Table 53.2 Effects of Catecholamine Drugs on Adrenergic Receptors
Dobutamine
Dobutamine is a synthetic catecholamine that is classified as an inodilator because it has positive inotropic and vasodilator effects.
Actions
Dobutamine is primarily a β1-receptor agonist, but also has weak β2-receptor agonist activity. The β1-receptor stimulation produces an in-crease in heart rate and stroke volume, while the β2-receptor stimulation produces peripheral vasodilatation (3,4). The stroke volume augmentation produced by dobutamine is shown in Figure 53.1 (4). Because the increase in stroke volume is accompanied by a decrease in systemic vascular resistance, the blood pressure is usually unchanged or slightly increased (3). The response to dobutamine, however, can vary widely in critically ill patients (5).
The cardiac stimulation produced by dobutamine is often accompanied by an increase in cardiac work and myocardial O2 consumption (3). These effects can be deleterious in heart failure because cardiac work and myocardial energy needs are already heightened in the failing myocardium.
Clinical Uses
Dobutamine has been used to augment cardiac output in patients with decompensated heart failure due to systolic dysfunction. However, the unfavorable effects of dobutamine on myocardial energetics has created a preference for other inodilators in decompensated heart failure (see pages 250–251). Dobutamine remains the preferred inotropic agent for the treatment of myocardial depression associated with septic shock (1), but it usually must be combined with a vasoconstrictor agent (e.g., norepinephrine) to raise the blood pressure.
Dosing Regimen
Dobutamine is started at an infusion rate of 3–5 µg/kg/min (without a loading dose), and this can be increased in increments of 3–5 µg/kg/min, if necessary, to achieve the desired effect. (A pulmonary artery catheter is usually needed to guide dobutamine dosing.) The usual dose range is 5–20 µg/kg/min (3), but doses as high as 200 µg/kg/min have been used safely (5). Therapy should be driven by hemodynamic end-points, and not by pre-selected dose rates.
Adverse Effects
Dobutamine produces only mild increases in heart rate (5-15 beats/min) in most patients, but it occasionally causes significant tachycardia (rate increases > 30 beats/min) (3), which can be deleterious in patients with coronary artery disease. Like all positive inotropic agents, dobutamine is contraindicated in patients with hypertrophic cardiomyopathy.
FIGURE 53.1 Stroke volume augmentation produced by equivalent doses of dobutamine and dopamine in post-cardiopulmonary bypass patients. Data from Reference 4.
Dopamine
Dopamine is an endogenous catecholamine that serves as a precursor for norepinephrine. When given as an exogenous drug, dopamine produces a variety of dose-dependent effects, as described next.
Actions
At low infusion rates (″3 µg/kg/min), dopamine selectively activates dopamine-specific receptors in the renal and splanchnic circulations, which increases blood flow in these regions (6). Low-dose dopamine also directly affects renal tubular epithelial cells, causing an increase in both urinary sodium excretion (natriuresis) and urine output that are independent of the changes in renal blood flow (6). The renal effects of low-dose dopamine are minimal or absent in patients with acute renal failure (7).
At moderate infusion rates (3–10 µg/kg/min), dopamine stimulates β-Receptors in the heart and peripheral circulation, producing an increase in myocardial contractility and heart rate, along with peripheral vasodilatation. The increase in stroke volume produced by dopamine is shown in Figure 53.1. Note the greater effect with dopamine compared to dobutamine at equivalent infusion rates.
At high infusion rates (>10 µg/kg/min), dopamine produces a dose-dependent activation of β-Receptors in the systemic and pulmonary circulations, resulting in progressive pulmonary and systemic vasoconstriction. This vasopressor effect increases ventricular afterload, and can reduce the stroke volume augmentation produced by lower doses of dopamine (4).
Clinical Uses
Dopamine can be used to manage patients with cardiogenic shock and septic shock, although other measures are favored in these conditions (i.e., mechanical assist devices are preferred for cardiogenic shock, and norepinephrine is preferred for septic shock). Low-dose dopamine is NOT recommended as a therapy for acute renal failure (see page 640).
Dosing Regimen
Dopamine is usually started at a rate of 3–5 µg/kg/min (without a loading dose), and the infusion rate is increased in increments of 3–5 µg/kg/min to achieve the desired effect. The usual dose range is 3–10 µg/kg/min for increasing cardiac output, and 10–20 µg/kg/min for increasing blood pressure. Dopamine infusions should be delivered into large, central veins, because extravasation of the drug through peripheral veins can produce extensive tissue necrosis.
Adverse Effects
Sinus tachycardia and atrial fibrillation are reported in 25% of patients receiving dopamine infusions (8). Other adverse effects of dopamine include increased intraocular pressure (9), splanchnic hypoperfusion, and delayed gastric emptying, which could predispose to aspiration pneumonia (10).
EXTRAVASATION OF VASOPRESSORS: The risk of tissue necrosis from extra-vasation of dopamine is a concern with all vasopressor (vasoconstrictor) drug infusions, and eliminating this risk is the reason that large, central veins are recommended for all vasopressor drug infusions. If dopamine or any other vasopressor drug escapes from a peripheral vein into the surrounding tissues, the tendency for ischemic tissue necrosis can be reduced by injecting phentolamine (an α-receptor antagonist) into the involved area. The recommended injectate is a solution containing 5–10 mg phentolamine in 15 mL of isotonic saline (6).
Epinephrine
Epinephrine is an endogenous catecholamine that is released by the adrenal medulla in response to physiological stress. It is the most potent natural β-agonist.
Actions
Epinephrine stimulates both α-adrenergic and α-adrenergic receptors (β1 and β2 subtypes), and produces dose-dependent increases in heart rate, stroke volume, and blood pressure (11). Epinephrine is a more potent β1-receptor agonist than dopamine, and produces a greater increase in stroke volume and heart rate than comparable doses of dopamine (12). This is demonstrated in Figure 53.2. The β-Receptor stimulation produces a non-uniform peripheral vasoconstriction, with the most prominent effects in the subcutaneous, renal, and splanchnic circulations. Epinephrine also has several metabolic effects, including lipolysis, increased glycolysis, and increased lactate production (from β-Receptor activation), and hyperglycemia from β-Receptor-mediated inhibition of insulin secretion (11,13).
Clinical Uses
Epinephrine plays an important role in the resuscitation of cardiac arrest (see pages 330–332), and it is the drug of choice for hemodynamic support in anaphylactic shock (see pages 274–276). Epinephrine is also used for hemodynamic support in the early postoperative period following cardiopulmonary bypass surgery (4). Although epinephrine is as effective as other catecholamines in septic shock (12,13), concerns about side effects have limited its popularity in septic shock.
FIGURE 53.2 Cardiac effects of epinephrine (Epi), norepinephrine (Norepi), and dopamine (Dopa) at infusion rates needed to maintain a mean arterial pressure of 75 mm Hg in patients with septic shock. Data from Reference 12.
Dosing Regimen
The dosing regimens for epinephrine in cardiac arrest and anaphylactic shock are presented in Table 14.5 (see page 275). Epinephrine infusions are not preceded by a loading dose. The initial infusion rate is usually 1–2 µg/min (or 0.02 µg/kg/min), and the rate is then increased in increments of 1–2 µg/min to achieve the desired effect (11). The usual dose range for augmenting cardiac output or correcting hypotension is 5–15 µg/min.
Adverse Effects
Epinephrine creates a greater risk of unwanted cardiac stimulation (which can be deleterious in patients with coronary artery disease) than the other catecholamine drugs (11,12). Other adverse effects include hyperglycemia, increased metabolic rate, and splanchnic hypoperfusion (which can damage the mucosal barrier in the bowel) (11–13). Epinephrine infusions are accompanied by an increase in serum lactate levels (11), but this is not an adverse effect because it reflects an increased rate of glycolysis (not tissue hypoxia), and the lactate can be used as an alternative fuel source (see page 187).
Norepinephrine
Norepinephrine is an endogenous catecholamine that normally functions as an excitatory neurotransmitter. When used as an exogenous drug, norepinephrine functions as a vasopressor.
Actions
The principal action of norepinephrine is β-Receptor-mediated peripheral vasoconstriction. However, the adrenergic response to norepinephrine is altered in patients with septic shock (15). For example, norepinephrine infusions are usually accompanied by a decrease in renal blood flow (15), but in patients with septic shock, renal blood flow is increased by norepinephrine infusions (15,16). Similar alterations may also occur with splanchnic blood flow (i.e., normally reduced, but not in septic shock) (15). Norepinephrine is also a weak β1-receptor agonist, but the effects of norepinephrine on stroke volume and heart rate can be comparable to dopamine (a more potent β1-receptor agonist) in patients with septic shock (see Figure 53.2).
Clinical Uses
Norepinephrine is the preferred catecholamine for circulatory support in patients with septic shock. This preference is not based on improved outcomes, because the mortality rate in septic shock is the same regardless of the catecholamine used for circulatory support (1,2,12). Instead, norepinephrine is favored in septic shock because it has fewer adverse effects than dopamine or epinephrine (8,12).
Dosing Regimen
Norepinephrine infusions are usually started at a rate of 8–10 µg/min, and the dose rate is then titrated upward or downward to maintain a mean blood pressure of at least 65 mm Hg. The effective dose rate in septic shock varies widely in individual patients, but is usually below 40 µg/min. Hypotension that is refractory to norepinephrine usually prompts the addition of dopamine or vasopressin, but there is no evidence that this practice improves outcomes.
Adverse Effects
Adverse effects of norepinephrine include local tissue necrosis from drug extravasation, and intense systemic vasoconstriction with organ dysfunction when high dose rates are required. However, whenever high doses of a vasoconstrictor drug are required to correct hypotension, it is difficult to distinguish between adverse drug effects and adverse effects of the circulatory shock.
Phenylephrine
Phenylephrine is a potent vasoconstrictor that has very few applications in the ICU.
Actions
Phenylephrine in a pure β-Receptor agonist that produces widespread vasoconstriction. The consequences of this vasoconstriction can include bradycardia, a decrease in cardiac stroke output (usually in patients with cardiac dysfunction), and hypoperfusion of the kidneys and bowel.
Clinical Uses
The principal use of phenylephrine is for the reversal of severe hypotension produced by spinal anesthesia. However, pure β-Receptor agonists are not universally favored in this situation because they can aggravate the decrease in cardiac stroke output that occurs in spinal shock (17). Phenylephrine is not recommended for hemodynamic support in septic shock, although a clinical study comparing phenylephrine and norepinephrine for the early management of septic shock showed no differences in hemodynamic effects or clinical outcomes with the use of either drug (18).
Dosing Regimen
Phenylephrine can be given as intermittent IV doses. The initial IV dose is 0.2 mg, which can be repeated in increments of 0.1 mg to a maximum dose of 0.5 mg (17). Phenylephrine can be infused at an initial dose rate 0.1–0.2 mg/min, which is progressively decreased after the blood pressure is stabilized (17).
Adverse Effects
The principal adverse effects of phenylephrine are bradycardia, low cardiac output, and renal hypoperfusion. These effects are magnified in hypovolemic patients.
ADJUNCTIVE VASOPRESSORS
The following drugs can be added to vasopressor therapy with catecholamines in selected situations.
Vasopressin
Antidiuretic hormone (ADH) is an osmoregulatory hormone that is also called vasopressin because it produces vasoconstriction.
Actions
The vasoconstrictor effects of vasopressin are mediated by specialized vasopressin (V1) receptors located on vascular smooth muscle. Vasocon-striction is most prominent in skin, skeletal muscle, and splanchnic circulations (19). Exogenous vasopressin does not increase blood pressure in healthy volunteers, but it can produce significant increases in blood pressure in patients with hypotension caused by peripheral vasodilatation (19). This type of hypotension occurs in septic shock, anaphylactic shock, autonomic insufficiency, and the hypotension associated with spinal and general anesthesia.
Other actions of vasopressin include enhanced water reabsorption in the distal renal tubules (mediated by V2 receptors), and stimulation of ACTH release by the anterior pituitary gland (mediated by V3 receptors). These actions are clinically silent when vasopressin is administered in the recommended doses (19).
Clinical Uses
Vasopressin can be used in the following clinical situations.
1. In the resuscitation of cardiac arrest, vasopressin can be given as a single IV dose (40 units) to replace the first or second dose of epinephrine (see page 332).
2. In cases of septic shock that are resistant, or refractory, to hemodynamic support with norepinephrine or dopamine, a vasopressin infusion can be used to raise the blood pressure and reduce the catecholamine requirement (catecholamine sparing effect) (19–20). Unfortunately, there is no survival benefit associated with the this practice (20).
3. In cases of hemorrhage from esophageal or gastric varices, vasopressin infusions can be used to promote splanchnic vasoconstriction and reduce the rate of bleeding.
Dosing Regimen
The plasma half-life of exogenous vasopressin is 5–20 min (17), so vasopressin must be given by continuous infusion to produce prolonged effects. In septic shock, the recommended infusion rate is 0.01–0.04 units/hr, and a rate of 0.03 units/hr is most popular.
Adverse Effects
Adverse effects are uncommon with infusion rates <0.04 units/hr (19). At higher infusion rates, unwanted effects can include consequences of excessive vasoconstriction (e.g., impaired renal and hepatic function), along with excessive water retention and hyponatremia.
Terlipressin
Terlipressin is a vasopressin analogue that has two advantages over vasopressin. First, it is a selective V1 receptor agonist, and does not produce the side effects associated with stimulation of the other vasopressin receptors. Secondly, terlipressin has a much longer duration of action than vasopressin, and a single IV dose of 1–2 mg can raise the blood pressure for 5 hours (19). The long duration of action allows terlipressin to be given by intermittent IV dosing. Terlipressin is a potent splanchnic vasoconstrictor, and may prove valuable in the management of variceal bleeding. However, there is an increased risk of splanchnic ischemia with terlipressin, and ischemic effects cannot be reversed for 5 hours after the drug is administered. Like vasopressin, there is no survival advantage associated with the addition of terlipressin in patients with septic shock (20).
NITROVASODILATORS
Drugs that produce vasodilatation via nitric oxide–mediated relaxation of vascular smooth muscle are known as nitrovasodilators (21). There are two drugs that act in this manner: nitroglycerin and nitroprusside.
Nitroglycerin
Nitroglycerin is an organic nitrate that produces a dose-dependent dilation of arteries and veins (22,23).
Vasodilator Actions
The biochemical basis for the vasodilator actions of nitroglycerin is illustrated in Figure 53.3 Nitroglycerin (glyceryl trinitrate) binds to the surface of endothelial cells and releases inorganic nitrite (NO2), which is converted to nitric oxide (NO) in the endothelial cells. The nitric oxide then moves out of the endothelial cells and into adjacent smooth muscle cells, where it produces muscle relaxation by promoting the formation of cyclic guanosine monophosphate (cGMP).
Venodilatation predominates at lower infusion rates of nitroglycerin (<50 µg/min), while higher infusion rates produce arterial vasodilatation as well. Both of these effects are advantageous in patients with heart failure; i.e., the venodilatation reduces cardiac filling pressures (which reduces edema formation), and the arterial vasodilatation reduces ventricular afterload (which increases cardiac stroke output).
FIGURE 53.3 The biochemical basis for the vasodilator actions of nitroglycerin (NTG) and nitroprusside (NTP). Chemical symbols: nitroglycerin [C3H5O3-(NO2)3], nitroprusside (FeCN5-NO), inorganic nitrite (NO2), nitric oxide (NO), guanosine triphosphate (GTP), cyclic guanosine monophosphate (cGMP).
Antiplatelet Effects
Nitrates inhibit platelet aggregation, and nitric oxide is believed to mediate this effect as well (24). Because platelet thrombi play an important role in the pathogenesis of acute coronary syndromes, the antiplatelet actions of nitroglycerin have been proposed as the mechanism for the antianginal effects of the drug (24). This would explain why nitroglycerin’s ability to relieve ischemic chest pain is not shared by other vasodilator drugs.
Clinical Uses
Nitroglycerin infusions are used to relieve chest pain in patients with un-stable angina (see page 306), and to augment cardiac output in patients with decompensated heart failure (see pages 248–249).
Dosage and Administration
Nitroglycerin binds to soft plastics such as polyvinylchloride (PVC), which is a common constituent of the plastic bags and tubing used for intravenous infusions. As much as 80% of the drug can be lost by adsorption to PVC in standard intravenous infusion systems (22). Nitroglycerin does not bind to glass or hard plastics like polyethylene (PET), so drug loss via adsorption can be eliminated by using glass bottles and PET tubing. Drug manufacturers often provide specialized infusion sets to prevent nitroglycerin loss via adsorption.
DOSING Regimen When nitroglycerin adsorption is not a problem, the initial infusion rate is typically 5–10 µg/min, which can be increased in increments of 5–10 µg/min every 5 minutes until the desired effect is achieved. The effective dose is 5–100 µg/min in most cases, and infusion rates above 200 µg/min are rarely necessary unless nitrate tolerance has developed (see later).
Adverse Effects
The venodilating effects of nitroglycerin can promote hypotension in hypovolemic patients and in patients with acute right heart failure due to right ventricular infarction. In either of these conditions, aggressive volume loading is required prior to initiating a nitroglycerin infusion.
Nitroglycerin-induced increases in cerebral blood flow can lead to in-creased intracranial pressure (25), while increases in pulmonary blood flow can result in increased intrapulmonary shunting and worsening arterial oxygenation in patients with infiltrative lung disease (e.g., pneumonia or ARDS) (26).
METHEMOGLOBINEMIA: Nitroglycerin metabolism generates inorganic nitrites (see Fig. 53.3), which can oxidize the iron moieties in hemoglobin to produce methemoglobin. However, clinically apparent methemoglobinemia is not a common complication of nitroglycerin infusions, and occurs only at very high dose rates (25).
SOLVENT TOXICITY: Nitroglycerin does not readily dissolve in aqueous solutions, and nonpolar solvents such as ethanol and propylene glycol are required to keep the drug in solution. These solvents can accumulate during prolonged infusions. Both ethanol intoxication (27) and propylene glycol toxicity (28) have been reported as a result of nitroglycerin infusions. Propylene glycol toxicity may be more common than suspected because this solvent makes up 30–50% of some nitroglycerin preparations (25). (For a description of propylene glycol toxicity, see page 911–912).
NITRATE TOLERANCE: Tolerance to the vasodilator and antiplatelet actions of nitroglycerin is a well-described phenomenon, and can appear after only 24–48 hours of continuous drug administration (25). The underlying mechanism may be oxidative stress-induced endothelial dysfunction (29). The most effective measure for preventing or reversing nitrate tolerance is a daily drug-free interval of at least 6 hours (25).
Nitroprusside
Nitroprusside is a rapidly-acting vasodilator that is favored for the treatment of hypertensive emergencies. The popularity of this drug is limited by the risk of cyanide intoxication.
Actions
The vasodilator actions of nitroprusside, like those of nitroglycerin, are mediated by nitric oxide (21). The nitroprusside molecule contains one nitrosyl group (NO), which is released as nitric oxide when nitroprusside enters the bloodstream. The nitric oxide somehow ends up in endothelial cells, where it proceeds as shown in Figure 53.3.
Like nitroglycerin, nitroprusside dilates both arteries and veins, but it is less potent than nitroglycerin as a venodilator, and more potent as an arterial vasodilator. Nitroprusside has variable effects on cardiac output in subjects with normal cardiac function (30), but it consistently improves cardiac output in patients with decompensated heart failure (30,31).
Clinical Uses
The principal uses of nitroprusside are the treatment of hypertensive emergencies, where rapid blood pressure reduction is desirable, and management of acute, decompensated heart failure, as described on page 248.
Dosing Regimen
Nitroprusside infusions are started at 0.2 µg/kg/min, and then titrated upward every 5 minutes to the desired result. Control of hypertension usually requires infusion rates of 2–5 µg/kg/min, but infusion rates should be kept below 3 µg/kg/min, if possible, to limit the risk of cyanide intoxication (30). In renal failure, the infusion rate should be kept below 1 µg/kg/min to limit thiocyanate accumulation (described later) (30).
Cyanide Intoxication
Nitroprusside infusions carry a considerable risk of cyanide intoxication. In fact, cyanide accumulation is common during therapeutic infusions of nitroprusside (25,32,33). The origin of the cyanide is the nitroprusside molecule, which is a ferricyanide complex with 5 cyanide molecules bound to an oxidized iron core (see Figure 53.4). This cyanide is released in the bloodstream when nitroprusside breaks up to release nitric oxide and exert its vasodilator actions. The clearance mechanisms for the released cyanide are shown in Figure 53.4. Two chemical reactions help to remove cyanide from the bloodstream. One involves the binding of cyanide to the oxidized iron moiety in methemoglobin. The other reaction involves the transfer of sulfur from a donor molecule (thiosulfate) to cyanide to form a thiocyanate compound, which is then cleared by the kidneys. The latter (transulfuration) reaction is the principal mechanism for removing cyanide from the human body.
FIGURE 53.4 The fate of cyanide (CN) that is released from nitroprusside molecules. See text for explanation.
Healthy adults have enough methemoglobin to bind the cyanide in 18 mg of nitroprusside, and enough thiosulfate to bind the cyanide in 50 mg of nitroprusside (25). This means that healthy adults can detoxify 68 mg of nitroprusside. At a nitroprusside infusion rate of 2 µg/kg/minute (therapeutic dose) in an 80-kg adult, the 68 mg limit of detoxification is reached in 500 minutes (8.3 hours) after the start of the infusion. Thereafter, the cyanide released by nitroprusside will combine with the oxidized iron in cytochrome oxidase and block the utilization of oxygen in the mitochondria.
The capacity for cyanide removal is reduced by thiosulfate depletion, which is common in smokers and postoperative patients (25,32). To eliminate the risk of thiosulfate depletion, thiosulfate can be routinely added to the nitroprusside infusate. About 500 mg of thiosulfate should be added for every 50 mg of nitroprusside (24).
CLINICAL MANIFESTATIONS: One of the early signs of cyanide accumulation is nitroprusside tachyphylaxis (25); i.e., progressively increasing requirements for nitroprusside to maintain the desired blood pressure. Signs of impaired oxygen utilization (i.e., an increase in central venous O2 saturation, and an increase in plasma lactate levels) often do not appear until the late stages of cyanide intoxication (34). As a result, the absence of lactic acidosis during nitroprusside infusion does not exclude the possibility of cyanide accumulation (25,32).
Evidence of possible cyanide intoxication should prompt immediate discontinuation of nitroprusside. Whole blood cyanide levels can be used to confirm the diagnosis of cyanide intoxication, but results are not immediately available, and clinical suspicion is the impetus to begin detoxification measures. These measures are described in Chapter 55.
Thiocyanate Intoxication
The most important mechanism for cyanide removal is the formation of thiocyanate, which is slowly excreted in the urine. When renal function is impaired, thiocyanate can accumulate and produce a toxic syndrome that is distinct from cyanide intoxication. The clinical features of thiocyanate intoxication include anxiety, confusion, pupillary constriction, tinnitus, hallucinations, and generalized seizures (25,32). Thiocyanate can also promote hypothyroidism by blocking thyroidal uptake of iodine (32).
The diagnosis of thiocyanate toxicity is established by the serum thiocyanate level. Normal levels are below 10 mg/L, and clinical toxicity is usually accompanied by levels above 100 mg/L (32). Thiocyanate intoxication can be treated by hemodialysis or peritoneal dialysis.
A FINAL WORD
The Vasopressor Folly
One of the frustrating aspects of critical care practice is the continuing high mortality rates in circulatory shock, particularly septic shock, de-spite correction of the blood pressure with vasopressor drugs. The likely explanation for this is the probability that low blood pressure plays little or no role in the pathogenesis of circulatory shock, or in the clinical outcomes. This is consistent with observations in septic shock indicating that the pathological injury is a defect in oxygen utilization in mitochondria, and the culprit is uncontrolled inflammation, not a low blood pressure. In light of this explanation, the decrease in blood pressure that occurs in shock is more likely to be the result of the pathological cell injury (i.e., shock of the blood vessels) rather than a cause of the injury. Hypotension then becomes one of several consequences of cellular shock, and correcting the hypotension is not expected to correct the primary pathological process. After at least 50 years of focusing on vasopressor therapy in shock, it’s time for a do-over.
REFERENCES
Reviews
1. Holmes CL, Walley KR. Vasoactive drugs for vasodilatory shock in ICU. Curr Opin Crit Care 2009; 15:398–402.
2. Beale RJ, Hollenberg SM, Vincent J-L, Parrillo JE. Vasopressor and inotropic support in septic shock: An evidence-based review. Crit Care Med 2004; 32(Suppl): S455–S465.
Dobutamine
3. Dobutamine hydrochloride. In McEvoy GK, ed. AHFS Drug Information, 2012. Bethesda: American Society of Health-System Pharmacists, 2012:1314–1316.
4. Steen PA, Tinker JH, Pluth JR, et al. Efficacy of dopamine, dobutamine, and epinephrine during emergence from cardiopulmonary bypass in man. Circulation 1978; 57:378–384.
5. Hayes MA, Yau EHS, Timmins AC, et al. Response of critically ill patients to treatment aimed at achieving supranormal oxygen delivery and consumption. Relationship to outcome. Chest 1993; 103:886–895.
Dopamine
6. Dopamine hydrochloride. In McEvoy GK, ed. AHFS Drug Information, 2012. Bethesda: American Society of Health-System Pharmacists, 2012:1314–1316.
7. Kellum JA, Decker JM. Use of dopamine in acute renal failure: A meta-analysis. Crit Care Med 2001; 29:1526–1531.
8. De Backer D, Biston P, Devriendt J, et al. Comparison of dopamine and norepinephrine in the treatment of shock. N Engl J Med 2010; 362:779–789.
9. Brath PC, MacGregor DA, Ford JG, Prielipp RC. Dopamine and intraocular pressure in critically ill patients. Anesthesiology 2000; 93:1398–1400.
10. Johnsom AG. Source of infection in nosocomial pneumonia. Lancet 1993; 341:1368 (Letter).
Epinephrine
11. Epinephrine. In McEvoy GK, ed. AHFS Drug Information, 2012. Bethesda: American Society of Health-System Pharmacists, 2012:1362–1368.
12. De Backer D, Creteur J, Silva E, Vincent J-L. Effects of dopamine, norepinephrine, and epinephrine on the splanchnic circulation in septic shock: Which is best? Crit Care Med 2003; 31:1659–1667.
13. Levy B. Bench-to-bedside review: Is there a place for epinephrine in septic shock? Crit Care 2005; 9:561–565.
Norepinephrine
14. Norepinephrine bitartrate. In: McEvoy GK, ed. AHFS Drug Information, 2012. Bethesda: American Society of Health System Pharmacists, 2012:1371–1374.
15. Bellomo R, Wan L, May C. Vasoactive drugs and acute kidney injury. Crit Care Med 2008; 36(Suppl):S179–S186.
16. Desairs P, Pinaud M, Bugnon D, Tasseau F. Norepinephrine therapy has no deleterious renal effects in human septic shock. Crit Care Med 1989; 17:426–429.
Phenylephrine
17. Phenylephrine hydrochloride. In: McEvoy GK, ed. AHFS Drug Information, 2012. Bethesda,: American Society of Health System Pharmacists, 2012:1306–1311.
18. Morelli A, Ertmer C, Rehberg S, et al. Phenylephrine versus norepinephrine for initial hemodynamic support of patients with septic shock: a randomized, controlled trial. Crit Care 2008; 12:R143.
Vasopressin
19. Treschan TA, Peters J. The vasopressin system: physiology and clinical strategies. Anesthesiology 2006; 105:599–612.
20. Polito A, Parisini E, Ricci Z, et al. Vasopressin for treatment of vasodilatory shock: an ESICM systematic review and meta-analysis. Intensive Care Med 2012; 38:9–19.
Nitroglycerin
21. Anderson TJ, Meredith IT, Ganz P, et al. Nitric oxide and nitrovasodilators: similarities, differences and potential interactions. J Am Coll Cardiol 1994; 24:555–566.
22. Nitroglycerin. In: McEvoy GK, ed. AHFS Drug Information, 2012. Bethesda: American Society of Health System Pharmacists, 2012:1824–1827.
23. Elkayam U. Nitrates in heart failure. Cardiol Clin 1994; 12:73–85.
24. Stamler JS, Loscalzo J. The antiplatelet effects of organic nitrates and related nitroso compounds in vitro and in vivo and their relevance to cardiovascular disorders. J Am Coll Cardiol 1991; 18:1529–1536.
25. Curry SC, Arnold-Cappell P. Nitroprusside, nitroglycerin, and angiotensin- converting enzyme inhibitors. In: Blumer JL, Bond GR, eds. Toxic effects of drugs used in the ICU. Crit Care Clin 1991; 7:555–582.
26. Radermacher P, Santak B, Becker H, Falke KJ. Prostaglandin F1 and nitroglycerin reduce pulmonary capillary pressure but worsen ventilation–perfusion distribution in patients with adult respiratory distress syndrome. Anesthesiology 1989; 70:601–606.
27. Korn SH, Comer JB. Intravenous nitroglycerin and ethanol intoxication. Ann Intern Med 1985; 102:274.
28. Demey HE, Daelemans RA, Verpooten GA, et al. Propylene glycol-induced side effects during intravenous nitroglycerin therapy. Intensive Care Med 1988; 14:221–226.
29. Münzel T, Gori T. Nitrate therapy and nitrate tolerance in patients with coronary artery disease. Curr Opin Pharmacol 2013; 13:251–259.
Nitroprusside
30. Sodium nitroprusside. In: McEvoy GK, ed. AHFS Drug Information, 2012. Bethesda: American Society of Health System Pharmacists, 2012:1811–1814.
31. Guiha NH, Cohn JN, Mikulic E, et al. Treatment of refractory heart failure with infusion of nitroprusside. New Engl J Med 1974; 291:587–592.
32. Hall VA, Guest JM. Sodium nitroprusside-induced cyanide intoxication and prevention with sodium thiosulfate prophylaxis. Am J Crit Care 1992; 2:19–27.
33. Robin ED, McCauley R. Nitroprusside-related cyanide poisoning. Time (long past due) for urgent, effective interventions. Chest 1992; 102:1842–1845.
34. Arieff AI. Is measurement of venous oxygen saturation useful in the diagnosis of cyanide poisoning? Am J Med 1992; 93:582–583.

Full access? Get Clinical Tree
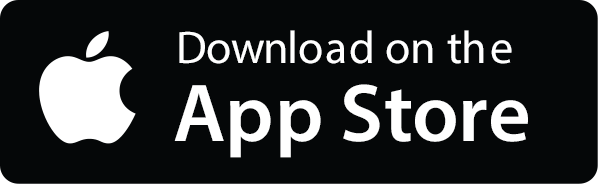
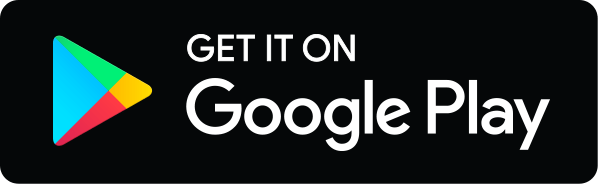