CHAPTER 92 ACUTE RESPIRATORY DISTRESS SYNDROME
There have not been many topics in critical care medicine that have received as much as attention as the acute respiratory distress syndrome (ARDS). Recently, there has been significant medical progress in this area—due in part to a better understanding of the pathophysiology of ARDS as well as earlier diagnosis and initiation of therapy. The “open lung” concept of mechanical ventilation has revolutionized the management of these patients. Newer modes of ventilation and novel strategies to improve oxygenation and reduce lung compliance have also contributed substantially.1 Despite these advances, a 30%–40% mortality can be attributed to ARDS.2 ARDS can occur directly from traumatic chest trauma or as a sequela of a host of disease processes (sepsis, fat emboli syndrome, pneumonia, severe blunt chest trauma, and so on). Therefore, a thorough understanding of the clinical presentation and treatment options for ARDS is essential for every practitioner who manages critically ill patients.
EPIDEMIOLOGY
The first estimate of the incidence of ARDS, 75 cases per 100,000 person per year population correlating with 150,000 cases per year in this country, was published in an expert panel report by the National Institute of Health in 1972.3 The American European Consensus Conference (AECC) redefined ARDS in 1992. Recent studies based on the AECC criteria found incidence rates that varied from 4.9 to 22 per person years. More recent studies reported high incidence rate of ARDS among ventilated patients. In a large multicenter study including 5183 mechanically ventilated patients, 9% of patients met ARDS criteria at the beginning or over the course of the ventilatory support.4 In a more recent prospective study, Estenssoro et al.5 found that 8% of patients admitted to an intensive care unit (ICU) and 20% of mechanically ventilated patients fulfilled criteria for ARDS.
The reported rate of mortality from ARDS ranges from 31% to 74%. Several investigators have observed a reduction in mortality rates over time, from more than 60% in the 1980s to less than 40% in the 1990s.2,6 Most studies have indicated that nonsurvivors of ARDS usually die of nonrespiratory causes. In 1985, Montgomery et al.7 highlighted that only 16% of deaths were caused by respiratory failure. In most cases, early death was caused by underlying disease, whereas late death was caused by sepsis. Recently, Bersten et al.8 showed that respiratory failure was the cause of death in only 9% of ARDS cases. Thus, ARDS is a systemic disease and the main cause of death is related to multiorgan failure. It is interesting that degree of hypoxemia is unimportant in terms of predicting mortality. Valta et al.9 showed that age, right ventricular dysfunction, and the presence of acute renal failure were found to have important prognostic value.
DEFINITION AND CLINICAL DIAGNOSIS
The definition of ARDS has been simplified over the past few years, allowing clinicians to identify patients earlier. Ashbaugh’s 1967 original description consisted of respiratory distress, cyanosis, decreased lung compliance, and bilateral infiltrates on chest radiograph.10 In 1988, Murray and Mathay devised a four-point scoring system that included the level of positive end expiratory pressure (PEEP), the ratio of partial pressure of arterial oxygen to the fraction of inspired oxygen (PaO2/FiO2), lung compliance, and chest x-ray findings.11 In 1994, the AECC modified the 1988 definition and their new definition included the following major components12:
Acute respiratory distress syndrome presents with dyspnea, usually developing within 72 hours of the initial insult. Some patients may progress to moderate or severe respiratory failure necessitating intubation. Stable patients with mild to moderate ALI can be cautiously managed with a trial of noninvasive ventilation.13 Arterial blood gas will often reveal hypoxia with respiratory alkalosis. The diagnosis is confirmed by chest x-ray, which shows bilateral infiltrates resembling pulmonary congestion. Infrequently, the chest x-ray will have an atypical pattern that will be asymmetric or unilateral. Computer tomography of the chest, if obtained, shows consolidation with atelectasis in the dependent zones.14
Acute respiratory distress syndrome is associated with several clinical disorders, sepsis being the most common among them (40%).5 Two clinical disorders often found in trauma patients are pulmonary contusion and multiple blood transfusions, which can lead to ARDS. Pulmonary contusion is a direct insult to the lung parenchyma, leading to the accumulation of blood and proteinaceous fluid at the alveolar-capillary interface.15 This will lead to ALI in many patients, with some patients progressing to ARDS. Trauma patients requiring multiple transfusions of packed red cells are at risk for ARDS. Transfusion-related ALI (TRALI) accounts for a small percentage of cases of ARDS. The exact mechanism of TRALI is still unclear.
PATHOPHYSIOLOGY
Acute respiratory distress syndrome is a devastating form of acute respiratory failure that frequently develops in patients with pulmonary and nonpulmonary organ failure. Experimental and clinical data regarding the pathogenesis of disease have evolved significantly over the past decade. ARDS is an extremely severe form of ALI that occurs as a result of systemic inflammation caused by either direct or indirect lung injury. Direct lung injury is associated with high mortality rate. Some causes of direct lung injury include pneumonia, aspiration, and pulmonary contusion. Common causes of indirect lung injury are sepsis, multiple blood transfusion, shock, and acute pancreatitis.16 Regardless of the initial etiology, ARDS is characterized histologically by diffuse alveolar damage with interstitial and alveolar infiltration with neutrophils and macrophages. On the other hand, it is a progressive disease and has indistinct stages with different histologic features.
The acute exudative phase is manifested by the rapid onset of respiratory failure. Arterial hypoxemia that is refractory to oxygen is a characteristic feature. Pathologically, this picture is characterized by injury of the alveolar-capillary membrane and accumulation of protein-rich fluid with neutrophils, macrophages, and disruption of the endothelial-epithelial barrier—ending with the development of the pulmonary interstitial edema secondary to microcapillary circulatory injury.17 The resulting interstitial accumulation of fluid and protein impairs diffusion capacity and thus oxygenation. These changes are responsible for the decreasing pulmonary compliance and hypoxemia. Disruption of the alveolar epithelium and loss of types 1 and 2 pneumocytes lead to loss of the mechanical barrier integrity, leading to bacterial translocation and sepsis.
The key role of the activated neutrophils and macrophages has been established based on analyses of bronchoalveolar (BAL) fluid in the acute phase of ARDS.18 Several recent studies stressed that activated neutrophils and macrophages produce cytokines, including TNF-α, interleukins IL-1βm IL-6, IL-8, and IL-10 (all of these playing essential roles in pathophysiology of ARDS).19 Recovery from ARDS is characterized by the resolution of the alveolar edema and recuperation of the alveolar epithelial barrier. During the acute phase of inflammation, alveolar epithelial cells undergo apoptosis. Once recovery begins, type 2 cells proliferate—producing surfactant. The type 2 cells then differentiate to type 1 cells. After the acute phase, some patients make uncomplicated and rapid recovery—whereas others progress to the fibrotic stage, which has been observed early in the course of disease.20 The alveolar space filled with mesenchymal cells (producing procollagen 3 peptide, collagen, and fibronectin, along with new blood vessels) gives a histologic picture of fibrosing alveolitis associated with poor outcome and an increased risk of death.21
TREATMENT
The use of PEEP in the treatment of ARDS patients dates back to the original description of the disease.10 The effectiveness of PEEP is due to its ability to recruit lung units, thereby increasing functional residual capacity (FRC) and hence increasing lung compliance. PEEP also increases the proportion of alveoli actively engaged in gas exchange, leading to a decrease in shunt fraction.22 PEEP has a third advantage of protecting against ventilatorassociated lung injury (VALI) by preventing the derecruitment-recruitment cycle that ultimately leads to alveolar damage. There has been much interest in the past few years in determining the optimum level of PEEP (a PEEP level that yields maximum oxygenation without overdistending the lung).
The use of upper and lower inflection points on the pressurevolume curve has been advocated by some as a more accurate way of determining this PEEP level.23,24 This method is highly sophisticated, often requiring computer software designed to graphically display pressure-volume curves. Therefore, this method has not gained widespread appeal. The current recommendation is to adjust PEEP to a level at which the FIO2 can be decreased to less than 60% and oxygen saturation can be maintained at 88% or greater.25 The ARDS network recently conducted a trial comparing lower to higher PEEP levels and there was no difference in mortality between the two groups.26 The use of high PEEP in ARDS remains controversial.
A landmark study conducted by the ARDS Network compared ventilating ARDS patients with tradition tidal volume (12 ml/kg) with lower tidal volume (6 ml/kg). A 22% reduction in risk was seen in patients ventilated with lower tidal volume.27 No other single ventilator strategy has been shown to impact survival dramatically in ARDS. Additional recommendations derived from the ARDS Network study included keeping plateau pressure less than 30 mm Hg and adjusting the PEEP to keep to oxygen saturation between 88% and 95%. Together, these recommendations comprise the “open lung” strategy of management of patients with ARDS.
Permissive hypercapnia has emerged as another important tool in the treatment of ARDS patients. With permissive hypercapnia, the partial pressure of carbon dioxide (PCO2) is allowed to rise as high as 70 mm Hg—and in some cases higher. Several studies have shown that this level of PCO2 is well tolerated and that attempts to normalize the PCO2 by increasing the respiratory rate or using bicarbonate replacement are unnecessary and perhaps detrimental. Permissive hypercapnia is thought to be lung protective.28
Pressure-controlled ventilation (PCV) was once thought to provide an advantage over volume-controlled ventilation in ARDS patients. It has been suggested that the rapid inspiratory flow characteristic of PCV leads to better oxygenation. A prospective randomized trial failed to show that PCV was superior.29 Inverse ratio ventilation (IRV) is used to improve oxygenation by allowing a greater time for inspiration to occur, this allowing more time for oxygen exchange. A longer inspiratory time can lead to hypercapnia and auto-PEEP. Some studies have found a small benefit to IRV, but others have failed to show a survival advantage.30 Other newer modes of ventilation are being used for ARDS. One mode that has recently attracted attention is airway pressure release ventilation (APRV). In this mode, continuous positive airway pressure is delivered for prolonged periods with exhalation allowed to occur over a fraction of a second.31 APRV has been successful in improving oxygenation in ARDS in anecdotal reports, but it can result in profound hypercapnia and auto-PEEP. No data exists to show this newer modality increasing survival.
It is important for the critical care team caring for ARDS patients on mechanical ventilation to be mindful of the potential to create VALI. VALI is an insult that arises in the lung as a result of the ventilator pressures causing alveoli to overdistend. This leads to not only physical stress-related injury to the alveolus but to activation of the proinflammatory response.32 The main components of VALI are oxygen toxicity and “volutrauma.” VALI is often seen after attempts to ventilate ARDS patients with high tidal volumes and when higher levels of oxygen (>60%) are used unnecessarily. The ARDS patient must be managed with a strategy that minimizes VALI.
Alternative Therapies
Inhaled nitric oxide improves oxygenation in respiratory failure by several mechanisms. First, it selectively vasodilates the pulmonary capillaries of well-ventilated alveoli—leading to a reduction in shunt and decreasing pulmonary vascular resistance. Second, inhaled nitric oxide may have some anti-inflammatory properties.33 It is usually delivered at concentrations of 1 to 10 parts per million, although concentrations as high as 40 parts per million can be delivered without toxicity. Several randomized clinical trials failed to show an outcome benefit.34 To date, the use of inhaled nitric oxide in ARDS is confined mostly to patients who are severely hypoxemic despite maximal efforts with conservative therapy.
Prone positioning has the theoretical benefit of improving oxygenation by redistributing lung ventilation and perfusion. Other important mechanisms include improving ventilation mechanics and alleviating the medial compression of the lung by the heart and mediastinum. A recent multicenter randomized trial conducted by Gattinoni et al.35,36 showed improved oxygenation in ARDS patients who were ventilated in the prone position. No improvement in mortality was shown in this study. Prone positioning is associated with several complications, including risk of pressure necrosis of facial structures, difficulty assessing sedated patients, and the possibility of dislodging the airway. Currently, there is no recommendation advising the routine use of prone positioning.
High-frequency ventilation (HFV) is achieved with a special ventilator or oscillator designed to deliver tidal volume of 1 to 5 ml/kg at rates of 60 to 300 breaths per minute. Ventilating the lung at such high frequency and with small tidal volumes is believed to have a lung-protective effect that reduces VALI.37 Multiple clinical trials of HFV in ARDS patients have failed to show a survival benefit. Its use is highly individualized and is often applied to patients who do not respond to conservative therapies.38
Partial liquid ventilation is a process in which the lungs are filled with perflouro-carbon (PFC) liquid and ventilated with conventional mechanical ventilator. PFC is an inert liquid with high affinity for oxygen. Partial liquid ventilation improves oxygenation by distributing PFC throughout the lung, allowing gas exchange in areas where gaseous oxygen will not.39 Previous studies have shown some promise for this modality in children with severe respiratory failure.40 A recent randomized study performed by Kacmerak et al.,41 which compared conventional mechanical ventilation with partial liquid ventilation, failed to show outcome benefit. With no large randomized studies to show efficacy partial liquid, ventilation remains investigational but it has been used as a salvage therapy at some institutions.
Extra corporeal membrane oxygenation (ECMO) utilizes a mechanical membrane oxygenator to directly oxygenate blood while blood is circulated through the machine by roller pumps. This is an appealing strategy potentially allowing the lung to “rest” while continuing to maintain maximal oxygenation. This procedure is fraught with logistical difficulties and is not available at many medical centers. The procedure also carries the risk of injury to vasculature from insertion of venous and/or arterial cannulas and the risk of bleeding because full anticoagulation is necessary. Clinical studies have not shown a benefit to using ECMO.42 ECMO is reserved almost exclusively for patients who fail conservative therapy and cannot be oxygenated by any other method.
Enthusiasm for the use of surfactant arose as a result of its beneficial effects on neonatal respiratory distress syndrome.43 Attempts to apply this therapy to adults have not proven fruitful. A recent randomized study comparing aerosolized surfactant to placebo in ARDS patients failed to show improvement in survival or an increase in ventilator-free days.44 To date, surfactant has no role in the management of adult patients with ARDS.
A significant proportion of patients with ARDS will begin to develop fibrosis early as a result of a deranged healing process. These patients may exhibit poor lung compliance, elevated peak airway pressures, and pulmonary hypertension—and have a “honeycomb” appearance on chest x-ray indicating fibrotic lung. It has been postulated that corticosteroids may arrest this process and possibly reverse it. Clinical studies looking at high-dose steroid (methylprednisolone 30 mg/kg every 6 hours) failed to show improvement.45 A recent study conducted by the ARDS Network used low-dose methylprednisolone (2 mg/kg) tapered over 25 days.46 The steroid group had higher 60-day mortality but was shown to have improved oxygenation, increased ventilator-free days, and increased shock-free days. However, despite these benefits the ARDS Network did not advocate the use of corticosteroids for fibroproliferative ARDS.

Full access? Get Clinical Tree
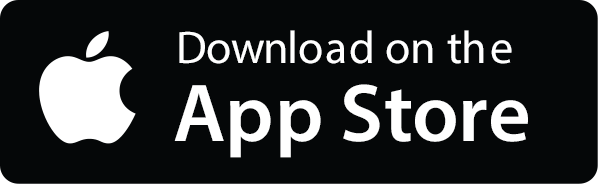
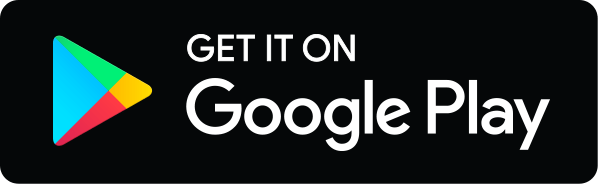