Airway Pressure Release Ventilation: Introduction
Controlled mechanical ventilation (CMV) is traditionally provided via an artificial airway to completely unload a patient’s work of breathing and assure adequate gas exchange during the acute phase of respiratory insufficiency, until the underlying respiratory function has resolved.1 The criteria used to determine when to terminate mechanical ventilation are essentially based on the clinical, and often, subjective assessment of the intensive care physician or on standardized weaning methods.2,3 The actual process of weaning the patient from CMV is carried out by allowing spontaneous breathing attempts with a T piece or continuous positive airway pressure (CPAP) or by gradually reducing mechanical assistance.4,5 Not surprisingly, gradual reduction of partial ventilator support benefits only patients who have difficulty in sustaining unassisted breathing.4 Although introduced as weaning techniques, partial support modes have become standard methods of providing primary mechanical ventilatory support in critically ill patients.
Basic Principles of Airway Pressure Release Ventilation
Airway pressure release ventilation (APRV)6 ventilates by time-cycled switching between two pressure levels in a high-flow (or demand-valve) CPAP circuit, permitting unrestricted spontaneous breathing in any phase of the mechanical ventilator cycle (Fig. 11-1). The degree of ventilator support with APRV is determined by the duration of the two CPAP levels and the mechanically delivered tidal volume (VT).6,7 VT depends mainly on respiratory compliance and the difference between the CPAP levels. By design, changes in ventilatory demand do not alter the level of mechanical support during APRV. When spontaneous breathing is absent, APRV is not different from conventional pressure-controlled, time-cycled mechanical ventilation.6,7
Figure 11-1
Airway pressure release ventilation ventilates by time-cycled switching between a high and low continuous positive airway pressure (CPAP) level in the circuit. Consequently, unrestricted spontaneous breathing is permitted in any phase of the mechanical ventilator cycle. Change between the two CPAP levels results in a change in functional residual capacity (ΔFRC), which equals the mechanical delivered tidal volume (VT). VT depends mainly on respiratory compliance and resistance and the airway pressure difference (ΔPaw) between the CPAP levels. Setting the time for the low (Tlow) and the high (Thigh) CPAP enables the adjustment of ventilator rate.
Synonyms used for APRV are biphasic positive airway pressure7 (BIPAP) and bilevel airway pressure (Bilevel). Biphasic positive airway pressure is identical to APRV except that no restriction is imposed on the duration of the low-CPAP level (release pressure).6,7 Based on the initial description, APRV keeps the duration of the low-CPAP level (release time) at 1.5 seconds or less.
Physiologic Effects
Radiologic studies demonstrate that ventilation is distributed differently during pure spontaneous breathing and CMV.8 During spontaneous breathing, the posterior muscular sections of the diaphragm move more than the anterior tendon plate.8 Consequently, when patients are supine, the dependent lung regions tend to be better ventilated during spontaneous breathing (Fig. 11-2). If the diaphragm is relaxed, it will be moved by the weight of the abdominal cavity and intraabdominal pressure towards the cranium; mechanical VT will be distributed more to the anterior, nondependent, and less perfused lung regions.9 When compared with spontaneous breathing, the latter leads, both in patients with healthy lungs and patients with diseased lungs, to lung areas in the dorsal lung regions close to the diaphragm, being less ventilated (or atelectatic). Recent results demonstrate that the posterior muscular sections of the diaphragm move more than the anterior tendon plate when large breaths or sighs are present during spontaneous breathing.10
Figure 11-2
During spontaneous breathing, the posterior muscular sections of the diaphragm move more than the anterior tendon plate. Consequently, in the supine position, spontaneous ventilation is preferably directed to well-perfused, dependent lung regions. Conversely, a mechanically delivered tidal volume is directed primarily to nondependent lung areas, away from regions with maximal blood flow. Thus, spontaneous breathing contributes to better ventilation–perfusion () matching.
Computed tomography (CT) of patients with acute respiratory distress syndrome (ARDS) reveals radiographic densities corresponding to alveolar collapse localized primarily in the dependent lung regions, which correlates with intrapulmonary shunting and accounts entirely for the observed arterial hypoxemia.11 Formation of radiographic densities is attributed to alveolar collapse caused by superimposed pressure on the lung and a cephalad shift of the diaphragm, most evident in dependent lung areas during CMV.12 Persisting spontaneous breathing is considered to improve the distribution of ventilation to dependent lung areas and thereby improve ventilation–perfusion () matching, presumably by diaphragmatic contraction that opposes alveolar compression.13 This concept is supported by CT observations in anesthetized patients demonstrating that contractions of the diaphragm induced by phrenic nerve stimulation favor distribution of ventilation to dependent, well-perfused lung areas, decreasing atelectasis formation.14
Spontaneous breathing with APRV in experimentally induced lung injury is associated with less atelectasis formation on end-expiratory spiral CT of the whole lungs and on CT scans above the diaphragm (Fig. 11-3).15–17 Although other inspiratory muscles may contribute to improvement in aeration during spontaneous breathing, the craniocaudal gradient in aeration, aeration differences, and the marked differences in aeration in regions close to the diaphragm between APRV with and without spontaneous breathing suggest that diaphragmatic contractions play a dominant role on the observed aeration differences and improvement of end-expiratory lung volume.15–17 Experimental data suggest that recruitment of dependent lung areas may be caused essentially by an increase in transpulmonary pressure (Ptp) secondary to the decrease of pleural pressure (Ppl) with spontaneous breathing during APRV.18
Because the posterior muscular sections of the diaphragm contract more than the anterior tendon plate, a decrease in Ppl and the concomitant localized increase in Ptp should explain entirely the successful recruitment of atelectatic areas in the dependent lung regions adjacent to the diaphragm.8,10,14–17 In the apical lung regions, differences in tidal volume distribution between the dependent and nondependent lung is not significantly different with spontaneous breathing.15,16 Inhomogeneous distribution of tidal ventilation during CMV in patients with acute lung injury (ALI)19,20 may be explained by regional differences in Ptp. A cephalocaudal decrease in Ptp may be partially explained by the transmission of abdominal pressure to the thoracic cavity,12 decreasing from base to apex. In addition, compression of lung tissue by the weight of the lungs and the heart may differ regionally.12,19,20 Although increase in Ptp caused by spontaneous breathing is maximal in the dependent lung areas adjacent to the diaphragm,8,10,14–17 it is unlikely that the resulting absolute Ptp in the dependent lung regions is higher than in cephalad lungs areas in the absence of spontaneous breathing. This concept is supported by experimental findings that gas volume and aeration are not higher in dependent lung regions with spontaneous breathing than in cephalad lung regions in the absence of spontaneous breathing.17 Furthermore, CT observations in experimentally induced lung injury demonstrate that, during spontaneous breathing with APRV a large portion of the VT is distributed to the initially collapsed dependent lung regions, resulting in less cyclical alveolar collapse.16
In patients with ARDS, APRV with spontaneous breathing of 10% to 30% of the total minute ventilation (VE) accounts for an improvement in matching, intrapulmonary shunting, and arterial oxygenation.13 These results confirm earlier investigations in animals with induced lung injury,21–23 which demonstrated improvement in intrapulmonary shunt and arterial oxygenation during spontaneous breathing with APRV. An increase in arterial oxygenation in conjunction with greater pulmonary compliance indicates recruitment of previously nonventilated lung areas. Clinical studies in patients with ARDS show that spontaneous breathing during APRV does not necessarily lead to instant improvement in gas exchange. Instead, improvement in oxygenation continues over the 24 hours after the start of spontaneous breathing.24
In patients at risk of developing ARDS, maintaining spontaneous breathing with APRV resulted in lower venous admixture and better arterial oxygenation over a period of more than 10 days as compared to CMV with subsequent weaning.25 These findings are supported by randomized controlled trials and case-matched analysis.26–28
Application of a ventilator breath generates an increase in airway pressure and, therefore, in intrathoracic pressure, which, in turn, reduces venous return to the heart. In normovolemic and hypovolemic patients, this action produces a reduction in right-ventricular and left-ventricular filling and results in decreased stroke volume, cardiac output, and oxygen delivery.29 To normalize systemic blood flow during mechanical ventilation, intravascular volume often needs to be increased and/or the cardiovascular system needs pharmacologic support. Reducing mechanical ventilation to a level that provides adequate support for existing spontaneous breathing should help reduce the cardiovascular side effects of ventilator support.30
Periodic reduction of intrathoracic pressure, achieved by maintaining spontaneous breathing during ventilator support, promotes venous return to the heart and right-ventricular and left-ventricular filling, thereby increasing cardiac output and oxygen delivery.31 Experimental21–23 and clinical13,25 studies show that when spontaneous breathing during APRV achieves 10% to 40% of total VE, with no change in VE or airway pressure limits, the cardiac index increases. A simultaneous rise in right-ventricular end-diastolic volume during spontaneous breathing with APRV indicates improved venous return to the heart.13 In addition, outflow from the right ventricle, which depends mainly on lung volume (the major determinant of pulmonary vascular resistance), may benefit from decrease in intrathoracic pressure during APRV.13
Patients with left-ventricular dysfunction may not benefit from either the augmentation of venous return to the heart or the increase in left-ventricular afterload that occurs with the lowering of intrathoracic pressure. Thus, switching abruptly from CMV to pressure-support ventilation (PSV) with a simultaneous reduction in airway pressure can cause further decompensation in patients with existing cardiac insufficiency.32 Räsänen et al33 showed a need for adequate ventilatory support and CPAP levels in patients with respiratory and cardiogenic failure. Provided that spontaneous breathing receives adequate support and that satisfactory CPAP levels are applied, maintaining spontaneous breathing during APRV should not be a disadvantage and is not per se contraindicated in patients with ventricular dysfunction.33
An increase in cardiac index and arterial oxygenation (PaO2) during APRV improves the relationship between tissue oxygen supply and demand because oxygen consumption remains unchanged despite the work of spontaneous breathing. In accordance with previous experimental21,22 and clinical findings,13 total oxygen consumption is not measurably altered by adequately supported spontaneous breathing in patients with low lung compliance. An increase in oxygen delivery with unchanged oxygen consumption indicates an improved tissue oxygen supply and demand balance, as reflected by a decrease in oxygen extraction ratio and higher mixed venous partial pressure of oxygen.
By reducing cardiac index and venous return to the heart, CMV can have a negative effect on the perfusion and functioning of extrathoracic organ systems. An increase in venous return and cardiac index, secondary to the periodic fall in intrathoracic pressure during spontaneous inspiration, should significantly improve organ perfusion and function during partial ventilator support. In patients with ARDS during spontaneous breathing with APRV, renal perfusion and glomerular filtration rate improve.34 Using the colored microsphere technique, Hering et al35

Full access? Get Clinical Tree
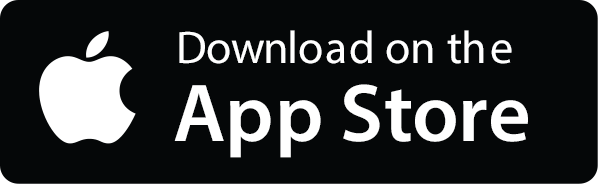
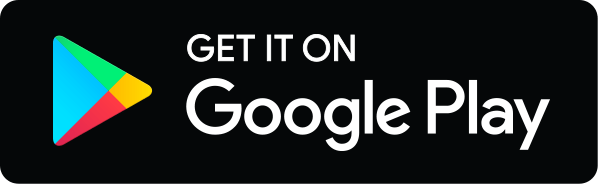
