molecules are found on immune and nonimmune cells. There are two main types of MHC gene products: class I and class II MHC molecules, which sample different pools of protein antigens, cytosolic or intracellular antigens, and extracellular antigens that have been endocytosed, respectively. MHC class I molecules are present on virtually all nucleated cells where they display antigens to be recognized by CD8+ cytotoxic T lymphocytes. MHC class II molecules are found primarily on APCs and primarily activate CD4+ helper T cells. Once an antigen is engulfed by an APC, it is degraded to its peptide fragments. These antigen fragments are then integrated with the MHC molecule and transported to the cell membrane, where they are exposed to neighboring cells within a complex that includes either class I or class II MHC molecules. T lymphocytes subsequently recognize the MHC–antigen (MHC–Ag) complex and initiate antigenic response (1).
All T lymphocytes recognize an antigen by specific T-cell receptor (TCR) molecules expressed on their cell membrane. These TCR molecules function similarly to a lock and key with the MHC–Ag complex. Only a few T lymphocytes constituting one T-cell clone are specific for one particular antigen. In addition to T-cell receptor binding to the MHC–Ag complex, multiple membrane receptors are used in APC–T-cell interaction. Specificity of T-lymphocyte clones plays important physiologic functions. Specificity assures a graded T-cell response to infection, where upon activation, clonal expansion is sufficient to successfully eliminate the offending organism, cell, or foreign body. Typically, only one in a million T cells responds to a given antigenic stimulus.
Clonal expansion can, in fact, fail in some instances being now recognized as a major pathophysiologic mechanism in different illnesses. Toxic shock syndrome (TSS) is a severe and often fatal disease that may occur in response to an infection by Staphylococcus aureus, although it can also occur as a response to other organisms. TSS was famously described with the use of certain types of tampons (now off the market). In contrast to appropriately processed antigens that stimulate a limited number of T cells (one clone) bearing the same TCR (approximately one in a million circulating T cells), some bacterial proteins and toxins are able to stimulate T cells without first undergoing endocytosis and degradation. Those molecules, characterized as superantigens, can simultaneously stimulate T cells with different antigen specificity, and subsequently induce polyclonal activation with the extensive systemic release of cytokines. The stimulatory effect of superantigens is a consequence of direct binding to the class II MHC on APCs and the non–antigen-specific part of TCR on T cells, thus being able to activate 2% to 20% of all T cells. The massive T-cell activation results in the release of large amounts of inflammatory cytokines that induce T-cell anergy or death (apoptosis), which severely disturbs the ability of the immune system to respond appropriately to infection. As a consequence of the systemic effects of released cytokines, infected patients develop systemic effects including fever, endothelial damage, profound hypotension, disseminated intravascular coagulation, multiple organ failure, and death.
Clonal deletion and clonal inactivation are essential processes that eliminate some T-cell clones or prevent them from reacting to an antigenic stimulus. Some clones of T lymphocytes recognize self-antigens in local cells and tissues, and these need to be eliminated to avoid self-injury. Clonal deletion is a process that occurs under physiologic circumstances and is responsible for self-tolerance. However, clonal elimination may fail and is, indeed, what happens in some autoimmune diseases such as rheumatoid arthritis and possibly in inflammatory bowel disease (IBD) (1).
Immune Effector Functions
Antigen Clearance and Initial Immune Activation
Once the immune system recognizes an antigen, inflammation and clearance processes are initiated. Activation and the effector phase of the adaptive immune response are intended to eliminate antigen in the most appropriate and efficient way. For example, one set of components of the immune system is activated in response to the extracellular antigen (antibodies and helper T cells), while others are more effective in the elimination of the intracellular antigen (CTLs and NK cells). Regardless of the type of antigen, processes involved in the activation and effector phase of immune response induce changes in the surrounding tissue, defined as inflammation. The antigen clearance process is enhanced within inflamed tissues by increased vascular flow, altered vascular permeability, and the recruitment of immune cells. Those changes also produce four cardinal clinical signs of inflammation or ongoing immune response: warmness (calor), redness (rubor), swelling (tumor), and pain (dolor), often accompanied by malfunction of the involved organ (functio laesa).
Several physiologic mechanisms are involved in circulating inflammatory cell adhesion to vascular endothelium and subsequent traffic of immune cells from the circulation into tissues (diapedesis). The process of adhesion is governed by adhesion molecules such as L-selectin, LFA-1, and MAC-1 in immune cells and in endothelial cells (P-selectin, E-selectin, ICAM-1, and ICAM-2 molecules) after stimulation. Expression and function of these molecules is modulated by “early response” cytokines (TNF-α and IL-1) secreted by activated tissue macrophages and other APCs. Additionally, IL-8 production by endothelial cells and tissue fibroblasts is a major component of the chemotactic gradient facilitating neutrophil migration across the endothelial surface. Neutrophils are capable of direct recognition and phagocytosis of circulating antigens. After neutrophil phagocytosis, enzyme-laden lysosomes fuse with the antigen-containing phagosome, digesting and destroying the antigen. Neutrophils possess receptors for the Fc portion of immunoglobulins as well as receptors for complement components. Thus, opsonization or coating of antigens by immunoglobulins and complement markedly enhances phagocytic capability and antigen elimination (5).
The predominant mechanism for adequate reaction to, and rapid clearance of, extracellular antigen involves antibodies or immunoglobulins. Antibodies possess unique antigen specificity, thereby narrowing the inflammatory response to the specific antigenic target. Antibodies circulating in the bloodstream or interstitial fluid promptly recognize, and bind to, an antigen; but because antibodies do not directly perform any effector function, the elimination of antigen requires interaction of antibody with the components of innate immunity such as complement proteins or phagocytes and eosinophils. Antibody-mediated effector functions include neutralization of microbes or toxic microbial products, activation of the complement system, opsonization (coating) of antigens for enhanced phagocytosis, antibody-dependent cell-mediated cytotoxicity (ADCC), and immediate hypersensitivity in which antibodies trigger mast cell activation.
Antibodies
Antibodies are highly specific to a particular antigen. The molecular site at which the antibody recognizes an antigen is called an epitope. Specificity and effector functions of antibodies depend on their basic structure. An antibody molecule has a symmetric core structure composed of two identical light chains and two identical heavy chains. Both heavy chains and light chains consist of amino terminal variable (V) regions and carboxyl terminal constant (C) regions. While light- and heavy-chain amino terminal variable regions together participate in antigen recognition, only the constant regions of the heavy chains are involved in antibody effector functions.
Several different types of antibodies or immunoglobulins (Ig) exist and can be divided into distinct classes and subclasses on the basis of differences in the structure (heavy chain), tissue and biologic fluid distribution, functional capability, and timing of the immune response. In order from the highest to the lowest serum concentration, those classes of antibody molecules (also referred to as isotypes) are designated as IgG, IgA, IgM, IgD, and IgE.
Different classes of antibodies perform different effector functions. Among the most notable functions of immunoglobulins are opsonization and the capacity to activate complement. IgG, IgM, and IgA are also crucial for phagocytic cells to recognize an antigen (known as opsonization). Opsonization by IgG and IgM expedites the clearance of circulating antigens, whereas the secretion of IgA onto mucosal surfaces facilitates the clearance of invading organisms by mucosal surface macrophages and neutrophils. Because of its larger size, the function of IgM is confined primarily to the intravascular clearance of antigens, whereas IgG readily diffuses into the extravascular space. After being coupled with an antigen, antigen–antibody complexes—also termed immune complexes—are normally cleared by phagocytic and red blood cells. The clearance of immune complexes from the circulation is dependent on effective opsonization, binding of the immune complex–bound C3b fragment to CR1 on erythrocytes, and subsequent transport to the liver and spleen. IgD is primarily found on the surface of naïve B cells where it functions as the receptor for antigen recognition; a small amount of IgD is also secreted, generally along with IgM. The physiologic function for IgD appears that of activation of basophils. In contrast to other immunoglobulin classes, once secreted, IgE is present free in the serum for a very short time, since it binds rapidly to the specific receptor on basophils, eosinophils, and mast cells. Antigen activation of cell-bound IgE results in the immediate release of various mediators, including histamine, serotonin, and leukotrienes. Although IgE is commonly connected to the allergic reactions, the physiologic function of IgE seems to be an immediate response to antigen and the induction of vascular dilation, increased vascular permeability, and the recruitment of immune cells; another important immune function of IgE is to protect the host against parasites.
The complement system is capable of generating a broad series of inflammatory actions associated with antigen clearance. These actions include lysis of cells bearing antigen–antibody complexes, opsonization of antigens, chemotaxis of inflammatory cells, and generation of anaphylactic reactions. Complement activation may be accomplished by either the classic pathway, initiated by antigen–antibody complexes, or the alternate route initiated by antigenic protein aggregates, endotoxin, or insoluble compounds with certain surface characteristics. With sequential proteolysis of complement substrates, various complement fragments with neutrophil and eosinophil chemotactic properties, as well as vasodilatory effects, are generated that produce the previously mentioned cardinal signs of inflammation (6).
Cellular Involvement in Immune Response
The major cells involved in antigen clearance include APCs and lymphocytes, neutrophils, and various organ-specific structural cells or tissue macrophages. Although many antigens may be destroyed within mononuclear phagocytic cells by intracellular enzymes, some antigens may become sheltered within these cells. Elimination of these antigens requires additional activation from helper T cells, which predominates in the case of bacterial infection. In general, the helper population of T lymphocytes (CD4+) supports the function of mononuclear phagocytic cells and enhances antibody production by B lymphocytes, thus supporting the clearance of extracellular antigens. Activated T cells increase the secretion of cytokines that are crucial for regulation of the immune response.
On the basis of the pattern of cytokines secreted, CD4+ lymphocytes are subdivided into two major classes: Th1 or Th2. The cytokines secreted by the Th1 group of CD4+ cells, including IL-2 and IFN-γ, are potent stimulants of the cell-mediated immune response. Th2 CD4+ cells secrete cytokines such as IL-4 and IL-10 and IL-13 that stimulate humoral responses including the secretion of antibodies while exerting a regulatory role on cellular immune responses.
It is apparent that Th1 and Th2 responses are carefully orchestrated for successfully overcoming illness as could be seen in critically ill patients. A Th1 response is essential for the survival against infection, while a Th2 response prevents an excessive inflammatory response and sets the stage for healing of tissues. While understanding helper T cell responses as a dichotomous response is a significant oversimplification of immune response, it has served to understand how an imbalance between Th1 and Th2 can be a cause of illness. Thus, caution has to be exercised while interpreting the role of Th1 and Th2 cellular responses in complex processes such as seen in critically ill patients.
Increasing attention is being paid to the presence of different Th1 and Th2 subpopulations during health and disease. Predominance (also known as polarization) of Th1 responses appear to be a causative factor in continued unabated inflammation and self-injury in patients with Crohn disease; on the other hand a predominance of Th2 responses and the subsequent regulation of Th1 functions may worsen outcomes in patients with certain cancers, HIV, and tuberculosis (7).
The CD8+ population of T lymphocytes (CTLs) is essential for combating infection generating, for example, lysis of the infected cell such as is observed during viral infections. In a carefully orchestrated process, after exposure to processed antigen, and under the influence of the lymphokines IL-2 and IFN-γ, activated CD8+ cells proliferate, synthesize, and secrete membrane attack molecules, which result in lysis of the antigen-bearing cell. Besides the important role of the T lymphocyte in clearing microbial pathogens, CTLs are crucial for recognizing and eliminating self-transformed tumor cells. Tumor cells may express new antigens, which are presented on the cell surface within class I MHC molecules antigenic complexes. When functioning adequately, recognition of tumor cells arising in tissues is considered to be an essential and, in most cases, successful mechanism that of tumor surveillance and killing.
Similar to the cell lysis by CTLs, NK cells lyse neighboring cells by secreting membrane attack molecules (perforin and granzymes). Unlike the CTL response, the NK cell lysis of antigen-bearing cells does not seem to be antigen specific. Killer lymphocytes, the third major cytolytic cell population, are coated with surface receptors for antibodies. Killer lymphocytes may localize to antigen–antibody-coated cells, where they release their cytotoxic granules. Antibody recognition is crucial to this system, and killer lymphocyte function seems to be a component of antibody-dependent cytotoxicity.
NK cells and killer lymphocytes can be activated and made to proliferate in vitro under the influence of cytokines. These lymphokine-activated killer (LAK) cells may be reinfused into the body and have been investigated as cancer immunotherapy (8).
In addition to the primary immune APCs or professional APCs, structural cells, such as those of the endothelium, epithelium, and connective tissue, are also important to an effective immune response. Not only are these cells capable of secreting cytokines and inflammatory mediators, but after stimulation, they also express class II MHC molecules and may function in antigen presentation to T lymphocytes. Those cells, termed nonprofessional APCs, can play important physiologic roles. However, the activation of nonprofessional APCs by particular cytokines (IFN-γ) may underlie the organ dysfunction associated with chronic immune stimulation and inflammation (1).
Additional Factors and Mediators
Multiple additional inflammatory mediators from migrating leukocytes, such as proteases, oxygen radicals, leukotrienes, platelet-activating factors are released early on upon infection or after tissue injury, expanding the local inflammatory process. Conversely, several cytokines and soluble cytokine receptors are normally present to downregulate or limit the inflammatory response. Among these “anti-inflammatory” factors are IL-4, IL-10, IL-13, transforming growth factor (TGF)-β, other growth factors, and IL-1 receptor antagonist.
Myeloid-Derived Suppressor Cells and Arginine Metabolism
Increased numbers of myeloid cells are observed accumulating in the tumor and in lymphoid organs in patients with certain cancers. Similarly, myeloid cells accumulate rapidly in the spleen and in the circulation within hours of an injury or after surgery (9,10). These cells generally exhibit markers of immature myeloid cells and, given the appropriate growth stimuli, appear to exhibit the potential to differentiate in macrophages, granulocytes, or APCs. In cancer and after injury, immature myeloid cells are capable of suppressing T-lymphocyte function through two different enzymatic pathways that utilize arginine as a substrate; hence the name myeloid-derived suppressor cells (MDSCs). Nitric oxide synthase (iNOS) expression can be induced in myeloid cells through classic inflammatory stimuli including IL-1, TNF, and IFN-γ. Nitric oxide (NO) generated by iNOS can profoundly inhibit T-lymphocyte function. Conversely, anti-inflammatory stimuli such as IL-4, IL-13, and prostaglandin E2 (PGE2) induce arginase 1, which efficiently destroys arginine, an essential amino acid during T-lymphocyte activation. Arginine depletion inhibits T-lymphocyte function by acting on protein translation targeting the mammalian target of rapamycin (mTOR) and the general control nondepressible 2 (GCN2) serine/threonine-protein kinase (11).
Phenotypical and functional characteristics of arginine depletion have been described in T lymphocytes. Zhu et al. (4) first described these changes in cell cultures of T cells grown with limited arginine availability, demonstrating a decrease in the number of T-cell receptors on the cell membrane, an incapacity to proliferate, and a modest capacity to generate/utilize IL-2. It was later observed that expression of the ζ-chain, a peptide dimer whose concentrations significantly decrease in certain cancers and after trauma was exquisitely sensitive to arginine deprivation.
Immune suppression caused by MDSC through arginine depletion can be profound. Under physiologic circumstances, by accumulating in the placenta, arginine depletion may prevent fetal rejection. Under pathologic circumstances, MDSC may be part of an elaborate mechanism of tumor evasion, significantly worsening the prognosis of these patients. Loss of T-cell function (also known as anergy) is characteristically observed after trauma or after major surgery, and is associated with increased susceptibility to opportunistic infections such as Candida albicans and other fungal species (12).
Metabolic Effects of Immune Responses
Cytokines released into the systemic circulation as a consequence of either localized or systemic inflammation have been directly implicated in the pathophysiologic mechanisms of the organ dysfunction associated with major trauma, sepsis, and burns. If high plasma concentrations are achieved, IL-1, TNF-α, and IL-6 have been shown to have profound effects on body metabolism and are capable of inducing hypotension, fever, and cachexia. Their functions have been implicated in the manifestations of septic shock, and their concentration correlates with mortality. In response to TNF-α, NO is produced by endothelial cells and, along with the other mediators, promotes smooth muscle relaxation and vasodilation.
HYPERSENSITIVITY REACTIONS INCLUDING ALLERGIES
Adaptive immunity serves the important function of host defense against microbial infections. However, the immune system is also capable of causing tissue injury and disease. Hypersensitivity reactions are a group of illnesses caused by immune responses leading to tissue injury and even death. Hypersensitivity is a term that arose from the clinical observations that an individual who has been exposed to an antigen instead of developing tolerance created a detectable clinical reaction becoming “sensitized” to subsequent encounters with the antigen. A common cause of hypersensitivity diseases is failure of self-tolerance, which, under physiologic conditions, ensures that the individual’s immune system does not respond to his or her own antigens. Hypersensitivity diseases also result from uncontrolled or excessive responses against foreign antigens such as microbes and noninfectious environmental antigens (13).
Hypersensitivity diseases represent a clinically heterogeneous group of disorders that have been classified into four different types based on the nature of the abnormal immune response, the tissue injury caused, and the clinical manifestations that arise from these (Table 18.2).
Type I Hypersensitivity
Type I hypersensitivity reaction, also called immediate hypersensitivity, is the most prevalent type of hypersensitivity diseases and is characterized clinically as an allergic reaction. Type 1 hypersensitivity occurs when the patient has become “sensitized” to an otherwise innocuous antigen (also known as allergen) to generate IgE antibodies, which are bound to mast cells, basophils, and eosinophils. Upon activation these cells degranulate, releasing a variety of mediators including histamine, leukotrienes, prostaglandins, and other substances. These mediators collectively cause increased vascular permeability, vasodilation, bronchial and visceral smooth muscle contraction, and local inflammation.
TABLE 18.2 Types of Hypersensitivity Responses |
![]() |

Full access? Get Clinical Tree
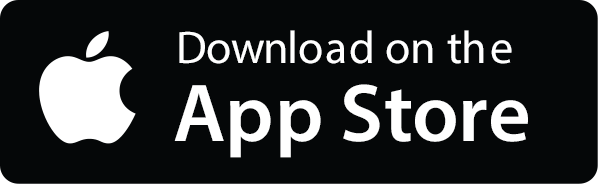
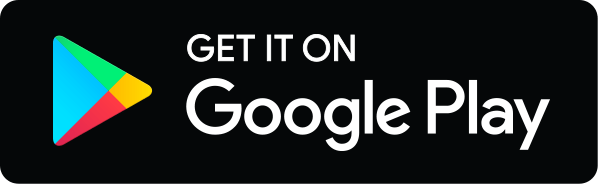