Andres Bacigalupo Landa1, Anthony Zapata2, Stephen A. Stayer1, and Erin A. Gottlieb2 1 Department of Pediatrics and Anesthesiology, Baylor College of Medicine, Arthur S. Keats Division of Pediatric Cardiovascular Anesthesiology, Texas Children’s Hospital, Houston, TX, USA 2 Division of Pediatric Cardiac Anesthesiology, Department of Surgery and Perioperative Care, The University of Texas at Austin, Dell Children’s Medical Center of Central Texas, Associate Professor of Surgery and Perioperative Care Dell Medical School, Austin, TX, USA The commonly reported incidence of congenital heart disease (CHD) in the United States is between 4 and 10 per 1,000 live births. The most common defects are ventricular septal defect, atrial septal defect, valvular pulmonary stenosis, tetralogy of Fallot, coarctation of the aorta, atrioventricular (AV) septal defect and transposition of the great arteries. The National Birth Defects Prevention Network data estimate that there are more than 6,100 cases annually of five defects in the US: truncus arteriosus, transposition of the great arteries, tetralogy of Fallot, AV septal defect, and hypoplastic left heart syndrome (HLHS). Annually, there are an estimated 9,200 patients requiring an invasive procedure related to CHD in the first year of life [1]. With improving survival, the number of unrepaired, repaired, and palliated patients of all ages is increasing. With this increase in the number of patients living with CHD, there is a concomitant increase in the number of patients of all ages presenting for noncardiac procedures requiring anesthetic care. Using data from the Pediatric Health Information System (PHIS), an administrative database that includes the surgical data from 52 freestanding children’s hospitals, Nasr et al. report a significant increase in noncardiac surgical encounters from 38,272 in 2015 to 45,993 in 2019 (p < 0.001) [2]. In fact, also according to PHIS data, 41% of pediatric patients with CHD requiring surgical management of their CHD in the first year of life also have at least one noncardiac procedure before the age of 5 years, most commonly gastrointestinal or otolaryngologic procedures [3]. Adult patients with CHD are also accounting for an increasing percentage of patients presenting for noncardiac surgical procedures and represent a challenge in perioperative management [4]. Data from the Pediatric Perioperative Cardiac Arrest (POCA) Registry report a higher risk of perioperative cardiac arrest in children with CHD undergoing anesthesia compared with the rest of the pediatric population, and 54% of arrests occurred during noncardiac procedures. Mortality after cardiac arrest is also substantially higher for patients with cardiac disease, being 33% among those with heart disease, compared with 23% among those without. It is critical to recognize the patients at highest risk of anesthesia‐related mortality: infants with single‐ventricle lesions and patients with left ventricular outflow tract obstruction (LVOTO), cardiomyopathy or pulmonary hypertension [5, 6]. Risk assessment and stratification has become increasingly important for perioperative planning. The recognition of high‐risk patient groups has led to improved multidisciplinary discussion, selection of appropriate operative venue and recovery area, selection of anesthesia care provider, conduct of anesthesia and surgery, and level of monitoring with the goal of reducing morbidity and mortality. In fact, over the last several years, there has been a reduction in the incidence of mortality in patients undergoing noncardiac surgery at children’s hospitals. This is attributed to improved patient selection, medical optimization, and expertise found at dedicated children’s hospitals [2]. Over the last several years, the literature suggests that an optimized milieu for the perioperative planning and discussion, acquired and shared expertise in caring for these patients, and multidisciplinary collaboration in care has allowed for improved outcomes in this fragile population undergoing noncardiac procedures. It is possible that shared decision‐making allows the group to attempt and be successful in performing procedures on patients that were once deemed too risky. Attempts have been made to identify risk factors to help stratify patients with CHD. The pediatric databases of the American College of Surgeons National Surgical Quality Improvement Program (ACS NSQIP) classifies patients with CHD according to the residual lesion burden and cardiovascular functional status, dividing them into minor, major, or severe CHD (Table 35.1). Using data from the same database, Faraoni et al. found that children with major and severe CHD undergoing noncardiac procedures have an increased risk of mortality and a higher incidence of postoperative reintubation compared with matched controls undergoing comparable procedures. Interestingly, there was no difference between children with minor CHD and their matched controls [7]. Taking this into consideration, Faraoni et al. identified eight predictors of in‐hospital mortality in patients with major and severe CHD and developed a risk stratification score system (Table 35.2). Patients with an overall score of less than or equal to 3 were associated with a low mortality risk (odds ratio [OR]: 1.54, 95% confidence interval [CI]: 0.78–3.04). Those with a score from 4 to 6 had a medium mortality risk (OR: 4.19, 95% CI: 2.56–6.87). Those with a score above 7 had a high mortality risk (OR: 22.15, 95% CI: 15.06–32.59) [8]. A further study by the same group confirmed that the predominant predictors of perioperative mortality in children with CHD were patient comorbidities and the severity of the cardiac lesion at the time of the noncardiac procedure, not the surgical risk [9]. Table 35.1 ACS NSQIP classification of CHD based on residual lesion burden and functional status Source: Faraoni et al. [7]. Reproduced with permission of Elsevier. ACS NSQIP, American College of Surgeons National Surgical Quality Improvement Program; CHD, congenital heart disease. Table 35.2 Multivariate risk stratification score to predict postoperative mortality Source: Faraoni et al. [8]. Reproduced with permission of Wolters Kluwer Health, Inc. Data obtained from multivariable logistic regression and presented as regression coefficient (B), SE, OR, 95% Cl. and Wald test P value. CHD, congenital heart disease; Cl, confidence interval; CPR, cardiopulmonary resuscitation; OR, odds ratio; SE, standard error. Patients with CHD often have other congenital defects and are often cared for by multiple specialists. Each patient most likely has a pediatrician, a cardiologist, and a cardiac surgeon. The patient may be referred to a general surgeon for a gastrostomy tube placement, a urologist for hypospadias repair, a dentist for dental cleaning and restoration, an orthopedic surgeon for treatment of limb deformities, and multiple other practitioners. In addition, the patient may be scheduled for magnetic resonance imaging (MRI) or computed tomography (CT) scanning to evaluate the cardiovascular system or another co‐morbid organ system. Many, if not all, of these procedures require general anesthesia or sedation. The consulted services may not be aware of the patient’s anatomy, physiology, and risk associated with anesthesia. The role of the anesthesiologist in the care of the patient with CHD undergoing noncardiac surgery should not be underestimated. The anesthesiologist may be the member of the collaborative team that understands the most about the surgical procedure, the patient comorbidities, and the anesthetic. A team with ample input from the anesthesiologist should determine where the procedure will take place, such as an outpatient operating room (OR) vs. an OR setup for emergent cardiopulmonary bypass, and how the associated risks can be mitigated. Preoperative management including hydration, medication management, management of anticoagulation, and fasting times should be discussed. In addition, postoperative disposition should be determined in advance, and an intensive care unit (ICU) bed should be reserved if needed. Multidisciplinary planning can also result in combining multiple procedures under the same anesthetic. The patient may require fewer anesthetics and may be at a lower risk of anesthesia‐related morbidity and mortality (see Box 35.1) [10]. The timing of a preoperative cardiology visit prior to a noncardiac procedure depends on the patient’s disease, the stage of repair or palliation, and the degree of limitation of activities of daily living (feeding, growing, neurodevelopment for infants; attending school for older children) due to cardiac disease. The type of noncardiac procedure should not impact these guidelines, as a general anesthetic may be required for any procedure. In general, acyanotic patients who are repaired or who have no limitations due to cardiac disease do not require a preoperative cardiology consultation. If these patients have limitations and are not well compensated, a preoperative consultation is indicated within 6 months of the procedure. For patients with cyanotic CHD who are well compensated and have no limitations due to their cardiac disease, a cardiology visit within 12 months of the procedure is adequate. For patients with cyanotic CHD who have limitations due to their cardiac disease, a preoperative consultation is indicated within 6 months of the procedure. Specific recommendations exist for patients with Williams syndrome (see below). Screening in a pre‐anesthetic clinic setting to gather the results of imaging and other diagnostic studies and to review cardiology records is helpful. If necessary, a preoperative cardiology visit can be scheduled, additional tests, studies and consultations can be scheduled, and all data can be collected and reviewed prior to the patient’s arrival on the day of surgery. This step facilitates the selection of a properly experienced anesthesiologist as well as the proper setting for the anesthetic, and it allows the anesthesiologist to prepare necessary medications and equipment in advance. A plan for the postoperative recovery site for the patient (post‐anesthesia care unit [PACU] vs. ICU) should be made at this time. These preparations translate into fewer cancellations on the day of surgery. Preoperative imaging and hemodynamic studies are addressed extensively in Chapter 18. Important information obtained from imaging studies and cardiac catheterization include right and left ventricular function, presence of valvular stenosis or regurgitation, the size and direction of flow of atrial and ventricular septal defects, patency of shunts or vascular connections, and an estimate of pulmonary vascular resistance (PVR). These key pieces of information help to identify patients with lesions that place them at an increased risk for perioperative morbidity and mortality (e.g. cardiomyopathy, aortic stenosis, pulmonary hypertension) and to construct an anesthetic plan with appropriate hemodynamic goals. Fasting times of 6 hours for solids or formula, 4 hours for breast milk, and 2 hours for clear liquids are the recommended guidelines from the American Society of Anesthesiologists.[11]. For patients with CHD, these fasting times are recommended as well. However, there are some important considerations in this patient population. Patients with LVOTO including hypertrophic obstructive cardiomyopathy, subaortic stenosis, aortic stenosis, and supravalvular aortic stenosis (Williams syndrome or non‐Williams syndrome); systemic‐to‐pulmonary artery shunts (e.g. modified Blalock–Taussig shunt, ductal stent, central shunt); and polycythemic, cyanotic patients have an increased risk of perioperative morbidity and mortality when hypovolemic. Hypovolemic patients with LVOTO may develop significant hypotension during an inhalation induction with a resultant decrease in coronary perfusion pressure that results in unresuscitatable cardiac arrest. Shunted patients with pulmonary blood flow that is completely dependent on systemic blood pressure may become profoundly hypotensive and hypoxemic on induction of anesthesia. Also, when there is decreased blood flow through the shunt, the likelihood of shunt thrombosis increases, as does an unfortunate cycle of hypotension and hypoxemia (Figure 35.1). Other polycythemic, cyanotic patients are at risk for thrombosis and stroke due to increased blood viscosity, which can be due to prolonged fasting. Hypovolemia due to fasting has been reported as a cause of periprocedural morbidity and mortality [12, 13]. Figure 35.1 The cycle of hypotension and hypoxemia resulting in cardiac arrest in shunted single‐ventricle patients. Prevention of hypovolemia is the key to minimizing this risk. Careful planning and communication will minimize the time these patients are without fluids. Families should be advised to give clear fluids liberally until 2 hours prior to the procedure, and if the procedure is delayed, clear fluids can be administered by mouth at the hospital until 2 hours prior to the procedure. In addition, intravenous fluids can be started upon arrival in the hospital to replace losses before induction of anesthesia. Patients may also be admitted the night before surgery to receive intravenous fluids while fasting. These strategies may decrease perioperative risk. Implementation of more liberal guidelines such as allowing clear fluids until one hour prior to the procedure have been shown to be associated with similar aspiration risks as classic NPO guidelines. Liberal guidelines for clears have been adopted in Sweden, Australia, and the United Kingdom with no increase in aspiration. The European Society of Anaesthesiology updated its guidelines as well [14–16]. With this in mind, it may be beneficial to allow patients with a higher hemodynamic risk profile to drink clear fluids until one hour prior to induction to maintain intravascular volume [15, 16]. In general, all chronic cardiac medications should be continued perioperatively. Anticoagulants such as aspirin, warfarin, and heparin may be discontinued at the preference of the surgical team after discussion of a perioperative plan for stopping, transitioning, and restarting anticoagulation with the cardiology team. Another exception may be diuretic therapy. Continuation of diuretic therapy in a fasting patient may not be indicated. However, perioperatively, after volume administration, diuretic treatment may be warranted. Angiotensin‐converting enzyme inhibitors and angiotensin receptor blockers may also be held preoperatively due to a significant incidence of hypotension on induction of anesthesia in the general population [17, 18]. These agents have also been associated with hypotension and hemodynamic instability on induction in pediatric patients with CHD undergoing noncardiac operations [19]. CHD is the leading risk factor for infective endocarditis (IE) in children in the developed world. Lesions associated with increased risk of IE in children are cyanotic CHD, left‐sided lesions, and endocardial cushion defects. In addition, the relative risk of developing IE is higher in the 6 months following cardiac surgery and in children less than 3 years of age [20]. The goal of IE prophylaxis is to provide appropriate antibiotic coverage for procedures that are associated with bacteremia. The three most common bacteria implicated in CHD‐associated IE are Streptococcus (including Streptococcus viridans), Enterococcus, and Staphylococcus species [21]. The 2007 American Heart Association (AHA) guidelines for IE prophylaxis reduced the number of patients with CHD whom they recommend receive IE prophylaxis and reduced the number of procedures for which IE prophylaxis is recommended. The patients with CHD for whom IE prophylaxis is recommended are those who have the highest risk of adverse outcome from endocarditis and these are listed in Box 35.2. Prophylaxis is recommended for patients who are within 6 months of complete repair with prosthetic material or a device. After 6 months, endothelialization of the prosthetic material has occurred, and IE prophylaxis is no longer recommended for these patients. IE prophylaxis is recommended when both patient indications (Box 35.2) and procedure indications occur. For example, IE prophylaxis is recommended for all dental procedures that involve the manipulation of gingival tissue or the periapical region of teeth or perforation of the oral mucosa, but only for patients in Box 35.2. It is also recommended for patients in Box 35.2 who undergo invasive procedures of the respiratory tract that involve incision of the respiratory mucosa, such as tonsillectomy and adenoidectomy or bronchoscopy with biopsy. It is not recommended for bronchoscopy that does not involve incision of the mucosa. The antibiotic regimen should be active against viridans group Streptococcus (see Table 35.3). Antibiotic prophylaxis solely to prevent IE is not recommended for endoscopic gastrointestinal or genitourinary procedures that do not incise mucosa, that is, uncomplicated endoscopies; if biopsies are taken then IE prophylaxis should be considered. However, if the patient is known to be infected or colonized, they should be treated with an antibiotic that covers Enterococcus [22]. IE prophylaxis is also recommended for high‐risk adult patients with CHD undergoing similar procedures. It is important to recognize that IE prophylaxis is also recommended for vaginal delivery and hysterectomy and should be considered for tattoos, and body‐piercings [23–26]. Table 35.3 Infective endocarditis prophylaxis for dental procedures in pediatric patients The number of pediatric patients with cardiovascular implantable electronic devices (CIEDs), pacemakers and defibrillators, has increased as technology has advanced [27]. An increasing number of pediatric patients with these devices will likely present for noncardiac as well as cardiac surgery. It is crucial to manage these devices properly to avoid injury, inappropriate shocking, and hemodynamic instability. A more extensive discussion of pacemaker and defibrillator therapy is presented in Chapters 22 and 34. Preoperatively, it is important to understand the type of device in place, generator location, associated cardiac disease, indications for placement, and the remaining battery life. The underlying cardiac rhythm and degree of pacemaker dependence for cardiac output (none, partial, or complete) are key points of information. This information is best gleaned from a combination of history, physical exam, review of chest radiograph, discussion with or previous note from the cardiologist, and an interrogation of the device in the holding area by a CIED programmer (cardiologist, specialized nurse, or certified company representative from the device manufacturer). Electromagnetic interference (EMI) in the OR is most commonly caused by monopolar electrocautery. EMI can result in inappropriate triggering or inhibition of pacing, reversion to asynchronous mode, inappropriate shock or anti‐tachycardia therapy, and induction of current in the leads, resulting in myocardial damage. Strategies for reducing EMI include the use of bipolar instead of monopolar electrocautery, using short bursts of cutting rather than coagulation current when monopolar is required, and placing the grounding pad for the electrocautery far from the device site. If EMI is likely during a procedure, the CIED programmer should reprogram the pacemaker to an asynchronous mode with a rate greater than the patient’s intrinsic rate. Similarly, the anti‐tachycardia function of a defibrillator should be disabled until the risk of EMI is low. It is critical to monitor the patient for a perfusing rhythm with either a plethysmographic or arterial line waveform, as well as electrocardiogram (ECG), after reprogramming. In addition, temporary pacing/defibrillation equipment must be available at all times, and the availability of appropriately sized equipment must be confirmed. After the procedure when the risk of EMI is low, the device should be interrogated and reprogrammed back to its original baseline settings. It is critical to monitor for a perfusing rhythm and to have pacing/defibrillation equipment present until the device is reprogrammed [28–30]. A magnet can be used in an emergency situation to reprogram a CIED. It is not a substitute for interrogation/reprogramming by a CIED programmer, and it can have unpredictable consequences, particularly with modern multifunction pacemaker/defibrillator devices. For pacemakers, application of the magnet will usually change the pacemaker mode to an asynchronous mode (AOO, VOO or DOO – see Chapter 22) at a fixed rate, usually between 65 and 100 beats/minute (bpm). This fixed rate is device‐specific and dependent on remaining battery life [31]. Untoward effects of magnet application include pacing at a rate or in a rhythm that does not meet the patient’s metabolic or hemodynamic needs. For defibrillators, application of the magnet usually suspends the anti‐tachycardia function, but does not change the pacemaker to an asynchronous mode. If the patient experiences ventricular tachycardia or ventricular fibrillation while the magnet is applied, the magnet should be quickly removed so that the anti‐tachycardia function is restored and the device can deliver a shock [29]. This restoration does not occur with all systems, however; some are disabled until interrogation/reprogramming occurs [32]. There is no substitute for consultation with an expert, assessing the function of the device and converting the functions to an appropriate intraoperative mode. Obviously, there may be emergency situations where this will not be possible. The critical importance of monitoring the patient for a perfusing rhythm throughout the procedure, having backup pacing/defibrillation equipment present, and interrogating and reprogramming the device postoperatively cannot be overemphasized. There is a discussion of MRI‐compatible pacemakers later in the chapter. Heart failure most commonly resulting from CHD, cardiomyopathy, and myocarditis is the diagnosis associated with more than 12,000 annual pediatric hospital admissions in the United States [33]. Most of these patients can be managed medically or surgically, but there are a growing number of pediatric patients that require extracorporeal membrane oxygenation (ECMO) or ventricular assist device (VAD) placement. There are now devices that are appropriate for all sizes of pediatric patients for long‐term support (see Table 35.4) [34, 35]. These devices are discussed in detail in Chapter 37. After placement, cardiac output can be maintained for these patients until cardiac function recovers or a suitable organ becomes available for transplant. In some cases, the patient can remain on VAD support indefinitely, as destination therapy. Destination therapy has been described in patients with end‐stage heart disease due to muscular dystrophy [36]. These patients on long‐term VAD support will likely require anesthesia or sedation for multiple imaging procedures and noncardiac interventions and procedures. In order to provide care for this population of patients, one should become familiar with the different types of devices and the management of hypotension, hypertension, and a decrease in output. A perfusionist or VAD‐trained nurse should be present for all noncardiac procedures to assist with management and transport of the device. In addition, bacterial endocarditis prophylaxis is warranted in all VAD‐supported patients. The Berlin EXCOR (EXCOR Pediatric Ventricular Assist Device, Berlin Heart, GmbH, The Woodlands, Texas, USA) is a pneumatically driven pump that generates a pulsatile pressure with a rate between 30 and 150 bpm depending on the size of the pump chamber and the size and needs of the patient. The transparent pump chamber allows for visual evaluation of filling, emptying, and thrombus formation. Because of the range of pump sizes, it can be used in neonates, infants, and children, and it is the device that is used most commonly for long‐term support in pediatric patients at the present time [35]. Anesthetic management of the patients with a Berlin EXCOR device requires familiarity with the pump and how to troubleshoot hypotension, hypertension, and reduced output. Cave et al. describe a 69% incidence of hypotension in a case series of 29 noncardiac procedures on 11 patients with a Berlin EXCOR device. This group found that hypotension on induction was most associated with the use of remifentanil and was least associated with the use of ketamine as an induction agent. Care should be taken with the administration of anesthetic agents associated with a decrease in systemic vascular resistance (SVR), and intravascular volume and vasoactive agents that increase SVR should be on hand [37]. When caring for a patient with a Berlin EXCOR device, it is critical to maintain the ability to visually inspect the pump. This may require clear drapes, a mirror, and a flashlight. The membrane should be inspected regularly to check for fibrin deposition and for membrane wrinkling. Membrane wrinkling is a sign of incomplete filling of the chamber. Device inspection is a key component of determining the cause of hypotension and the appropriate treatment [34]. Intraoperative emergencies in a patient with a Berlin EXCOR include malignant dysrhythmias, sudden loss of cardiac output, the appearance of air or clot in the pump chamber, and sudden cyanosis. If the patient develops a dysrhythmia, the pump should be examined for filling and output. The right ventricle (RV) can act like a conduit in the short term, and VAD output can be maintained. The patient may require external cardioversion. Loss of cardiac output is most commonly associated with an alarm from the Berlin EXCOR driver unit. It is most commonly caused by a kink in the external tubing of the device. The device should be examined and the tubing unkinked. Appearance of air or clot in the pump chamber can signal an impending embolism. A cardiac surgeon and a perfusionist should be called to the bedside for an urgent pump exchange. Sudden cyanosis in a patient with a Berlin EXCOR device may be a sign of pulmonary embolism with right‐to‐left shunting through an interatrial communication. Transthoracic echocardiography can aid in diagnosis [38]. Table 35.4 Long‐term ventricular assist device options for pediatric patients Source: Data from Mossad et al. [34]. BSA, body surface area. Most left ventricular assist devices (LVADs) are preload dependent and afterload sensitive. Preload dependence means that, in the case of an LVAD, the left side of the heart must receive enough blood to fill the pump. Lack of left ventricular filling can be due to hypovolemia or right ventricular dysfunction. Treatment can include volume administration and support of the RV either with the administration of inotropic agents or decreasing PVR. Increased afterload can lead to inadequate ejection in the Berlin EXCOR or a decrease in VAD flow in a continuous flow device. The troubleshooting of the Berlin EXCOR and continuous flow devices can be found in Figures 35.2 and 35.3, respectively [39, 40]. In the patient with a right ventricular assist device, the afterload is the PVR which must be managed to facilitate flow. VAD use in the failed single ventricle population is increasing, although it is fairly uncommon [41, 42]. In the case of the Fontan, there is no “right heart” that must be supported. In this case, preload is maintained through volume administration and the reduction of PVR using standard measures and pulmonary vasodilators such as nitric oxide or sildenafil. Preoperative evaluation for the patient with a VAD starts with an investigation into the patient’s anatomy and indications for VAD placement. An understanding of renal, hepatic, neurologic function should be sought. The patient may be on a variety of medications including diuretics, ACE inhibitors (or other afterload reducing agents), antiarrhythmics, pulmonary vasodilators, and inotropic agents for right heart dysfunction. The patient will also likely be on antiplatelet agents and anticoagulants. The ECG should be reviewed and the rhythm noted. Many patients on VAD support have CIEDs, which should be managed similarly to other patients in the perioperative period. The cardiologist managing the VAD should be contacted, and the VAD should be interrogated for alarm history including low flow, power elevations, malfunction, and backup battery. A recent echocardiogram should also be reviewed. Information about RV function, PVR, and aortic insufficiency should be sought out [39, 40, 43]. Figure 35.2 Berlin Heart Excor perioperative troubleshooting guide. Target MAPs and flows specific to the patient should be defined preoperatively. iNO, inhaled nitric oxide; PEEP, positive end expiratory pressure; PPV, positive pressure ventilation; PVR, pulmonary vascular resistance; RV, right ventricle; SVR, systemic vascular resistance. (Source: Navaratnam et al. [40]. Reproduced with permission of John Wiley & Sons.) Figure 35.3 Continuous flow devices perioperative troubleshooting guide. Target MAPs and flows specific to the patient should be defined preoperatively. CVP, central venous pressure; LV, left ventricle; MAP, mean arterial pressures; PE, pulmonary embolus; PVR, pulmonary vascular resistance; RPM, revolutions per minute (VAD speed); RV, right ventricle. Source: Navaratnam et al. [40]. Reproduced with permission of John Wiley & Sons.) Monitoring should include standard ASA monitors: ECG, temperature, capnography, blood pressure monitoring, and pulse oximetry. Unfortunately, with continuous flow devices, pulse oximetry and noninvasive blood pressure (NIBP) monitoring can be unreliable. Invasive arterial blood pressure monitoring should be considered for all cases and is necessary for major noncardiac operations. NIRS monitoring can also yield important information about perfusion and cardiac output. Intraoperative transesophageal echocardiography can be used to optimize right heart function and filling [39, 40, 43]. Intraoperatively, the VAD console for a continuous flow device should be in plain sight and monitored. For the Berlin EXCOR, the chamber should be visually inspected to look for thrombus or membrane wrinkles. The management of systemic vascular resistance is critical for optimal VAD functioning. An age‐appropriate MAP should be selected, and optimization of the MAP may include the manipulation of anesthetic depth and the administration of vasopressors and vasodilators. For teenagers and adults, a MAP of 70–80 mmHg should be adequate to maintain good VAD flow and provide an adequate perfusion pressure to end organs. In adults, a MAP greater than 90 mmHg has been shown to be associated with low VAD flow, pump stasis, and embolic stroke [40, 43]. Right heart function may require augmentation with inotropic agents, and PVR may be manipulated to optimize cardiac output. Data from an adult cohort of 246 VAD patients undergoing 702 noncardiac procedures provide some useful information about perioperative complications and monitoring. The most common perioperative complications were intraoperative hypotension (27%) and acute kidney injury (18%). Interestingly, invasive arterial blood pressure monitoring was only used in 20% of the cases. There were also gaps in blood pressure monitoring of greater than 20 minutes in 55% of cases. There were no recorded blood pressures in 5.5% of cases. During these gaps in monitoring, there is potential for unrecognized hypotension [44]. Postoperatively, the patient should be monitored in a special care or ICU to monitor cardiac, respiratory, and neurologic status. Increases in PaCO2 associated with hypoventilation can lead to increases in PVR and suboptimal filling of the pump, as can hypovolemia associated with fluid shifts. Pain can increase both PVR and SVR and should be managed carefully. Patients with VAD support may need to undergo noncardiac surgeries where the intraoperative management for the procedure is not aligned with optimal VAD management. For example, during one‐lung ventilation for a video‐assisted thoracic surgery (VATS), the patient may become hypercarbic and hypoxemia resulting in an increase in PVR, a decrease in right ventricular function, and a decrease device filling. The anesthesiologist should be prepared to take steps to decrease PVR and augment right ventricular function. Transesophageal echocardiography assessing septal configuration, and central venous pressure (CVP) monitoring can facilitate the diagnosis and treatment [45]. Likewise, during neurosurgical procedures, it is important to balance the provision of brain relaxation with the goals for optimal VAD function. In a report of an adult patient undergoing craniotomy for tumor resection, some of the management goals were similar such as the maintenance of a MAP of 70–80 mmHg and hyperventilation. However, mannitol was held due to the effect on preload and the potential for suboptimal VAD function [46]. Of note, the AHA has issued a new statement on resuscitation for the patient with a ventricular assist device. Defibrillation and medication administration per Pediatric Advanced Life Support or Adult Cardiac Life Support Algorithms are indicated, and at this time, external compressions are advised [39, 47]. See Chapter 25 for an extensive discussion of management of cardiac arrest in patients on mechanical support.
CHAPTER 35
Anesthesia for Noncardiac Surgery and Magnetic Resonance Imaging
Introduction
Risk assessment and stratification
Classification
Definition and criteria
Minor CHD
Major CHD
Severe CHD
Variables
B (SE)
OR
95% Cl
P
Risk score
Emergency procedure
0.50 (0.17)
1.66
1.19–2.31
0.003
+1
Severe CHD
0.50 (0.19)
1.65
1.15–2.39
0.007
+1
Single ventricle physiology
0.61 (0.26)
1.83
1.10–3.06
0.020
+1
Surgery within 30 days
0.70 (0.18)
2.01
1.40–2.89
<0.001
+1
Inotropic support
0.72 (0.19)
2.05
1.40–3.01
<0.001
+1
Preoperative CPR
0.90 (0.32)
2.46
1.32–4.57
0.004
+2
Acute or chronic kidney injury
1.48 (0.40)
4.42
2.00–9.75
<0.001
+3
Mechanical ventilation
2.05 (0.18)
7.80
5.42–11.21
<0.001
+4
Preoperative preparation for noncardiac surgery
Multidisciplinary planning
Preoperative cardiology visit
Interpretation of imaging and hemodynamics
Nil per os
Continuation of medications
Endocarditis prophylaxis
Route
Drug and dose
Oral
Amoxicillin 50 mg/kg
Oral – penicillin/ampicillin allergic
Cephalexin 50 mg/kg or clindamycin 20 mg/kg or azithromyicin or clarithromycin 15 mg/kg
Intravenous/intramuscular (IV/IM)
Ampicillin 50 mg/kg or cefazolin 50 mg/kg or ceftriaxone 50 mg/kg
IV/IM – penicillin/ampicillin allergic
Cefazolin 50 mg/kg or ceftriaxone 50 mg/kg or clindamycin 20 mg/kg
Pacemakers and defibrillators
The patient on ventricular assist device support
Device
Positioning
Flow type
BSA (m2)
Ambulation
Discharge home
Berlin EXCOR®
Paracorporeal
Pulsatile
0.2–1.3
Yes
No
Thoratec®
Paracorporeal
Pulsatile
>0.7
Yes
Yes
Heart Mate II®
Intracorporeal
Axial
>1.4
Yes
Yes
HeartWare®
Intracorporeal
Axial
>0.7
Yes
Yes

Full access? Get Clinical Tree
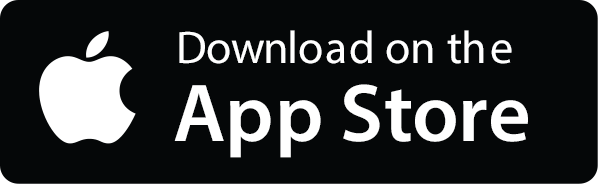
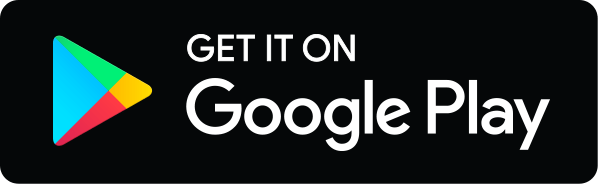