Acid and Base Physiology
Aaron Kirsch
Jeffrey Kirsch
▪ INTRODUCTION
There is a vast amount of water in the human body, distributed as the cytoplasm within cells (intracellular fluid) and the extracellular fluid, which includes plasma. Dissolved in all of this water are a wide variety of solutes. Among the most important solutes are acids and bases. Acids are compounds that, when dissolved in water, will donate a hydrogen ion (H+). Bases are compounds that, when dissolved in water, will accept an H+ from an acid. Examples of acids include hydrochloric acid (HCl) or carbonic acid (H2CO3); examples of common bases include ammonia (NH3) or sodium hydroxide (NaOH).
We measure the concentration of acid in the body fluids using the pH scale. The pH of a solution is defined as follows:

where [H+] is the concentration of H+.
If there is an abundance of acid in the fluid, there will be a high [H+]. This corresponds to a low pH. Therefore, the lower the pH measurement, the more acidic is the fluid. If there is an abundance of base in the solution, there will be a relatively lower [H+], because bases will eagerly accept and incorporate free H+. This corresponds to a high pH. Therefore, the higher the pH measurement, the more alkaline (basic) is the fluid. In chemistry, a pH of 7 is considered neutral, a pH less than 7 is considered acidic, and a pH greater than 7 is considered alkaline. In human physiology, however, the optimal pH of arterial blood is 7.4 or slightly alkaline. Venous blood will have a lower pH, as it is carrying waste from the periphery for removal by the lungs, kidneys, liver, and bowel.
The balance between acids and bases is crucial to proper body functioning. Even slight deviations from a pH of 7.4 can result in serious pathologic consequences. For example, reduction in pH to less than 7.0 can be fatal. This is because the organs and tissues of the body depend on the functionality of their cells. The functional actors in cells are enzymes, special proteins that catalyze chemical reactions. Alterations to the body’s acid-base balance change the chemical structure of enzymes, rendering them dysfunctional. This may result in organ malfunction. When the pH of the plasma is less than 7.4, the plasma is acidotic. When the pH is greater than 7.4, it is alkalotic.
▪ PHYSIOLOGIC CONSEQUENCES OF ACID-BASE DISTURBANCES
In the operating room (OR), acidosis produces its most significant and obvious effects on the cardiovascular system. As pH drops to 7.2, there is a noticeable reduction in heart muscle contractility and at a pH of 7.1, the entire cardiovascular system becomes much less responsive to catecholamines, making it very difficult for the anesthesiologist to treat hypotension with commonly used agents (e.g., norepinephrine, phenylephrine, ephedrine). Acidosis also causes hypotension due to peripheral arteriolar dilatation and constriction of the pulmonary arteries. Importantly, acidosis results in shifting of potassium out of cells and into the general circulation, which then may result in additional cardiac dysfunction.
▪ RESPIRATORY ACID-BASE DISTURBANCES
The acids in the body come from a variety of sources. When proteins are metabolized, weak acids are released into the bloodstream. But the main contribution of acid comes from a gas. When cells metabolize fats and carbohydrates, carbon dioxide (CO2) gas is produced. Inside of cells, this CO2 dissolves in the cytoplasm, producing H2CO3.
The enzyme carbonic anhydrase (CA) rapidly converts this H2CO3 into H+ and HCO3– (bicarbonate), which can be illustrated as follows:

As illustrated above, CO2 production results in an increase in [H+]. This translates into a lower pH. Therefore, excess CO2 leads to acidosis. If CO2 were not removed from the body, acid would build up and pH would decrease dramatically. Fortunately, the CO2 produced in metabolism is transported in the bloodstream to the lungs. Here, it is removed from the blood in exchange for oxygen (O2) and is breathed off into the environment. CO2 travels in the blood in three forms. The majority of CO2 dissociates into H+ + HCO3–. The H+ binds to hemoglobin molecules (Hb·H+), while the HCO3– travels freely in the plasma. Once at the lungs, the H+ and HCO3– recombine to form CO2, where it is exhaled. A small amount of CO2 also travels as dissolved CO2, and a small amount binds directly to hemoglobin (Hb·CO2).
When acidosis is caused by the lungs being unable to breathe off CO2, it is termed respiratory acidosis. When alkalosis is caused by the lungs breathing off too much CO2, it is termed respiratory alkalosis. Respiratory acidosis occurs when ventilation is impaired. For instance, the diaphragm or intercostal muscles may be paralyzed or too weak. A paralyzed diaphragm can be commonly observed following interscalene blockade, whereas intercostal muscles become weak in patients with a high spinal anesthetic. Patients who are administered opiate drugs will also have a predictably depressed breathing rate. Patients with respiratory acidosis may appear anxious or delirious, or even have myoclonic convulsions or seizures in extreme cases. The acidosis is best treated by increasing ventilation (breathing rate), by treating the underlying cause, or, if necessary, by mechanically ventilating the patient (oxygen treatment). Other common causes of respiratory acidosis include pulmonary disease that impairs gas exchange (e.g., chronic obstructive pulmonary disease). Respiratory acidosis also occurs when there is excess production of CO2, beyond the ability of the patient’s ventilatory ability. For example, in patients who are anesthetized and mechanically ventilated (i.e., have a fixed ventilation), excess CO2 production may occur from high fevers or malignant hyperthermia. Decreased ability to clear CO2 from the lungs may occur secondary to extremely compromised pulmonary function.
Respiratory alkalosis occurs due to hyperventilation (breathing too fast). This happens in patients suffering anxiety attacks. It is also seen in patients with pulmonary embolisms (blood clots that typically travel from the legs to the lungs), liver failure, pregnancy, and an overdose of aspirin. Patients might present with arrhythmias (irregular heartbeats), muscle cramps, tingling sensations, or even seizures. Although correcting the underlying cause is essential, hyperventilation can be urgently treated with sedating drugs if necessary.
Oxygen (O2) is another important gas in maintaining acid-base balance. It is inhaled from the environment and enters capillaries in the lungs in exchange for CO2. If breathing is impaired, O2 cannot enter the lungs to be exchanged with CO2. As shown above, the buildup of CO2 in the blood results in acidosis. Furthermore, O2 is essential for aerobic metabolism. When cells lack O2, they produce energy via anaerobic glycolysis. Prolonged glycolysis causes the accumulation of lactic acid. This results in further acidosis. Low O2 supply to the tissue may result from systemic hypoxia (e.g., high altitude, poor pulmonary gas exchange) or compromised circulation to an individual body region (local ischemia). Global ischemia may be caused by overall poor circulation (e.g., cardiac failure or severe hypotension), while local ischemia may be secondary to surgical intervention (e.g., aortic cross-clamp, limb tourniquet) or patient disease (arterial blood clot, peripheral vascular disease, trauma, etc.).
▪ ARTERIAL BLOOD GASES
Because O2 and CO2 are important factors in determining acid-base balance, being able to measure their partial pressures (concentrations) in the bloodstream can provide valuable insight into the physiologic condition of the patient. This is accomplished by arterial blood gas measurement (ABG). A sample of blood is drawn from an artery and is placed into a blood gas analyzer device (See Chapter 37). This device uses a variety of electrochemical probes to measure the pH and the partial pressures of O2 and CO2. It is important to understand that the [HCO3–] is
calculated and not directly measured by most blood gas machines. Assessment of a patient’s acid-base status from a reading on the blood gas analyzer can depend on the temperature of the patient and the temperature setting on the machine performing the measurement. Two different approaches exist: “alpha-stat” and “pH stat.” In blood, the pH changes inversely with temperature. Thus, at temperatures below 37°C, a pH of 7.4 would be considered acidotic. With the pH-stat approach, the anesthesia technician will run the blood sample entering the patient’s temperature into the blood gas machine, while with the alpha-stat approach, all blood gases are run at 37°C. Since there is great controversy among anesthesiologists regarding the best approach (alpha or pH-stat), the anesthesia technician should ask anesthesiologists their preference before analyzing the sample blood on the blood gas machine.
calculated and not directly measured by most blood gas machines. Assessment of a patient’s acid-base status from a reading on the blood gas analyzer can depend on the temperature of the patient and the temperature setting on the machine performing the measurement. Two different approaches exist: “alpha-stat” and “pH stat.” In blood, the pH changes inversely with temperature. Thus, at temperatures below 37°C, a pH of 7.4 would be considered acidotic. With the pH-stat approach, the anesthesia technician will run the blood sample entering the patient’s temperature into the blood gas machine, while with the alpha-stat approach, all blood gases are run at 37°C. Since there is great controversy among anesthesiologists regarding the best approach (alpha or pH-stat), the anesthesia technician should ask anesthesiologists their preference before analyzing the sample blood on the blood gas machine.
When obtaining a patient’s vital signs during an operation or at the bedside, oxygen saturation (“pulse ox”) is often measured by clipping a pulse oximeter probe to the fingertip (see Chapter 33). Both the red and infrared waves emitted by this probe allow for the measurement of the amount of hemoglobin in the blood that is bound to the oxygen (Hb·O2). This defines oxygen saturation (SaO2). Although this gives us a good estimate of oxygenation, it does not tell us anything about CO2 or acid-base balance. This is why we must examine the ABG.
ABG is obtained in the following manner. First, you must evaluate the arteries of the patient. Because of ease of access and the presence of a redundant circulation to the hand via the ulnar artery, the radial artery is typically used. You can palpate the radial artery pulse by placing one or two fingers just proximal (above) to the hand on the anterior-lateral (front, thumb side) surface of the wrist. Next, some clinicians try to assess the collateral circulation of the hand. They argue that if the radial artery is damaged during the procedure, it is important to be assured that collateral circulation to the hand via the ulnar artery is intact. The test that is done is called the Allen test. Those who use this test will choose an alternate site (e.g., femoral artery) for drawing an ABG if the Allen test demonstrates poor collateral circulation in the hands. However, a number of authors have demonstrated that the Allen test has very limited predictive value, regardless of whether it demonstrates good or poor collateral circulation via the ulnar artery. Therefore, this test is not used very often in clinical practice. In order to perform the Allen test,
The patient should extend the hand at the wrist
The patient should make a fist
The clinician should compress both the radial and ulnar arteries at the patient’s wrist
Have the patient open and close a tight fist several times
The palm should appear white, as it is not receiving any blood flow
Release your hold on the ulnar artery, restoring ulnar blood flow
If there is good ulnar circulation, the palm should become red within 5-10 seconds (maximum of 15 seconds) after restoring ulnar blood flow. A palm that remains white constitutes a failed Allen test, meaning that ulnar flow is too poor for an ABG to be measured in this hand.
If your hospital has preassembled kits for ABG measurement, they should be used. A 23-gauge (or smaller) needle is used, along with a preheparinized syringe, containing 30-100 units of heparin per milliliter of blood to be obtained. A syringe with too much heparin risks diluting the sample. When preassembled kits for ABG

Full access? Get Clinical Tree
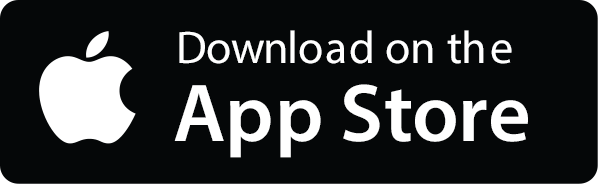
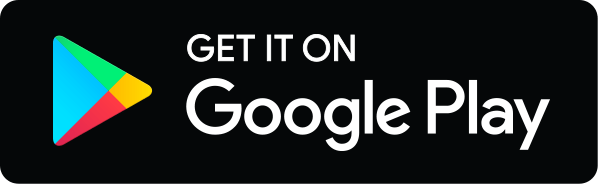