Despite many advances in the field of lung transplantation, lung transplant recipients have the lowest median survival of any solid organ transplant population. Complications such as reperfusion injury, graft rejection, infection, and anastomotic breakdown increase morbidity and mortality during the immediate postoperative period.
Ventilator management with lung protective strategies can not only minimize ventilator time and mitigate the risk of ventilator-associated pneumonia, but it may also decrease the risk of primary graft dysfunction and graft failure. Maintaining fluid balance, pain control, and preserving renal function also decrease postoperative complications.
Advancements in immunotherapy with the use of calcineurin inhibitors and monoclonal antibodies have been shown to decrease the incidence of acute rejection. However, when unexpected complications occur, appropriately timed rescue therapies such as the initiation of extra-corporeal membrane oxygenation, retransplantation, and plasmapheresis are important considerations geared toward a positive transplant outcome.
Acute postoperative period – a critical time for the lung transplant recipient
A variety of complications arise early in the postoperative period after lung transplantation, leading to 3-month mortality rates of 9–11% depending on the indication for transplantation . Recipients who survived to 1 year after the primary transplant had a conditional median survival of 8.0 years; therefore, treatment during the acute perioperative period can help maximize survival.
The most common causes of death within the first 30 days after lung transplantation are graft failure and non-cytomegalovirus (non-CMV) infections, causing 24.3% and 19.3% of deaths, respectively . Areas of interest are ventilator management, hemodynamics and fluid balance, immunosuppression, and diagnosis and management of acute allograft rejection.
A multidisciplinary team approach with close communication between the team members about the patient’s progress and plan is essential. As the clinical picture is continuously evolving, re-evaluation of the health care plan is needed. This involves not only hemodynamics, fluid balance, and respiratory care but also decisions regarding antibiotics, nutrition, immunosuppression, and diagnosis and management of allograft dysfunction.
Accurate and complete transfer of information during the transition of care between health care providers is critical. It provides the basis for a safe and smooth continuation of care. These high-risk patients cannot tolerate the slightest error.
The goal of hemodynamics management is to maintain an adequate blood pressure and optimize end-organ perfusion. While urine output is used as the standard reflection of perfusion pressure, a physical examination and measures of mixed venous oxygen saturation (SvO 2 ), lactate, and serum creatinine are adjuncts to the assessment of adequate blood flow to organs. Excessive blood volume or inotropic support resulting in a hyperdynamic right ventricle and the exacerbation of lung reperfusion injury should be avoided.
Norepinephrine and vasopressin are the preferred vasopressor therapy. If the SvO 2 is low and there is hemodynamic instability, transthoracic echocardiography is an excellent way to quickly rule out cardiac tamponade and evaluate left and right heart function. Dobutamine (low dose, 2–3 mcg/kg/min) may be considered the first drug of choice for inotropic support; however, it should be avoided for high heart rates (HR > 110). Milrinone should be used cautiously because it can cause systemic vasodilation and tachycardia. Epinephrine may cause increased pulmonary edema secondary to its hemodynamic effects of increased adrenergic activity .
Monitors
Monitoring in the ICU is similar to intraoperative monitoring. Most centers continue using arterial line, pulmonary arterial catheter, EKG, pulse oximeter, manometer, capnometer, and echocardiography in the early postoperative setting. The monitoring in the operating room is designed to evaluate acute perturbations in vital functions caused by the administration of medications and as a response to surgical manipulations. In the ICU, monitoring is quintessential as it provides information used in the diagnostic evaluation of a patient’s clinical status and the plan for their future care. Meticulous attention to preventing infections of indwelling lines over days of care and the performance of frequent line changes, the consistent adjustment of monitoring tools to provide precise reporting, and mobility of the patient with multiple monitoring tools in place are only a few examples of how monitoring in the ICU and OR differ.
Arterial blood gases should be performed regularly for early detection of acid–base, electrolyte, hematocrit, CO 2 , and oxygen level changes and to guide the appropriate adjustments. Although helpful in defining dead space and shunts and as a trend monitor, end tidal CO 2 (EtCO 2 ) does not accurately represent the pulmonary arterial CO 2 levels. Lung compliance and volume-flow loops are far more useful. Close monitoring of fluid administration and urine output is exceedingly important. In general, large volumes of fluids should be avoided as the donor lung is susceptible to edema . Echocardiographic monitoring provides useful information throughout the postoperative period and helps guide diagnosis and management. It is important to evaluate changes in right heart function and size, worsening of tricuspid regurgitation, and filling status of the left ventricle.
Appropriate monitoring aids in the early identification of postoperative complications and is the key to the successful management and treatment of the lung transplant patient postoperatively.
Monitors
Monitoring in the ICU is similar to intraoperative monitoring. Most centers continue using arterial line, pulmonary arterial catheter, EKG, pulse oximeter, manometer, capnometer, and echocardiography in the early postoperative setting. The monitoring in the operating room is designed to evaluate acute perturbations in vital functions caused by the administration of medications and as a response to surgical manipulations. In the ICU, monitoring is quintessential as it provides information used in the diagnostic evaluation of a patient’s clinical status and the plan for their future care. Meticulous attention to preventing infections of indwelling lines over days of care and the performance of frequent line changes, the consistent adjustment of monitoring tools to provide precise reporting, and mobility of the patient with multiple monitoring tools in place are only a few examples of how monitoring in the ICU and OR differ.
Arterial blood gases should be performed regularly for early detection of acid–base, electrolyte, hematocrit, CO 2 , and oxygen level changes and to guide the appropriate adjustments. Although helpful in defining dead space and shunts and as a trend monitor, end tidal CO 2 (EtCO 2 ) does not accurately represent the pulmonary arterial CO 2 levels. Lung compliance and volume-flow loops are far more useful. Close monitoring of fluid administration and urine output is exceedingly important. In general, large volumes of fluids should be avoided as the donor lung is susceptible to edema . Echocardiographic monitoring provides useful information throughout the postoperative period and helps guide diagnosis and management. It is important to evaluate changes in right heart function and size, worsening of tricuspid regurgitation, and filling status of the left ventricle.
Appropriate monitoring aids in the early identification of postoperative complications and is the key to the successful management and treatment of the lung transplant patient postoperatively.
Primary graft dysfunction
Primary graft dysfunction (PGD) is a form of acute lung injury encountered in the immediate postoperative period. It is sometimes referred to as reperfusion injury, although there is some evidence that autoimmunity plays a role . Commonly recognized characteristics of PGD are as follows:
- 1)
hypoxemia in the absence of left heart failure.
- 2)
hypoxemia with coincident infiltrates on chest radiograph.
- 3)
new diagnosis of pulmonary hypertension in the immediate postoperative period.
- 4)
otherwise unexplained respiratory failure.
The International Society of Heart and Lung Transplantation (ISHLT) has unified these diagnostic criteria in their consensus document for diagnosis of PGD ( Table 1 ).
Grade | PaO 2 /FiO 2 | Radiographic infiltrates consistent with pulmonary edema |
---|---|---|
0 | >300 | Absent |
1 | >300 | Present |
2 | 200–300 | Present |
3 | <200 | Present |
Up to 30% of all deaths occurring in the immediate postoperative period can be attributed to PGD . In a retrospective review of over 400 patients who underwent lung transplantation, high-grade PGD was associated with increased ICU length of stay and hospital length of stay . Moreover, PGD has been associated with higher incidence of bronchiolitis obliterans and chronic rejection .
Multicenter cohort studies have identified several independent risk factors for PGD, many of which are modifiable: .
- 1)
overweight and obesity.
- 2)
preoperative sarcoidosis or pulmonary hypertension.
- 3)
use of cardiopulmonary bypass.
- 4)
single lung transplant.
- 5)
and increased FiO 2 during allograft reperfusion.
- 6)
Blood products administered during surgery may also pose an increased risk of development of PGD.
- 7)
Donor age less than 21 or greater than 45 years, female gender, smoker, African American origin.
Early graft failure (EGF) is a term used to describe the most severe subset of patients with early graft dysfunction resulting in graft loss. From 2005 to 2013, EGF occurred in 2.3% of patients who underwent lung transplant, leading to retransplantation in 7% and death in the remaining 93% of cases. Of note, patients who underwent retransplantation had a higher incidence of EGF than primary lung transplantation (4.1% versus 2.2%) .
The preoperative characteristics of the transplant recipient are discussed in detail in McCartney et al. (Long Term Outcomes and Management of the Heart Transplant Recipient) and Prabhu and Valchanov (Pre-Anesthesia Evaluation Of The Patient With End-Stage Lung Disease). However, it is important to emphasize that a rapid deterioration of the patient’s clinical condition or the progression of the recipient to a premorbid state prior to transplantation has a tremendous effect on the development of EGF, morbidity, and mortality.
Prevention of PGD focuses on minimizing inflammation and edema during the donation process. Minimizing organ ischemia time and avoiding barotrauma to the lung are obvious strategies. More controversial strategies during organ donation include administration of corticosteroids as an immunosuppression agent and to decrease inflammation and conservative fluid management and diuretic use targeting central venous pressure <10 mmHg or extrapulmonary lung water index <10 cc/kg to protect against pulmonary edema . To ensure hemodynamic stability, early administration of vasoactive drugs and administration of thyroid hormone have been proposed .
Treatment of primary graft dysfunction
Protective lung ventilation strategy
Treatment of PGD is supportive. Low tidal volume and high positive end-expiratory pressure (PEEP) levels may help improve oxygenation—these strategies are similar to the treatment of acute respiratory distress syndrome, as discussed below in the section on ventilator management.
Pulmonary vasodilators
The incidence of PGD is correlated with a marked decline in endogenous nitric oxide and cyclic guanosine monophosphate levels. Therefore, the administration of inhaled nitric oxide (iNO) during lung transplantation has been proposed as a possible therapeutic treatment to prevent or attenuate PGD pathogenesis. There is evidence that iNO administration during PGD can improve oxygenation and reduce pulmonary hypertension without altering systemic vascular resistance. There are currently no randomized controlled studies that demonstrate a reduction in morbidity [time to extubation, length of intensive care unit (ICU) or hospital stay] or mortality. Thus, it is difficult to recommend the routine use of prophylactic iNO in lung transplant surgery . Iloprost has also been used as a selective pulmonary vasodilator .
Extra-corporeal membrane oxygenation
Early institution of extra-corporeal membrane oxygenation (ECMO) has been shown to improve the overall outcome in some cohorts .
Veno-venous (VV) ECMO is an option for patients experiencing severe PGD when lung protective strategies cannot fully support the patient’s ventilator needs. This strategy allows for low tidal volume ventilation and minimal sedation . VV ECMO decreases the patient’s reliance on the lungs for gas exchange as oxygen delivery and carbon dioxide extraction occur in the ECMO circuit. The combination of VV ECMO and ultra-protective ventilation (3 cc/kg tidal volume) may limit ventilator-induced lung injury (VILI) and increase the number of ventilator-free days in lung transplant recipients with severe PGD. This concept has been studied in the ARDS population with encouraging results . Oxygen supply by the pulmonary arteries to the graft is crucial as bronchial arteries are lacking. Low saturation of the venous admixture may result in pulmonary and systemic hypoxia.
Veno-arterial (VA) ECMO is indicated in the case of hemodynamic instability or severe post-transplant pulmonary hypertension to protect the new lung from hyperperfusion resulting in pulmonary edema aggravated by the absence of lymphatic drainage, which hampers the clearance of the lung interstitium. A downside of VA ECMO therapy is that it shunts blood away from the lungs and can potentially decrease perfusion to the allograft itself. The resultant potential for graft hypoxia is of serious concern and can lead to PGD and possibly graft failure.
VVA ECMO is a combination of the two techniques that allows the possibility to regulate the amount of blood flow through the lungs and systemic oxygenation.
Retransplantation
Retransplantation raises many of the same considerations as the initial transplantation, with higher incidence of complications such as PGD, rejection, and infection . The most common reasons for retransplantation are bronchiolitis obliterans syndrome (BOS, 63%), PGD (15%), and acute rejection (4%) .
Postoperative ventilator management
The goal of ventilator management in the early postoperative period is to avoid respiratory acidosis and maintain adequate oxygenation. Our routine is to institute EtCO 2 monitoring immediately on admission to the ICU and maintain it while the patient is on mechanical support. Routinely, patients are mechanically ventilated for the first postoperative night to monitor for reperfusion injury. Dead-space calculation, chest X-ray, EtCO 2 -PaO 2 gradient, and EtCO 2 trend are fundamental to that assessment.
Optimal ventilator management will minimize the risk of pulmonary infection and PGD. No large multicenter trials exist to guide ventilator management. In the IMPROVE study, patients in a general surgical population who were treated with brief periods of intraoperative lung-protective ventilation had lower rates of lung injury and even shorter length of stay than those who received standard of care ventilation strategy . With the emerging data showing the benefit of lung-protective ventilation in populations with and without ARDS, many centers have adapted this strategy for the perioperative ventilator management of lung transplant recipients.
Survey data of postoperative ventilator management after lung transplant show that most centers aim for short ventilator times: 32% of respondents estimated ventilator time to be less than 24 h, 36% estimated it was 24–36 h, and 16% estimated that it was 36–48 h . Short ventilator time is optimal because it lowers the risk of ventilator-associated pneumonia (VAP) and limits VILI . Survey respondents also report a mean target for oxyhemoglobin saturation of 91.5% or greater and a mean target PaO 2 of 69.5 mmHg. The majority of respondents (91%) reported routinely assessing and minimizing airway pressure, while many also reported a maximum acceptable PEEP. These practice patterns model the lung-protective ventilation strategy with special care to prevent VILI.
After lung transplantation, the bronchial circulation is typically not restored, putting the patient at risk for ischemia of the transplanted airway and airway mucosa. Thus, bronchial anastomosis sites are prone to poor healing, infection, and anastomotic airway complications. Anastomotic airway complications, including infection, stenosis, and dehiscence, occur in approximately 10–20% of lung transplant recipients . Positive-pressure ventilation could potentially impair perfusion to transplanted airways, especially when high airway pressures are required.
Special consideration for single lung transplant
Ventilator settings should account for donor height as hyperventilation of an undersized allograft increases the risk of EGF, PGD, prolonged intubation, and tracheostomy and increases resource utilization . Lung-protective ventilation for undersized allografts may prevent VILI, although no trials have investigated this issue.
It is important to remember that the native lung has end-stage disease, either obstructive or restrictive, and therefore has different respiratory mechanics compared to the allograft. A large percentage of the tidal volume will go to the more compliant lung.
In obstructive lung disease, e.g., chronic obstructive pulmonary disease (COPD), the native lung is more compliant, leading to over-distention and auto-PEEP. The compliance difference may be magnified by edema in the newly transplanted lung; therefore, the incidence of acute hyperinflation in single lung transplants with COPD may be as high as 30% . Ventilator management strategy should accommodate for the diseased native lung in order to prevent severe hyperinflation of the native lung, mediastinal shift, and compression of the transplanted lung. Appropriate ventilator settings include long expiratory time (6–13.5 s), short inspiratory time, low PEEP (5 cm H 2 O or less) and low respiratory rate (4–8 breaths per minute). If severe dynamic hyperinflation occurs, a rescue maneuver can be performed by briefly disconnecting the patient from the ventilator, thus resolving the culmination of pressure. If repeated severe hyperinflation events occur, independent lung ventilation using a double-lumen endotracheal tube should be started. This strategy will allow for protective ventilator strategy to be applied to the graft, while the native lung can benefit from the ventilator management strategies listed above.
Recipients of a single lung transplant for interstitial pulmonary fibrosis (IPF) can have an IPF flare in the native lung triggered by the lung surgery, leading to lower compliance.
Postoperative considerations
Atrial arrhythmias
Atrial arrhythmias (AAs) are a common occurrence after thoracic noncardiac surgery, with an incidence of 20–40% . The incidence of AAs is higher after lung transplant than after heart transplant, in part because of cardiac autonomic denervation and because of surgical manipulation of the pulmonary veins and left atrium .
AAs are not a benign finding. A recent meta-analysis examining the relationship between postoperative AAs and mortality in lung transplant recipients found that patients who had postoperative AAs had significantly higher perioperative mortality, longer hospital length of stay, more frequent requirement for tracheostomy, and higher mortality in years 1–6 .
Considering the possible adverse effect of AAs, aggressive treatment is warranted. The 2014 American Association for Thoracic Surgery guidelines suggest management that includes avoidance of postoperative beta blocker withdrawal, potential prophylaxis with calcium channel blockers or amiodarone in high-risk patients, an individualized approach to rate versus rhythm control, and anticoagulation for postoperative AAs >48 h in duration . Of note, Orrego et al. found increased mortality in patients whose postoperative AAs were treated with antiarrhythmics . This may reflect the association between amiodarone and increased mortality and LOS after lung transplantation.
Gastroesophageal reflux
There is a high prevalence of gastroesophageal reflux (GER) after lung transplantation . In the acute postoperative period, the concern with GER is that it may lead to THE aspiration of gastric contents and provoke damage in the lung allograft. The increased prevalence of GER in the advanced lung disease patient population appears to be associated with the presence of esophageal dysmotility along with a hypotensive lower esophageal sphincter in almost 80% of patients and associated with delayed gastric emptying in 44% of patients .
In addition to pre-existing GER, vagus nerve injury during surgery can cause delayed gastric emptying. As many as 90% of the post-lung transplant patients have experienced delayed gastric emptying . Cystic fibrosis patients have the highest prevalence of increased acid GER on monitoring (90%) . GER is less prevalent in patients with COPD and IPF (57% and 67%, respectively) .
The high prevalence of GER necessitates prophylactic therapy to limit gastric secretion. Commonly used agents are proton-pump inhibitors and H2 histamine receptor blockers. Prokinetic agents, such as macrolide antibiotics, may also play a role in inhibiting acid exposure to the allograft. In a cross-sectional study, patients receiving azithromycin experienced significantly fewer acid reflux events and had decreased esophageal acid exposure .
Preoperative fundoplication surgery prior to lung transplantation or in patients awaiting transplantation yielded no benefit in lung function .
Acute renal insufficiency
The risk factors for early postoperative acute renal failure following lung transplantation include the following :
- 1.
Effective volume contraction.
- 2.
Over-aggressive diuresis.
- 3.
Ventricular dysfunction.
- 4.
Acute tubular necrosis.
- 5.
Acute interstitial nephritis.
- 6.
Peri-operative sepsis.
- 7.
Hypotension.
- 8.
Radiographic contrast dye.
- 9.
Nephrotoxic drugs (immunosuppressives, etc.).
- 10.
Calcineurin inhibitors.
- 11.
Atheroembolism.
Optimizing fluid management before and after transplantation has the advantage of preventing volume depletion and maintaining adequate levels of renal perfusion. Attention should be given to avoid all potentially nephrotoxic insults. There is no evidence that continuous dialysis has any advantage over intermittent dialysis in heart or lung transplantation . Renal insufficiency after lung transplantation increases the complexity of care and, not surprisingly, increases morbidity and mortality among lung transplant recipients .
Bleeding
Bleeding of >1 L overnight or 200 cc/h is considered excessive and will most likely result in re-exploration in the operating room. Blood transfusion has no effect on survival, but blood product administration can lead to transfusion-related acute lung injury . In addition, platelet administration may lead to thrombosis at vascular anastomoses, although no studies have shown an association between platelet administration and thrombosis in lung transplant patients. Interestingly, lung function at 6 months is significantly better for patients who were transfused with more blood products .
Thromboembolism
Because of absent collateral bronchial circulation and resultant risk of pulmonary infarct, pulmonary embolism can be a devastating complication to a lung transplant recipient. Thromboembolic complications occur at a rate of 12% during the first 3 years after lung transplantation. No studies have attempted to quantify the incidence of thromboembolic complications during the acute postoperative period, possible because the incidence is very low and DVT prophylaxis is standard. Subcutaneous heparin injections, 5000 U every 8 h, are an adequate dose for prophylaxis in most patients.
Surgical nerve injury
Phrenic nerve injury may result from stretching or direct instrumentation of the nerve during thoracic surgery. It typically manifests as hemidiaphragm paralysis. The incidence of phrenic nerve dysfunction following lung transplant varies from 8% to 30% . In a case series of 27 patients by Sheridan et al., the right phrenic nerve was most commonly injured (78%) and the incidence of injury was higher for bilateral lung transplantation. Phrenic nerve paralysis should be considered a cause of postoperative respiratory failure in the absence of graft failure or infection.
Sedation and pain considerations
Dexmedetomidine infusion can be started to ensure adequate comfort and avoid excessive sedation. Fentanyl at low doses (25–50 mcg/h) is added for pain control. This is done particularly in patients without a thoracic epidural for postoperative pain relief. The use of low-dose dexmedetomidine avoids emergence agitation and delirium and facilitates smooth tracheal extubation .
Postoperative pain control involves a multimodal approach. Ketamine, gabapentinoids, acetaminophen, and nonsteroidal anti-inflammatory medications have been shown to be effective.
If needed, pain control procedures such as placing neuraxial catheters (epidural, paravertebral catheters) may be performed postoperatively in the ICU. To safely place these catheters, the patient should be awake, oriented, and conversant. Thus, many believe that intravenous analgesics may be successfully continued as the procedural risk of placing these catheters may not be warranted.
Infection
VAP and ventilator-associated tracheobronchitis (VAT) are the most common types of infections, most dangerous to the allograft site, and temporally occurring as bacterial infection within the first several weeks after transplant . Specifically, gram-negative rods cause the majority of infections in the first month after surgery, followed by gram-positive cocci and then by viruses and fungi, causing infections most commonly between 100 and 400 days after surgery.
Interestingly, neither donor nor recipient colonization was significantly associated with a higher incidence of ventilator-associated respiratory infection. Even in studies of patients with cystic fibrosis, who are often colonized with multi-drug resistant organisms prior to surgery, there appears to be no association between preoperative colonization and postoperative respiratory infection. Patients who develop postoperative respiratory infections require longer mechanical ventilation (+43 days), longer ICU stay (+42.5 days), and longer hospital stay (+35 days) and experience higher in-hospital mortality .
Viral infections are uncommon in the immediate postoperative period, but the prevalence and impact of disease dictates the need to initiate prophylaxis in the early postoperative period. CMV infection and disease commonly occur in lung transplant patients and may play a crucial role in the short-term function of the allograft . CMV prophylaxis with valganciclovir or ganciclovir is typically instituted during the immediate postoperative period in patients who are susceptible to CMV infection depending on the donor and recipient CMV status.
Although bacterial and viral infections are more common, invasive fungal infections are associated with higher mortality . Risk factors for the development of fungal infection include IPF, older age, increased BMI, and increased lung allocation score.
Pneumocystis jirovecii prophylaxis with trimethoprim-sulfamethoxazole (TMP-SMX) is commonly instituted after all solid organ transplants because of the high incidence of pneumocystis pneumonia among immunocompromised patients . No consensus exists about the duration of prophylactic treatment, but prophylaxis should be started as soon as stable renal function is established in the immediate postoperative period—TMP-SMX is excreted through the kidney and can cause worsening renal function in the perioperative setting. Dapsone is a reasonable option if the patient is unable to tolerate TMP-SMX.
Antibiotic prophylaxis varies from center to center but typically consists of at least 72 h of gram-positive and gram-negative coverage commenced upon the induction of anesthesia .
Acute allograft rejection
The ISHLT reports that 34% of lung transplants encounter acute allograft rejection in the first year after transplant, with 89% of these episodes receiving some form of treatment . Graft rejection in the immediate postoperative period is believed to occur by one of two distinct mechanisms: acute cellular rejection (ACR) or antibody-mediated rejection (AMR). AMR is uncommon owing to cross-match screening. Acute rejection is rarely fatal, but it is a major risk factor for the development of BOS . Hyperacute rejection can still be seen in the first few hours of transplantation.
Patients may be asymptomatic; complain of dyspnea, cough, or sputum production; or experience acute respiratory failure. Findings of ground glass opacities, septal thickening, volume loss, and pleural effusions on high-resolution computed tomography (CT) scans suggest acute rejection . However, chest radiography and CT have low sensitivity and specificity for acute rejection . Bronchoscopy with biopsy is the most important diagnostic modality for acute allograft rejection .
Pulse steroids are used to treat ACR and AMR and have been shown to improve the clinical symptoms associated with acute rejection . In the setting of acute rejection, switching immunosuppression agents can be advisable. Several studies support switching from cyclosporine to a calcineurin inhibitor, typically tacrolimus . Alemtuzumab has been shown to be useful in patients who previously failed anti-thymoglobulin therapy .
AMR may be resistant to steroids, but in several cases, it responsive to plasmapheresis, suggesting an antibody-mediated process. Plasmapheresis is the best treatment for patients with pulmonary capillaritis unresponsive to steroids. Intravenous immunoglobulin (IVIG) may also be considered. IVIG causes B-cell apoptosis, reduces B-cell numbers, and may inhibit complement activation . Rituximab, an anti-CD20 monoclonal antibody that causes B-cell depletion, has been proven effective in the treatment of renal transplant recipients in conjunction with IVID . Rituximab plus IVIG can clear newly acquired antibodies, leading to freedom from BOS and better overall survival .

Full access? Get Clinical Tree
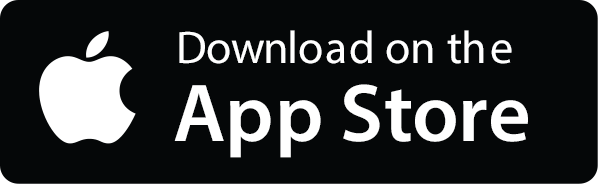
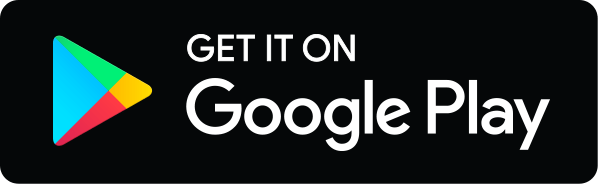