Fig. 7.1
Representation of the oxygen pathway, from air to tissues, in mammals
The index of availability of oxygen for the tissues/organs is DO2 (oxygen delivery), that is, the product of cardiac output (CO) by the content of oxygen in arterial blood (CaO2). This value can be obtained knowing the arterial hemoglobin saturation (SaO2), since CaO2 = SaO2 × Hb × 1.34 (i.e., the oxygen-carrying capacity of Hb in mlO2/g Hb) + O2 dissolved in plasma. The amount of dissolved gas is negligible when Hb concentration is not very low and air is inhaled.
In a healthy resting adult, DO2 ranges from 800 to 1,200 ml/min, while global oxygen consumption (VO2) is 200–300 ml/min. The oxygen extraction ratio (O2ER), representing the ratio VO2/DO2, is thus 20–30 % and a large reserve exists for metabolic demand. When DO2 is reduced for any reason affecting the oxygen pathway, enclosed acute normovolemic anemia, VO2 remains mostly stable (unless fever, anxiety, or other causes of increased oxygen consumption are present), resulting an increased O2ER. Lowering further the DO2, a certain point is reached (DO2 CRIT), where VO2 begins to decrease being O2ER not increasable (value at peak 60–70 %). Then, oxygen is not sufficient anymore for the cell aerobic metabolism, hypoxia develops, and an anaerobic metabolism takes over, with a low production of ATP, unable to meet the tissue demand and lactate is released. Theoretically, DO2 CRIT is one of the real, physiological trigger points. Therefore, we should be able to point at this key index, to guide any goal-directed therapy, including blood transfusion.
Nevertheless, it is to be considered that diverse DO2 CRIT can coexist in an organism, because of reduced DO2 and/or O2ER at regional level, where an ischemic injury can develop in some organs, in the absence of global markers of hypoxia.
On the basis of what is described above, optimization of transfusion therapy would require reliable markers of hypoxia in tissues. Currently, because of the lack of validated, practicable markers of tissue hypoxia, we refer to surrogate ones. So, traveling back on the O2 pathway (Fig. 7.1), the first element easy to derive (in quantitative terms) is hemoglobin concentration in blood. Therefore, it is obvious that, over last decades, a great effort has been put in research in the attempt to validate such an inexpensive and quickly obtainable indicator as a transfusion trigger. Nevertheless, the predictive value of determinate Hb levels as transfusion thresholds is high in patients who are young and without known comorbidities and become lower when other factors are present that can affect the oxygen pathway at any level.
A feasible index that can be integrated with Hb concentration is quantification of arterial hemoglobin saturation, (SaO2), investigating the first part of the O2 pathway, from inhaled air to diffusion toward pulmonary capillaries. SaO2 measurement is effected by means of a pulse oximetry that gives a reliable esteem and is practicable in any ward.
Doing so, we still miss any information about the real amount of oxygen transferred to the tissues. As already mentioned, more refined indicators of tissue hypoxia, defined “physiological trigger points,” have been developed to address more closely questions related with the oxygen utilization by the tissues, in order to guide a goal-directed therapy. On the basis of what is described above, ideal investigation on the critical part of O2 pathway would require measurement of the key factor O2ER. This is indirectly obtainable through measurement of mixed venous O2 saturation (SvO2), being O2ER = (SaO2 − SvO2)/SaO2. Targeting O2ER for goal-directed therapy has shown, when integrated in an algorithm of treatment, its capability to guide clinical decision and to increase the rate of favorable outcomes (Donati et al. 2007). SvO2 measurement requires pulmonary artery catheterization (PAC), by means of a Swan-Ganz catheter, but because of low feasibility and risks associated with this maneuver, it has been almost abandoned. Through a more practical central venous catheter, it is possible to measure the ScvO2 (central venous O2 saturation). ScvO2 has been advocated to be close to SvO2, only slightly overestimating, according to some authors (Reinhart et al. 2004), but presenting a more variable relationship (Dueck et al. 2005) and not fully substitutable to SvO2, according to other authors (Chawla et al. 2004). Therefore, extending its use has required an entirely new step of validation as a marker of global tissue hypoxia and tool for goal-directed therapy. Most, but not all, studies pointing at ScvO2 as fundamental indicator for monitoring clinical conditions and therapy have shown its effectiveness in diverse critical illness (see van Beest et al. 2011). Moreover, its perioperative use for goal-directed therapy in high-risk surgery has been found to be effective in predicting complications (Pearse et al. 2005a; Boyle et al. 2014) and in improving clinical outcomes (Pearse et al. 2005b; recently reviewed by Arulkumaran et al. 2014). Therefore, ScvO2 appears to be one of the best candidates to guide the goal-directed therapy in ICUs. Still, its intrinsic main limit is related to the requirement in invasive procedures, and currently it is not usable in most settings, such as in uncomplicated surgery.
Nevertheless, the value of ScvO2 in monitoring goal-directed therapy in ICUs cannot overshadow other more “traditional” measures, such as blood pressure and heart rate monitoring, diuresis control, pulse oximetry, ECG, EEG, central venous pressure, serum lactate concentration, and other index obtained by hemo-gas analysis. However, it has to be considered that the rise in lactate is delayed in comparison to increased O2ER (Donati et al. 2007). CO, assessed by thermodilution or by a less invasive ultrasound technique, is a central factor in evaluation of hemodynamics and therapy, since measures to support cardiac inotropy are critical for a favorable outcome.
In several studies performed in critical care settings such as fluid resuscitation and sepsis, focusing on the safety and effectiveness of goal-directed therapy, blood transfusion were administered according to an established protocol, usually Hb-based. It has been observed that monitoring and therapies based on global oxygenation indexes determine an improvement in clinical outcomes; we underline that this approach appears to entail an increase in blood transfusion rates (Rivers et al. 2001; Trzeciak et al. 2006). Thus, when transfusion therapy is guided by reliable indicators of tissue hypoxia, its beneficial effects appear to be maximized. On the other hand, in septic patients (Mazza et al. 2005; Fuller et al. 2012) as well as in unselected ICU population (Saugel et al. 2013), blood transfusion appears not to affect ScvO2. What seems to emerge from these studies is that blood transfusion affects global indexes of hypoxia only in cases of severe dysfunction, while when RBC are administered on the base of Hb only, they may not exert any improvement and might be not beneficial at all.
Surprisingly enough, global oxygenation indexes have not been widely investigated as drivers for transfusion therapy; only a few published studies focus on transfusion “oxygenation” triggers. Targeting the potential utility of oxygen extraction ratio (O2ER) as an adjunct to the hemoglobin (Hb) concentration for guiding RBC transfusion, it has been observed that, ultimately, ABT rate can be impressively affected, determining either a relevant reduction (by 40–43 %, according to Sehgal et al. 2001; Orlov et al. 2009) or an increase (de Oliveira et al. 2008) or no difference (Donati et al. 2007). An overlook at all the investigational work so far conducted on global oxygenation indexes as drivers for clinical decision about blood transfusion seems to suggest that the direction is correct, but the tools are limited, especially in the perspective of a wide clinical use. Limited results from controlled trials also suggest that application of more complex algorithms including multiple parameters, in the framework of specific clinical situations, may be more informative and useful for monitoring the balance between oxygen supply and tissue demand (Futier et al. 2010).
7.4 New Perspectives
More indicative and practical tools appear to be required to better address questions concerning tissue oxygenation and transfusion need. In this respect, we look with interest at the development of new, or renewed, technologies. Microdialysis, whose application has been widely studied in neurointensive care (Tisdall and Smith 2006), can produce information about multiple indicators, among which particularly valuable is the lactate/pyruvate ratio (L/P) as index of anaerobic metabolism (Waelgaard et al. 2012). It has been found related to the severity of illness in hemorrhagic shock (Ohashi et al. 2009) and sepsis (Dimopoulou et al. 2011) and as predictor of poor outcome (idem; Nikitas et al. 2013). Interestingly, L/P has been found to be significantly affected by blood transfusion (Kopterides et al. 2012) in critically ill patients.
Another interesting noninvasive technique is near-infrared spectroscopy (NIRS) that gives information about the saturation of Hb within the tissues (StO2). Notwithstanding intrinsic limits, it has been suggested as a valuable mean for monitoring the situation of the microcirculation and the effects of therapies in some clinical settings, including non-cardiac surgery (Abdelmalak et al. 2013). In particular, NIRS utilization may result very informative for the evaluation of effects of RBC administration (Creteur et al. 2009; Dani et al. 2010; Yuruk et al. 2012).
Also, orthogonal polarization spectral (OPS) imaging is a noninvasive technique developed to investigate tissue microcirculation and is applied to sublingual district obtaining information about capillary density and blood flow. It has been shown that RBC transfusion has a variable effect in septic patients (Sakr et al. 2007), but in surgical patients, transfusion with fresh RBC causes an increase in the amount of perfused microvessels (Ayhan et al. 2013).
As a practical marker of anemia-induced tissue hypoxia, Hare et al. (2013) propose methemoglobin blood concentration, which may reflect activation of adaptive mechanisms which stimulate NO signaling. Nevertheless, at present, this hypothesis remains to be definitively proven.
In conclusion, in acute normovolemic anemic patients, currently available guidelines indicate RBC transfusion when Hb concentration drops under a determined threshold or in the presence of symptoms that reflect activation of compensatory mechanisms. This strong recommendation is consequent to analysis of results of RCTs that show inferiority, or not superiority, of a liberal transfusion strategy. In particular, in the postoperative management after hip surgery of high-risk patients (actually defined as having any of common cardiovascular risks factors in history), applying a protocol comparing restrictive versus liberal transfusion strategy has not modified the relevant rate of severe outcomes (an overall rate of 7.1 % of death was observed; Carson et al. 2011). Therefore, it appears that other strategies are to be explored in order to improve clinical outcomes in patients belonging to elderly and high-risk populations (i.e., most patients undergoing lower limb arthroplasty). Implementation of monitoring global indexes of tissue hypoxia, possibly by means of noninvasive techniques, should be considered as a future standard of care, even if cost-effectiveness evaluations are still lacking. Doing so, we will be able to restore oxygen delivery to hypoxic tissues, withiin a framework in which the management of every surgery patient, considering his/her centrality, is based on integration of knowledge, communication and formation skiils, also evaluations of organizational constrains. We define this process “Patient Tissue O2 Supply Management.”
Key Points
Hb is chosen as transfusion trigger because so far experimental and clinical researches have not been able to address issues concerning a more “physiological” approach.
Known and unknown comorbidities can be underestimated when considering a single indicator.
Existing results from clinical research are dampened by intrinsic limitations.
Strict implementation of recommendations concerning transfusion thresholds may be difficult to be applied in a clinical routine.
A better blood product may feature a better benefit/cost ratio.
New technologies, applied to noninvasive investigation of tissue oxygenation, may shortly be validated and constitute new standards for appropriateness of blood transfusion.
References
Axler O, Megarbane B, Lentschener C, Fernandez H (2003) Comparison of cardiac output measured with echocardiographic volumes and aortic Doppler methods during mechanical ventilation. Intensive Care Med 29:208PubMed
Boyle MS, Bennett M, Keogh GW, O’Brien M, Flynn G, Collins DW, Bihari D (2014) Central venous oxygen saturation during high-risk general surgical procedures-relationship to complications and clinical outcomes. Anaesth Intensive Care 42(1):28–36PubMed
Carson JL, Terrin ML, Noveck H, Sanders DW, Chaitman BR, Rhoads GG, Nemo G, Dragert K, Beaupre L, Hildebrand K, Macaulay W, Lewis C, Cook DR, Dobbin G, Zakriya KJ, Apple FS, Horney RA, Magaziner J, FOCUS Investigators (2011) Liberal or restrictive transfusion in high-risk patients after hip surgery. N Engl J Med 365(26):2453–2462PubMedCentralPubMedCrossRef

Full access? Get Clinical Tree
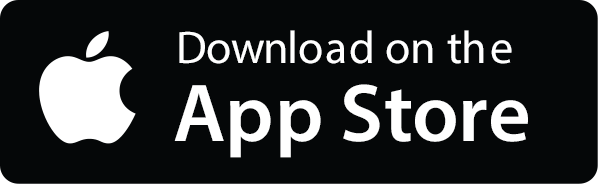
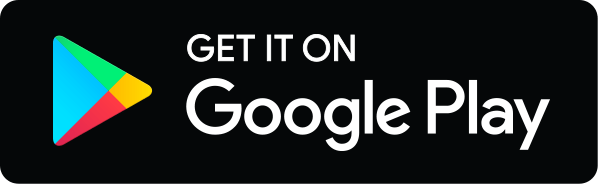