Ian McKenzie1, Maria Markakis Zestos2, Stephen A. Stayer4, Edward Kaminski2, Paul Davies1, and Dean B. Andropoulos3 1 Department of Anaesthesia and Pain Management, The Royal Children’s Hospital, Melbourne, Victoria, Australia 2 Department of Anesthesiology, Children’s Hospital of Michigan, Wayne State University, Detroit, MI, USA 3 Department of Anesthesiology, Perioperative and Pain Medicine, Department of Anesthesiology, Baylor College of Medicine, Texas Children’s Hospital, Houston, TX, USA 4 Department of Pediatrics and Anesthesiology, Baylor College of Medicine, Arthur S. Keats Division of Pediatric Cardiovascular Anesthesiology, Texas Children’s Hospital, Houston, TX, USA The congenital cardiac anesthesiologist is often faced with caring for patients with lesions and diseases that do not fit neatly into the anatomic and surgical classifications presented elsewhere in this book. This chapter presents vascular rings, coronary artery anomalies, pericardial effusion and tamponade, mitral regurgitation (MR), cardiac tumors, aortic aneurysm and aortopathy, and mediastinal masses. The anatomy, incidence, and natural history are reviewed for all lesions, followed by a discussion of pathophysiology. The surgical approach and outcomes are discussed next, and finally, anesthetic considerations and approaches are presented for each lesion. Vascular rings have a reported incidence of between 1 in 1,000 births and 1 in 10,000 births [1, 2]. The abnormalities develop from aberrations of the resorption of the six embryonic aortic arches that connect the primitive dorsal and ventral aorta. Normally, the left part of the fourth arch persists as the aortic arch. The persistence of the right part creates a right arch, while the persistence of both parts of the fourth arch creates a double aortic arch. When these arch abnormalities form a ring, partial in some cases, they may produce either esophageal or tracheal compression or obstruction. Chapter 6 presents an extensive discussion of the embryology of congenital cardiac and vascular defects. The presence of a complete ring would appear to provide an anatomical explanation for obstruction of the encircled structures, but the relationship between symptoms and the ring and whether it is open or closed is complex and varies with the subgroups of lesions. A comprehensive classification of vascular rings is complex, as an extraordinary number of possible combinations of abnormalities are possible. The International Nomenclature and Database Conferences for Pediatric Cardiac Surgery [3] produced a pragmatic summary noting that describing the particular anatomy of the patient and planning for management based on that description (and symptoms) was more important than creating a “classification.” These authors noted that more than 95% of cases associated with symptoms could be described by four categories (Table 31.1) [4]. It is noteworthy that a variant of an otherwise normal left aortic arch is the presence of an aberrant right subclavian arising from the distal arch, which occurs in about 0.5% of normal individuals and is usually asymptomatic. This variant occurs in about a third of babies with trisomy 21. Additional chromosomal variants found in vascular ring patients include 47XXY and 22q11 deletion (DiGeorge syndrome) [5]. Since the increasing use of 3‐vessel tracheal view fetal echocardiography after 2004, prenatal detection rates have increased significantly (Figure 31.1) and although there is insufficient data on whether prenatal diagnosis reduces overall morbidity, it does allow for a prospective care plan in those diagnosed [6] (Table 31.2). Table 31.1 Lurie Children’s Hospital, Chicago, vascular ring experience, 1947–2015 (Source: Backer et al. [4]. Reproduced with permission of Elsevier.) Figure 31.1 Vascular ring prenatal detection rates from 2004–2015 by 3‐year intervals. (Source: Evans et al. [6]. Reproduced with permission of SAGE Publications.) Definitive diagnosis of vascular rings, once clinical suspicion has been aroused, relies on imaging and, in some cases, endoscopy. Historically barium swallow was a key investigation demonstrating the indentation of the ring on the esophagus. Magnetic resonance imaging (MRI), computed tomographic and CT angiography are now the modalities of choice, allowing three‐dimensional (3D) reconstruction of the images and detailed surgical planning [7] (Figure 31.2). CT provides a more precise definition in the tracheobronchial tree and can often be performed without the need for general anesthesia. Greater refinement of operative strategies based on detailed imaging data can lead to improved outcomes for vascular ring patients [8]. Assessment of the fibrous non‐vascular parts of the ring is important. Echocardiography can contribute to the diagnosis but is dependent on achieving adequate echocardiographic windows, which may be difficult and is not suitable for assessing the airway [9]. Bronchography and bronchoscopy may be required to define the functional changes, particularly to assess tracheobronchomalacia and to define the extent of tracheobronchial narrowing associated with a pulmonary artery sling. Double and right aortic arches are the result of the persistence of the fourth right embryonic aortic arch and form complete rings around the esophagus and trachea [1] (Figure 31.3). They comprise less than 1% of congenital cardiac lesions. The double aortic arch group is commonly described according to whether the left or right arch is dominant or whether they are “balanced,” i.e., similar in size. The right aortic arch group may be subclassified by the origin of the left subclavian artery from the right arch. In one‐third of right aortic arches, the right arch is a “mirror image” of the normal left arch with the left subclavian branch of the brachiocephalic first branch of the arch, whereas, in two‐thirds of cases, the left subclavian is the last major branch of the right arch, passing retro‐esophageal to the left upper limb from the right side of the midline. This latter variant may also be associated with dilatation of the origin of the vessel, reflecting the persistence of a segment of fetal aortic arch known as a Kommerell’s diverticulum. If this segment is not dealt with at the time of surgical repair, it can cause persistent or recurrent compressive symptoms. Double or right aortic arches with stenosis or coarctation of the aorta are rare. Patients with vascular rings should be echocardiographically assessed for associated cardiac anomalies that occur in approximately 10% of patients [10]. Table 31.2 Diagnostic data for a 92 patient vascular ring series (Source: Evans et al. [6]. Reproduced with permission of SAGE Publications.) Figure 31.2 Three‐dimensional enhanced computed tomography (CT) reconstruction in an 8‐year‐old patient with right aortic arch, retroesophageal left subclavian artery and Kommerell’s Diverticulum. Note severe esophageal compression (yellow arrow) by the Kommerell diverticulum, causing symptoms of “food getting stuck.” (Source: Backer [8]. Reproduced with permission of Elsevier.) Double and right aortic arches are most commonly present in the first few years of life with respiratory symptoms from tracheal compression. These signs and symptoms include stridor, recurrent respiratory infections, a barking cough, and apnea in infants [4] (Table 31.3). Symptoms of esophageal obstruction are less common, being the primary presentation in about 15% of cases. Esophageal dysfunction may also interact with the respiratory symptoms, because reflux may lead to pulmonary aspiration and episodes of apnea. The development of aspiration pneumonia in the presence of tracheal dysfunction will increase the risk of prolonged pneumonia. The increased work of breathing associated with pneumonia will exaggerate the signs of airway obstruction that may have been absent or subtle when the patient was well. Vascular rings based on double and right aortic arches will encircle the esophagus and trachea. An in utero diagnosis by fetal ultrasound does not correlate with the timing and severity of symptoms, and surgery is not indicated unless the patient has symptoms. Children with mild symptoms often have resolution of those symptoms by 4 years of age. Simply observing children who are asymptomatic or mildly symptomatic regardless of aortic arch anatomy is considered a safe approach [11]. However, earlier surgical repair may limit or prevent the development of tracheomalacia, which is not immediately reversed by surgical relief of the obstruction due to the ring. The long‐term outcome of vascular ring patients is generally very good. Most commonly these are isolated abnormalities, with approximately 10% of patients also having other significant cardiovascular anomalies. A 2014 study by Razon et al. postulated that although prenatal diagnosis has increased the apparent incidence of disease, the age of surgical repair has decreased. This is perhaps because clinicians caring for these patients now may inherently have a higher index of suspicion that new respiratory or gastrointestinal symptoms are related to the vascular ring. Despite the ability to diagnose prenatally, the authors emphasized that a fetal diagnosis should not be the only indication for surgery [5]. Vascular rings are usually repaired through a left thoracotomy. Right thoracotomy or median sternotomy are occasionally used for unusual variants, with median sternotomy being most common when there are other cardiac lesions needing repair. The principle of the surgery is to ensure that the ring is completely divided, which may require division of the ligamentum arteriosum as well as a vascular structure or a fibrous remnant of a vascular structure [1, 12] (Figures 31.4 and 31.5). If a vascular structure is divided, such as one side of a double aortic arch, it is critical to ensure flow to all parts of the body is maintained. Test clamping and assessment of perfusion to the limbs and head may be required. Some variations of anatomy require reimplantation of blood supply. Such is the case with an aberrant origin of a left subclavian artery from a right aortic arch with a Kommerell’s diverticulum – a swelling at the insertion of the left subclavian. The Kommerell’s diverticulum can be resected and the left subclavian artery re‐implantated onto the left common carotid artery [13] (Figure 31.6). The primary excision of the Kommerell diverticulum is currently the preferred approach since it is now known that its natural history is to steadily enlarge over time [8]. The outcome after surgical repair of vascular rings related to right or double aortic arches is very good with almost no mortality. Relief of obstruction is usually significant. Tracheomalacia may continue to produce postoperative symptoms, but these diminish with the growth of the patient and tissue remodeling [14, 15]. Video‐assisted thoracoscopic techniques may be used for these procedures and will influence anesthetic management [16]. Figure 31.3 Embryonic aortic arches and double aortic arch. (A) Diagram of the embryonic aortic arches. Six pairs of aortic arches originally develop between the dorsal and ventral aorta. The first, second, and fifth arches regress. Preservation or deletion of different segments of the rudimentary arches results in a double aortic arch (B), a right aortic arch (C), or the “normal” left aortic arch (D). Ao, aorta; CCA, common carotid artery; L, left; PA, pulmonary artery; R, right; SA, subclavian artery. Backer & Mavroudis [1]. Reproduced with permission of John Wiley & Sons. The preoperative assessment should consider the impact that the vascular abnormality has had on the patient, the details of the anatomy and function of the components of the vascular abnormality, the surgical plan, and the requirements for postoperative care. The severity of preoperative obstruction and chronic lung damage from recurrent infections is relevant to the risk of difficulty with oxygenation and ventilation during surgery and in the postoperative period. Chest radiograph findings suggesting chronic lung disease, tachypnea at rest, and rapid desaturation with crying are important indicators of associated lung disease. All other imaging studies, including CT scan, echocardiography, barium swallow, and MRI, should be thoroughly reviewed. The medical and surgical team should decide on the optimal timing of surgical intervention, e.g., can the lung function be further optimized prior to surgery vs. the risk of delaying definitive therapy? The anesthesiologist and surgeon must have a clear plan regarding the need for lung isolation and discuss monitoring requirements if temporary or permanent occlusions of blood vessels are part of the surgical plan. This will influence whether intraoperative access to limbs for circulatory assessment by clinical means, pulse oximetry, intra‐arterial pressure monitoring, transcranial Doppler or near‐infrared spectroscopy (NIRS) will be required and whether a particular limb is most appropriate for arterial pressure monitoring (not at risk of being lost due to temporary clamping during the procedure). Table 31.3 Symptoms leading to clinical presentation in patients with vascular ringsa (Source: Backer et al. [4]. Reproduced with permission of Elsevier.) Reproduced with permission from Backer et al5. a More than one symptom occurred in many patients. b Our records did not provide symptoms for the earlier patients in the series. Intra‐arterial pressure monitoring is usually warranted due to the risk of massive hemorrhage, the need for significant intrathoracic retraction during the procedure, and, in some cases, the need to confirm regional perfusion during intraoperative clamping of intrathoracic vessels. Central venous catheterization is usually done for these cases, especially if aortic clamping and suture lines will be required, as pharmacologic control of blood pressure is more safely performed with central venous access. Substantial peripheral venous access should be secured in anticipation of the possible need for rapid resuscitation in the face of surgical blood loss. The procedures usually are associated with minimal blood loss, but preparation should be made for the possibility of massive transfusion. The tracheal narrowing from vascular rings will not usually prevent an endotracheal tube from being passed through the narrowing. It is rare that positive pressure ventilation and continuous positive airway pressure (CPAP) or positive end‐expiratory pressure (PEEP) will not be sufficient to allow ventilation during the induction of anesthesia. A single‐lumen endotracheal tube is usually adequate for open thoracotomy and often acceptable for thoracoscopic procedures where lung retraction provides good surgical access. If desired, lung isolation may be achieved (see Chapter 23 for a discussion of lung isolation techniques). Rigid or flexible bronchoscopy may be performed pre‐ and post‐procedure in some cases. Most patients will tolerate early extubation if appropriate analgesia is provided. This will vary with the institution and whether an open or thoracoscopic technique was used. Thoracic epidural analgesia is used in some institutions for thoracotomy for vascular rings. Significant preoperative lung disease or predicted postoperative airway obstruction may require more prolonged intubation and respiratory support. Innominate artery compression of the trachea is not a true vascular ring [1] (Figure 31.7). Some degree of innominate artery indentation is normal in the first years of life, and the origin of the vessel from the aorta to the left of the trachea is also normal in young children. The normal changes in geometry of this region with growth cause the origin of the innominate artery from the aorta to move to the right of the trachea (not crossing it) and to rotate to the cranial side of the aorta. These changes decrease the degree of tracheal compression caused by the innominate artery as a child grows. Tracheal compression is more likely if the origin of the innominate artery from the aorta is more distal (to the left) and posterior than usual, resulting in anterior compression of the trachea as the vessel passes toward the right side across the front of the trachea. Severity of symptoms and efficacy of medical therapy for acute exacerbations associated with respiratory infection will influence the decision about whether to offer surgery or continue observation and medical therapy, knowing that the natural history of the condition is to improve with growth. Some other congenital abnormalities of this region, including congenital heart disease (CHD), and some patients with repaired esophageal atresia and tracheoesophageal fistula may cause “crowding” of the area, making innominate artery compression of the trachea more likely and perhaps less likely to resolve with time. Dynamic multidetector CT scan (MDCT) is a technique that can provide 2D and 3D reconstructions of thoracic structures. End inspiration and end‐expiration images can be used to compare airway patency and can potentially diagnose other anomalies [17]. If extrinsic compression is suspected or identified, MDCT can be vital for surgical planning. For patients with symptomatic tracheobronchial malacia (TBM) the Esophageal and Airway Treatment (EAT) Center at Boston Children’s Hospital has found that the dynamic 3‐phase bronchoscopy has great diagnostic utility. The first phase occurs while the patient is shallow breathing to reveal resting airway compression, secretion accumulation, and vocal cord motion. The second phase is to induce coughing and Valsalva while observing for maximum dynamic collapse in the entire airway. The third phase is to distend the airway with 30–60 cm H20 pressure in order to reveal lesions that may not have been previously recognized such as fistulas, diverticuli, and aberrant bronchi. Careful documentation of the area of tracheal involvement can also aid in the correct classification and subsequent response to treatment [17]. Figure 31.4 Double aortic arch division. (A) Double aortic arch, right arch dominant. (B) Dividing left aortic arch. (C) Arch divided. Ao, aorta; CA, carotid artery; L, left; LCCA, RCCA, left, right carotid artery; PA, pulmonary artery; R, right; SA, subclavian artery. (Source: Backer & Mavroudis [1]. Reproduced with permission of John Wiley & Sons.) Symptomatic innominate artery compression syndrome produces a localized narrowing of the trachea, often with a localized segment of tracheomalacia. Symptoms typically do not appear until there is more than 50% obstruction of the trachea. The condition presents with stridor and airway obstruction, often with an abnormal harsh cough relating to the associated tracheomalacia. Innominate artery compression of the trachea may underlie some presentations of recurrent croup. Because there is some degree of tracheal impression from the innominate artery in normal young children, it is important not to overdiagnose this condition with the risk of significant surgical intervention offered inappropriately. Surgery for this condition is not commonly indicated except for significantly symptomatic patients where conservative therapy is deemed inappropriate. Most of the procedures are done through a left thoracotomy, but sternotomy and right thoracotomy have been used for vascular suspension procedures. Surgery can involve aortopexy of the innominate artery and aorta anterior to the sternum, which has a modest risk of recurrence, or reimplantation of the innominate artery so that its origin from the aorta is to the right of the trachea and from the cephalad aspect of the aorta [1] (Figure 31.8). This is a more complex procedure with a greater risk of complications, but it definitively eliminates the innominate artery as a cause of tracheal compression. Anterior and/or posterior tracheopexy have been described as a novel management technique for TBM when aortopexy alone was unable to address tracheal cartilage abnormalities or membranous luminal intrusion. Anterior tracheopexy anchors the trachea to the sternum similar to aortopexy and posterior anchors the trachea to the anterior longitudinal spinal ligament. Both techniques are done with direct bronchoscopic guidance to ensure the sutures remain extraluminal and avoid excess tension in the trachea [17].either procedure, residual tracheomalacia may cause ongoing symptoms. Figure 31.5 Right aortic arch – division and oversewing (B) of ligamentum arteriosum (A). Ao, aorta; CCA, common carotid artery; L, left; MPA, main pulmonary artery; R, right; SA, subclavian artery.) (Source: Backer & Mavroudis [1]. Reproduced with permission of John Wiley & Sons.) The preoperative general assessment is similar to that described for vascular rings. The conduct of anesthesia is simplified if aortopexy of the innominate/aorta is the surgical technique because the risk of major intraoperative complications or the need for vascular clamping is minimal. Reimplantation of the innominate artery will involve more specific and intense intraoperative monitoring, which will include consideration of the aortic surgery and temporary occlusion of the innominate artery. For continuous arterial pressure monitoring, an arterial line can be placed in the left radial artery. Information about the adequacy of collateral perfusion during clamping of the innominate can be gained by placing an arterial line in the right radial artery. Previous case series have not noted stroke as a significant risk during temporary clamping of the innominate artery, but monitoring of cerebral perfusion, e.g., with NIRS, may be warranted to confirm minimal risk of ischemic stroke due to poor perfusion relating to clamping. Anticoagulation (normally 100 units/kg heparin; target activated clotting time 180–200 seconds) is used to minimize the risk of embolus or thrombosis related to innominate artery reimplantation. Bronchoscopy, either rigid or flexible, is frequently performed pre‐ and post‐procedure to assess results of surgery or in the case of tracheopexy may actually be needed to guide the surgical procedure. Postoperative care will be predicated on similar principles to vascular ring procedures. Pulmonary artery sling results from an aberrant course of the left pulmonary artery around the trachea, which may restrict the space in which the trachea forms [1] (Figure 31.9). The left pulmonary artery arises from the posterior surface of the right pulmonary artery and passes over the superior surface of the right main bronchus then posterior to the trachea and anterior to the esophagus to the left lung. The most important factor that determines prognosis is the association of this lesion with segmental narrowing of the trachea, and sometimes bronchi, with complete cartilaginous rings in the affected segment, the so‐called “rat‐tail trachea.” A long segment of narrowing worsens the prognosis. Curiously, these patients frequently do not present for some months after birth, often with airway obstruction associated with an apparently minor respiratory infection. Recent advances in tracheal reconstruction have been associated with an improved outcome. Imaging studies are crucial for accurate diagnosis. Echocardiography may provide initial diagnosis, but anatomic detail is better provided by CT or MRI; cardiac catheterization is rarely indicated for this problem. Figure 31.6 Resection of Kommerell’s diverticulum. (A) Typical anatomy of a patient with a right aortic arch, retroesophageal left subclavian artery, and large Kommerell’s diverticulum. LCA/RCA, left/right carotid artery; LSA/RSA, left/right subclavian artery. (B) There is a vascular clamp partially occluding the descending thoracic aorta at the origin of the Kommerell’s diverticulum, which has been completely resected. (C) The completed repair. The orifice where the Kommerell’s diverticulum was resected is usually closed primarily or patched if necessary. The left subclavian artery has been implanted into the side of the left common carotid artery. (Source: Backer et al. [13]. Reproduced with permission of Elsevier.) Figure 31.7 Innominate artery compression of anterior trachea. Ao, aorta; PA, pulmonary artery. (Source: Backer & Mavroudis [1]. Reproduced with permission of John Wiley & Sons.) Figure 31.8 Innominate artery suspension. Exposure is through a right submammary thoracotomy. Fixing the adventitia of the innominate artery to the posterior table of the sternum with pledgeted sutures pulls the innominate artery anteriorly and actively pulls the tracheal wall forward and opens it. Asc Ao, ascending aorta. (Source: Backer & Mavroudis [1]. Reproduced with permission of John Wiley & Sons.) Figure 31.9 Pulmonary artery sling. L, left; M, main; PA, pulmonary artery; R, right. Inset: left lateral view of anterior compression of the esophagus and posterior compression of the trachea. (Source: Backer & Mavroudis [1]. Reproduced with permission of John Wiley & Sons.) Pulmonary artery sling pathophysiology is focused on the severity and length of the associated tracheobronchial narrowing. It is not clear whether the usual delay in presentation until some months of life represents primarily a failure of further growth of airway diameter or an increase in ventilatory requirements as the child grows. A common clinical scenario is an apparently minor respiratory infection in the middle of the first year of life, leading to obvious signs of increased work of breathing relating to respiratory obstruction with slow or incomplete resolution of the illness. Distinguishing this from the far more common bronchiolitis and laryngotracheobronchitis infections in children with underlying normal airways is difficult at the primary presentation. The severity of obstruction, failure of resolution, and recurrent presentation are the usual triggers for further investigation and diagnosis of the patient with pulmonary artery sling. Huang and colleagues recommend tracheoplasty surgery if the tracheal width is less than 3 mm [18]. An Australian retrospective study of 33 PA sling patients by Yong and colleagues analyzing cases showed that long‐term outcomes and survival are often worsened by the need for tracheal surgery [19] (Figure 31.10). Early mortality was clearly increased in those requiring surgery on the trachea. A review of pulmonary function testing several years after tracheal surgery demonstrated that a non‐reversable obstructive pattern was often demonstrated on pulmonary function testing suggesting the need for long‐term respiratory assessment of these patients. The long‐term outcome of the surgical intervention for these patients depends on the tracheal reconstruction. The surgery is done through a sternotomy, on cardiopulmonary bypass (CPB). The aberrant left pulmonary artery can be dealt with at the time of reconstruction of the trachea, most commonly by dividing and reimplanting it, to mimic the normal takeoff from the main pulmonary artery, but alternative methods to relocate the vessel in relation to the reconstructed trachea have been used. Figure 31.10 Kaplan‐Meier curve showing probability of survival in (A) all patients who had pulmonary artery (PA) sling repair and (B) patients who had PA sling repair only compared to patients who had both PA sling and tracheal repair. CI, confidenc interval. (Source: ReproducedYong et al. [19]. Reproduced with permission of Springer Nature.) For patients with a short segment of tracheal stenosis, resection of the stenosis, mobilization of the trachea, and direct anastomosis may be sufficient and relatively straightforward surgery [1] (Figure 31.11). For patients with a longer segment of narrow trachea with complete rings, the long‐term problem with tracheal reconstruction has been to achieve both continuing adequate airway patency and a functional tracheal epithelium. The airway patency must be throughout the respiratory cycle, which requires a degree of rigidity to resist collapse in expiration. For long segment reconstruction, the slide tracheoplasty has become the preferred surgical approach. Tracheal surgery is technically demanding and should ideally be performed in centers that do large numbers of cases [20]. Alternatives, such as simple patches (e.g., with pericardium), will usually be associated with tracheomalacia in the long term. Scarring and granulation formation and the lack of cilial function will often complicate stented patches, whether stented by external, surgically placed cartilage grafts or internal stents, similar to those used for vascular stenting. There is currently no data to support stenting as a primary approach for tracheal stenosis patients but they are often used as a bridge to surgical therapy [20]. The most commonly cited complications of currently available intraluminal stents are stent migration, degradation and fragmentation [21]. A systematic review of 26 studies of intraluminal stents showed an overall complication rate of 21.7% (8.7% stent fragment foreign body and 4.3% each for stent migration, local stenosis, and granulation tissue) A more complex style of self‐expanding helical stent is under preclinical investigation. It is made of nitinol, a nickel‐titanium alloy, designed specifically to minimize foreign‐body reaction, preserve mucociliary transport, and facilitate atraumatic removal [22, 23]. External custom 3‐D printed bio‐reabsorbable tracheal splints are also under clinical investigation [18], and may allow time for tracheal growth but require an more complex open surgical procedure [18, 24]. Alternative sources of tissue, such as laboratory‐based tissue engineering or donor tissue, are possible, but slide tracheoplasty has the potential to provide airway patency, with a non‐collapsing wall and normal functioning respiratory epithelial mucosa. Figure 31.11 Illustration of operative technique for pulmonary artery sling repair with tracheal resection (performed with cardiopulmonary bypass). (A) Left pulmonary artery (LPA) encircles and compresses the distal area of the trachea and right main‐stem bronchus. (B) Relationship of the LPA to the trachea and esophagus. (C) Repair is by transecting the LPA at its origin from the right pulmonary artery (RPA) and anastomosing the LPA to the main pulmonary artery (MPA) at a site that approximates the usual anatomic configuration. The tracheal stenosis has also been resected, and an end‐to‐end tracheal anastomosis is shown. (D) Postoperative relationship of neo‐LPA to MPA anastomosis showing the relatively long distance from the original LPA takeoff from the RPA. (Source: Backer & Mavroudis [1]. Reproduced with permission of John Wiley & Sons.) Slide tracheoplasty is a solution for many longer segment narrowings which optimizes the use of native tissues to reconstruct the trachea and potentially proximal bronchi [25] (Figure 31.12). The technique involves an oblique division of the trachea, with the distal and proximal ends of the incision continued on the proximal trachea and distally, and potentially along a bronchus if there is bronchial involvement. The trachea is mobilized so that the oblique end of the proximal trachea can slide into the apex of the distal incision, and the oblique end of the distal trachea can slide into the apex of the proximal incision. This achieves expansion of the lumen, with essentially normal tracheal tissue and mucosa forming the reconstructed trachea. The long oblique anastomosis is less likely to stricture than a circular anastomosis. The slide maneuver shortens the trachea. Independent of symptom severity, DLR (diameter/length ratio) is defined as the ratio of the narrowest diameter divided by the stenotic segment length. DLR has been reported by Hong and colleagues as a potentially reliable indication for surgery in patients with congenital tracheal stenosis with pulmonary artery sling [26]. According to Harada and colleagues, a DLR of less than 5.9 may be a reliable indicator for slide tracheoplasty in addition to vascular repair [27]. Patients with a long segment of tracheobronchial narrowing in association with a pulmonary artery sling may well benefit from a multidisciplinary assessment. These patients should be managed at an appropriate referral center. According to an analysis by Chen and colleagues of 23 children between 2003 and 2018, slide tracheoplasty can be performed for up to two thirds of the length of the trachea. Beyond this length, tension‐relieving maneuvers may be required to prevent complications such as anastomotic breakdown, recurrent granulation tissue, and a narrowed lumen [28]. It is desirable that cardiac surgery, cardiology, thoracic and otolaryngological surgery, anesthesia, pulmonary medicine, intensive care, and medical imaging and intervention should all participate and collaborate in planning the management of these patients. The anesthesia assessment of these patients is particularly focused on the impact and extent of the associated tracheal stenosis. The indications and timing of surgery are entirely driven by symptoms related to the tracheal obstruction. The spectrum of these patients’ conditions may well range from a minimally symptomatic baby who is requiring no current intervention to a patient in a perilous situation with maximum intensive care support, with complex ventilator requirements and potentially requiring extracorporeal oxygenation or carbon dioxide clearance. Positive pressure ventilation in these patients is difficult to optimize and assessing flow volume loops created by different ventilator strategies can be helpful. Common strategies include permissive hypercapnea, attention to adequate expiratory time to minimize hyperinflation which limits ventilation, and continuous positive pressure to maintain airway patency. Anesthesia is similar to other procedures requiring CPB. Passing an endotracheal tube through the larynx is initially as for a normal patient. The difference is that a tube that is appropriate for the upper trachea will not pass through the narrow segment. No attempt should be made to advance the endotracheal tube through the narrowing. Bronchoscopic assistance may be utilized or the tube can be gently advanced through the larynx until resistance is felt and minimally withdrawn from that point. The narrow segment of the trachea can easily be blocked by blood, swelling, or secretions associated with trauma to the mucosa, with potentially catastrophic results. Avoiding these complications by minimizing airway trauma is a primary aim. If required, suctioning may require a fine catheter and lubrication to clear secretions from the narrow segment. Suctioning may be necessary for adequate ventilation but runs the risk of traumatizing the mucosa. Suctioning may be life‐saving if secretions or blood occlude the narrow segment. High inspiratory pressures may be required to inflate the lungs, as the narrow segment may have a very high resistance to flow. For this reason, consideration should be given to ensuring that the largest appropriate tube is placed above the narrowing to minimize the risk of an excessive leak at high ventilator pressures or inability to generate adequate pressure. If there is sufficient length of trachea above the narrowing, a tube with a cuff, particularly one with the minimum distance between the cuff and the tip of the endotracheal tube, may be appropriate. Bronchoscopic confirmation and guidance of placement should be considered. The mobilization and slide maneuver associated with slide tracheoplasty will tend to draw the trachea down into the chest, meaning that the distance between the larynx and the tip of the endotracheal tube placed at the beginning of the procedure will decrease. This should be assessed, and the endotracheal tube confirmed to be in a good position before transporting the patient to the intensive care unit. With adequate surgical relief of tracheal obstruction and air‐tight closure of the tracheal (or tracheobronchial) anastomosis, the patient should tolerate positive pressure ventilation postoperatively and the endotracheal tube can be left above the anastomosis. Early extubation is usually avoided, as postoperative swelling over the next few days may still be associated with a restricted airway. Fiberoptic bronchoscopic examination via the endotracheal tube can guide decision‐making about airway management, as well as provide controlled airway suctioning and assist with deciding the best position for the endotracheal tube during the postoperative course. Although a relatively early return to spontaneous ventilation may minimize the need for positive pressure ventilation, decreasing the risk of air leak, coughing or straining on the endotracheal tube, perhaps related to decreased sedation to allow spontaneous ventilation, could be associated with a greater risk of air leak. The management plan will require repeated assessment if clinical circumstances change. Figure 31.12 Slide tracheoplasty. (A) The trachea is transected in the midportion of the tracheal stenosis. This site is determined by either external examination or internal bronchoscopic findings. The inferior portion of the trachea is incised anteriorly and the superior portion of the trachea is incised posteriorly. (B) The ends of the trachea are beveled as shown in the small inset. The suture line is started superiorly (parachute technique) and finished inferiorly just above the carina. (C) Completed slide tracheoplasty. (Source: Backer et al. [12]. Reproduced with permission of Elsevier.) Box 31.1 Summarizes the surgical approach to vascular rings and slings [1]. There is a wide anatomic variation of the coronary arteries. There are a number of common variants which are accepted as normal but which may have significance if the disease of the coronaries develops (e.g., proximal atheroma in a single coronary artery which gives rise to left and right coronary arteries) or surgery of the region is required (where intramural or unusually placed vessels may be more likely to be injured). The physiological function of the coronary arteries and the heart is usually normal in these variations. The focus of this section is congenital anomalies of the coronary arteries. Acquired abnormalities are also important in childhood. These include infective causes, of which Kawasaki disease is the most prominent, iatrogenic causes arising from surgery or invasive cardiological procedures, and the development of abnormalities in relation to other lesions, such as the coronary perfusion issues that can be associated with supravalvular aortic stenosis. The Congenital Heart Surgery Nomenclature and Database Project provided an excellent classification and review of the significance of anomalies of the coronary arteries [29]. The major types of coronary anomalies are divided into the following seven groups: Coronary anomalies are a diverse group of disorders with varying clinical manifestations for which history and physical exam are extremely unreliable in diagnosing these rare conditions which rarely cause symptoms at rest. They are the second most common cause of sudden cardiac death in young competitive athletes [30]. A similarly high incidence of coronary anomalies has also been seen at autopsy of young military recruits who died suddenly [31]. A 2018 MRI screening study by Angelini and colleagues of a general population of 5,000 adolescents participating in school sports revealed a 1.3% incidence (70 cases) of high‐risk cardiovascular disease including 23 study members with AOCOS anomalies (anomalous origin of a coronary artery from the opposite sinus) [32] (Table 31.4). Left sided AOCOS with an intramural course (L‐AOCOS‐IM) constitutes the most serious group of all LCA anomalies [34]. During strenuous exercise the proximal intramural course predisposes to lateral compression and phasic narrowing during systole potentially leading to ischemia. An intra‐arterial course between the great vessels has also been found to be problematic and is often difficult to confirm with imaging [35]. Screening for these abnormalities in children has become a recent topic of focus but no clear guidelines have yet been established. Supravalvular aortic stenosis is also clinically important and associated with sudden death, sometimes in association with apparently stable anesthesia, and the complex mechanisms, which may be both structural and dynamic. The development of abnormalities of coronary perfusion in this condition is discussed. Table 31.4 Prevalence of potentially high‐risk cardiovascular conditions (Source: Angelini [32]. Reproduced with permission of Springer Nature.) ACAOS, anomalous origin of the right or left coronary artery from an ectopic coronary artery with intramural course; CI, confidence interval; CMP, cardiomyopathy; CVC, cardiovascular condition; DCM, dilated cardiomyopathy; ECG, electrocardiogram; HCM, hypertrophic cardiomyopathy; HO, high origin; hr high risk; L‐ACAOS‐IM, left ACAOS from the right sinus (RSV) with an intramural course; NC, non‐coronary sinus; NCLV, non‐compaction left ventricle; R‐ACAOS, right ACAOS; WPW, Wolff‐Parkinson‐White. NCLV is currently not considered a high‐risk cardiovascular condition but is reported here because of the potential need for a new discussion of the entity, still under study. These are rare lesions with the commonest and most important being anomalous left coronary artery arising from the pulmonary artery (ALCAPA) with an incidence of only 1 in 300,000 live births. ALCAPA presents early in life with a mortality of up to 90% in the first year, if untreated. Anomalous right coronary artery from the pulmonary artery (ARCAPA) is even rare, usually presenting after a few years of life, but with a wide clinical spectrum. Later presentations are not infrequent and some adults with this condition are at risk of sudden death [36–38]. Common presenting findings in the adolescent or adult population include EKG changes (ST changes and Q waves), cardiomegaly, a pansystolic murmur, and echocardiographic findings of mitral regurgitation [39]. Extensive intercoronary collaterals from the right side of the heart and/or retrograde flow from LCA‐ derived collaterals back to the pulmonary artery are also often seen in these older ALCAPA patients [30]. Anomalous right coronary artery from the pulmonary artery may be more benign than ALCAPA, as the right ventricle, which is supplied by the ARCAPA, has more favorable myocardial oxygen supply and demand characteristics when compared with the left ventricle. Other, even rarer variants include both right and left coronary arteries arising from the pulmonary artery, anomalous single coronary artery from the pulmonary artery (ASCAPA) (images with permission) as well as accessory coronary artery arising from the pulmonary artery [40] (Figure 31.13). The rest of this discussion will be restricted to ALCAPA. The pathophysiology of ALCAPA is complex and will vary with the exact anatomy and the changing physiological circumstances as the neonate develops and pulmonary vascular resistance (PVR) and pressure falls. The ALCAPA vessel provides a connection between the pulmonary artery and the myocardium supplied by the left coronary artery. Left ventricular coronary perfusion is dependent on diastolic perfusion, as the pressure generated by left ventricular contraction prevents coronary perfusion during systole. In the early neonatal period, PVR and pulmonary artery pressure are high, compared with later in life. In this phase, the left ventricle may have adequate oxygenation, albeit with mixed venous blood with relatively low hemoglobin oxygen saturation, which can be adequate for left ventricular function. As PVR and pulmonary artery pressure decrease, flow in the ALCAPA vessel may reverse, acting as a fistula and causing run‐off of coronary blood flow to the pulmonary artery, creating a “coronary artery steal” and worsening left ventricular perfusion. This will cause ventricular ischemia, which will lead to ventricular dysfunction, especially in diastole, which will lead to an increase in left ventricular diastolic pressures, thus further decreasing the perfusion pressure. The “coronary steal” may also affect right ventricular perfusion in both systole and diastole, if there are significant collaterals, but the presence of some systolic perfusion of the right ventricle muscle, as in normal coronary physiology, is protective. A peculiar potential benefit of the reverse flow in the ALCAPA vessel (towards the pulmonary origin) is that this may help to maintain PVR and pressure, potentially delaying the onset of symptoms. Figure 31.13 Anomalous single coronary artery arising from the pulmonary artery. (A) Aortogram with absent coronary ostia. (B) Coronary filling after contrast injection into the pulmonary artery demonstrating a short single coronary artery (1) bifurcating into right (2) and left (3) branches. (Source: Machovec et al. [40]. Reproduced with permission of Wolters Kluwer Health, Inc.) The worsening left ventricular function will be associated with dilation of the left ventricle. Left heart dilation may be associated with a shift of the interventricular septum towards the right, with associated impairment of right heart function. Mitral valve regurgitation can be induced by both dilatations of the left atrioventricular ring and ischemic dysfunction of the papillary muscles. This will lead to volume loading and further deterioration of left ventricular function with worsening pulmonary congestion and a low output state. The commonest presentation of ALCAPA is at weeks to sometimes months after birth with a condition that is essentially a severe dilated cardiomyopathy of ischemic origin. All infants presenting with dilated cardiomyopathy must be closely screened for anomalies of coronary origin. Variations in the anatomy of the ALCAPA may influence the presentation. As with normal coronary arteries, there may be significant differences in the balance of these arteries. The more that the right coronary is dominant, supplying significant portions of the ventricular septum and other regions of the left ventricle, the more likely it is that there will be a later presentation with less severe deterioration in myocardial function. Similarly, stenosis of the ALCAPA vessel, which may occur at its origin, will actually lessen the impact of the coronary steal and allow more chance for collateral vessels from the right coronary to perfuse the left side of the heart. There is some evidence that these more delayed presentations may have a long‐term negative impact, as the underlying ischemia will have been more long‐lasting, producing fibrosis, and ventricular recovery after repair will be impaired. For patients with a dominant ALCAPA distribution, presentation is likely to be early with severe myocardial dysfunction. Although recovery in this circumstance can be excellent, some patients may have completed infarction of regions of the left ventricle with no prospect of recovery of function with reperfusion, and with a risk of formation of aneurysm of the left ventricle. The more severe early presentations are also at risk of lethal complications before surgical repair can be performed. Additionally, about 10–15% of ALCAPA patients are adult‐type which without surgical repair results in a risk of sudden death before the age of 35 in 80–90% patients. It is for this reason that surgical repair of all those diagnosed as adolescents or adults is recommended [41]. Historically, simple ligation of the ALCAPA vessel was used, improving survival [42]. This would prevent the coronary steal and, depending on the adequacy of collateral circulation, improve perfusion of the left ventricle. Mortality was still high and recovery of left ventricular function usually incomplete. This procedure is no longer performed in major congenital cardiac surgery centers. Current surgical management involves restoring aortic perfusion of the ALCAPA vessel, thus providing a two‐coronary circulation originating from the aorta, usually by mobilization and reimplantation of the ALCAPA vessel onto the aorta [43–45] (Figure 31.14). A specific example that can be used for a very short ALCAPA vessel is the Takeuchi procedure. This involves using an intra‐arterial pulmonary baffle to direct flow from a surgically created aortopulmonary window to supply the origin of the ALCAPA vessel with systemic arterial blood in situ, with patch expansion of the pulmonary artery to allow space for the baffle and prevent pulmonary obstruction [46–48](Figure 31.15). The unit managing these cases should be able to provide extracorporeal circulatory support with a ventricular assist device (VAD) or extracorporeal membrane oxygenation (ECMO) if required. The techniques may be necessary as part of preoperative resuscitation or for support of the patient in the early postoperative period, as the recovery of myocardial function is usually not immediate [49]. Imamura et al. reported a series of 26 ALCAPA operations over a 20‐year period [50]. Twenty‐one patients did not require ECMO support and five required ECMO for a mean of 10.7 ± 6.7 days. One patient in each group required cardiac transplantation, and four others required six reoperations (five for MR). There were no deaths in the series, and actuarial freedom from transplantation or reoperation at 5 years was 0% in the ECMO group, and 92% in the non‐ECMO group. Chapter 37 presents a detailed discussion of mechanical support of the circulation. Figure 31.14 Direct aortic reimplantation of the anomalous left coronary artery from the pulmonary artery. The anomalous ostium is excised with a button of the pulmonary artery wall. This button is then implanted into the left lateral side of the ascending aorta. The resulting defect is closed with a pericardial patch (Source: Arciniegas [45]. Reproduced with permission of Elsevier.) There is controversy about the role of surgical procedures to improve mitral valve regurgitation at the primary operation because mitral valve function usually improves as improved myocardial function results in decreased end‐diastolic volume and better papillary muscle function [51]. In a series of 25 operations for ALCAPA, Kudumula et al. repaired the mitral valve only for moderate to severe MR, accompanied by structural mitral valve abnormalities, observed in four patients [52]. Two patients had mitral valve cleft, one had a prolapse, and one had a dysplastic double orifice mitral valve. One patient with a structural mitral valve abnormality that was not repaired at the original operation developed severe MR and required repair at a second operation. Of 14 patients in the series without structural mitral valve problems with moderate or severe MR, 13/14 had none or mild MR at the time of follow‐up. A Saudi Arabian retrospective observational cohort of 29 patients from 1999 to 2018 analyzed by Ismail showed similar findings (Figure 31.16). Spontaneous regression of MR was reported by Ismail et al. In these patients, LV systolic function showed echocardiographic improvement within 6 weeks and returned to normal within 1 year’s time and in addition somatic growth of children improved 6 months after surgical repair [53]. Some surgeons still argue that there is a reasonable argument that surgical techniques that decrease the mitral valve annulus size but allow for future growth may improve mitral valve function acutely, improving the performance of the dysfunctional early postoperative heart. Although repairing the mitral valve does increase the cross‐clamp time on an already ischemic myocardium, this does not seem to have a clinically significant effect on the later normalization of LV function or other postoperative outcomes. According to a 2016 study by Naimo et al. which evaluated 42 ALCAPA patients, late reoperation was uncommon in the 33 patients who did not undergo concomitant MV repair despite MR ranging from none to severe. In fact, late MV reoperation was only needed in 14% at 5 & 10 years, and 19% in 20 years after original surgery. Persistent MR does warrant close followup and late MV operative repair may eventually be necessary in some patients [54]. Figure 31.15 Takeuchi procedure through median sternotomy with extracorporeal circulation and cross‐clamped aorta. Left: The tunnel for coronary flow has been made between the aorta and the anomalous left coronary artery by means of a flap of pulmonary artery wall. Middle: Construction of the tunnel to direct flow from the aortic root to the anomalous left coronary artery. Right: The pulmonary artery is then reconstructed with pericardium. (Source: Reproduced with permission of Texas Children’s Hospital.) Figure 31.16 Spontaneous regression of mitral regurgitation after ALCAPA repair. MR, mitral regurgitation. (Source: Ismail et al. [53] / Cureus / CC BY 4.0.) The degree of myocardial dysfunction is the factor that dominates the condition and prognosis of these patients throughout the perioperative period. Preoperatively, the patient with ALCAPA may be receiving cardiorespiratory support, ranging from none to an intubated ventilated patient on ECMO. In between these extremes will be patients with various degrees of inotropic support receiving tailored vasodilators or, in some cases, vasoconstrictors. The general principle that a failing heart, especially with MR, will have output improved by systemic vasodilation is sound, but the severity of the dysfunction in some of these patients means there may be a role for vasoconstrictors. If the patient is already hypotensive, it is possible that the vasodilation is actually excessive for that heart and results in greater tachycardia. The poor coronary perfusion is worsened by both systemic hypotension and tachycardia. In this scenario of myocardial dysfunction with relatively excessive vasodilation, judicious use of vasoconstrictors may improve blood pressure and decrease heart rate, improving coronary perfusion while maintaining an acceptable cardiac output. The patient should be monitored for signs of the inability of the heart to cope with the increased afterload or worsening MR. Preoperative assessment of patients with ALCAPA should also include assessment of the complications of the myocardial dysfunction, such as respiratory, renal and hepatic dysfunction, as well as a general medical review. The group of patients with ALCAPA who provide perhaps the greatest challenge for the anesthesiologist comprises those who have been diagnosed after presenting with respiratory distress, but who are stable and do not require acute cardiorespiratory support. They may have intravenous access, and non‐invasive monitors have been used for them. Echocardiography will have confirmed the diagnosis and the critically poor myocardial function. The anesthesiologist will be responsible for the induction of anesthesia and stabilization of the patient prior to the initiation of CPB in a situation where minor changes in sympathetic drive or myocardial function might be associated with cardiac arrest. Induction of anesthesia, intubation, positive pressure ventilation, placing a central venous catheter or transesophageal echocardiography (TEE) probe, excessive anesthesia with myocardial depression or depression of central nervous system‐driven sympathetic activity, or inadequate anesthesia resulting in excessive sympathetic response can all lead to life‐threatening incidents. The anesthesiologist should be prepared to heparinize and defibrillate the patient at any time. The nursing, perfusion, and surgical team should all be prepared for the potential need for emergent sternotomy and initiation of CPB, and they should be in the operating room during the induction of anesthesia. If it is possible to obtain pre‐induction arterial access without upsetting the patient, this is highly desirable for the earliest warning of cardiovascular compromise. This can sometimes be accomplished in young infants with local anesthesia, either topically or by injection, along with 50% N2O inhalation or small analgesic doses of fentanyl or other opioids intravenously. The induction of anesthesia in patients with ALCAPA requires great care in the slow titration of small increments of whatever drugs are selected to minimize the myocardial depression and vasodilation that accompanies the usual doses of most of these drugs. Opioids, such as fentanyl and remifentanil, although tending to spare myocardial function, can rapidly depress central sympathetic drive, causing relative bradycardia and vasodilation, which may be poorly tolerated by these patients. Similar problems can arise even with drugs, such as etomidate and ketamine, that tend to preserve sympathetic function and cardiovascular stability in normal patients. Ketamine is a direct myocardial depressant, and these hemodynamic effects are most commonly seen in the patient who has depletion of endogenous catecholamines, such as the infant with ALCAPA. Ketamine is also implicated as possibly inducing brain injury in the developing brain and some anesthesiologists avoid it in this age group. The complex risk balance of choice of agent and combination of agents for ALCAPA patients makes judicious, cautious use of agents with which the anesthetist is very familiar a reasonable choice. Preoperative fluid restriction to manage pulmonary congestion may make the patient especially prone to hypotension with the initiation of positive pressure ventilation. Judicious intravascular volume loading at this time may prevent this. Successful transition to anesthesia and positive pressure ventilation may improve lung function and create some afterload reduction, which may improve left ventricular function. Lung compliance is usually low due to pulmonary congestion, and significant ventilator pressures are commonly required. Maintenance of elevated PVR will minimize the “coronary steal” due to the ALCAPA flow into the pulmonary artery. Avoiding a high inspired oxygen concentration and maintaining a normal or moderately high arterial carbon dioxide level are key strategies for maintaining PVR, but pulmonary congestion secondary to left heart failure may mean that increased inspired oxygen levels and high ventilator pressures will be required. Significant PEEP may serve both to improve function in the congested lung and to maintain PVR. Increasing ventilatory pressures, particularly PEEP, will require attention to maintaining adequate intravascular volume to support ventricular filling and avoid low cardiac output and systemic hypotension. Rapid repetitions of non‐invasive blood pressure monitoring should be continued until intra‐arterial continuous blood pressure monitoring is available. Adequate monitoring of the circulation, respiration, and depth of anesthesia in these patients requires constant vigilance. It is advantageous to have at least one, and perhaps two, other pairs of experienced hands to perform the procedures required to prepare the patient for surgery after induction of anesthesia, so one anesthesiologist can focus on the monitoring and anesthesia. Patients with ALCAPA who have been intubated, ventilated and had appropriate vascular access obtained preoperatively may be sicker in many respects than the previous scenario, but the anesthesiologist will have to make less of a transition for the patient to anesthesia and establish invasive monitoring and other preparation. It is still important that there is great vigilance in the transfer of the patient to the operating room and that the whole operative team is present to initiate CPB at short notice before the transfer commences. Patients who have already been placed on mechanical circulatory support present challenges in transfer, but provided, there is no interruption to that support, they are very safely supported. The anesthesiologist must ensure the patient has adequate intravascular volume to allow good venous drainage into the external mechanical circuit. Monitoring the inlet pressure of the circuit can greatly assist this process. Delivery of cardioplegia in patients with ALCAPA requires variation from routine procedures. Ensuring adequate cardioplegia flow to all the myocardium is crucial for the preservation of myocardial function. Usually, this can be achieved by anterograde delivery of cardioplegia via both the normally placed right coronary and the anomalous left coronary, the latter usually achieved by cannulating and perfusing into the pulmonary artery clamped so that that flow is restricted to the ALCAPA vessel. After the cross‐clamp is removed and the heart is perfused, recovery of electrical and motor function of the heart may be slow. As long as the heart is not distending, perfusion pressure and myocardial perfusion are adequate, allowing the heart considerable time to eject without the work of supporting the full circulation, which is beneficial for myocardial recovery. Forcing the heart to support the circulation with early weaning from CPB and administration of large doses of inotropes carries a high risk of return to CPB and of causing myocardial injury. TEE is crucially important in these infants to assess myocardial function and the degree of MR after repair. Translocation of a coronary artery has the major risks of bleeding from the suture line and poor flow in the coronary, leading to myocardial ischemia and poor function. Blood loss may be minimized by reversal of heparin (as far as possible, but may be limited by the need for mechanical support of the circulation), by optimizing coagulation with appropriate blood products and the use of an antifibrinolytic. Concern about thrombotic and embolic complications may deter some pediatric cardiac units from using these agents. Poor function of the translocated ALCAPA vessel may require revision of the surgery. These patients are at significant risk for myocardial failure and circulatory collapse after weaning from CPB. Depending on the circumstances, leaving the sternum open and closing the skin with a membrane and dressing may be safer than formal closure of the chest. Not closing the sternum will prevent early pressure on the heart and allow room for some postoperative swelling without compression of the heart. The membrane closure also allows rapid access for resuscitation and return to bypass in the early postoperative period. Purse strings for cannulation for bypass may be left in place during this period and removed when the chest is formally closed. For patients who are less stable in the operating room, transitioning from a CPB circuit with venous reservoir to other mechanical support such as left ventricular assist device, biventricular assist device or ECMO will provide circulatory support of the patient while the myocardium recovers and improves function. Occasionally, failure to wean from CPB for other operations, i.e., ventricular septal defect (VSD) with left ventricular dilation and failure, may be due to an unrecognized ALCAPA; Callagan et al. reported this occurrence in a 6‐week‐old patient where pulmonary hypertension was suspected to have prevented severe left ventricular dysfunction, decreased the symptoms and masked the diagnosis of ALCAPA [55]. Coronary angiography confirmed the ALCAPA diagnosis, and emergency reoperation, repair of ALCAPA, and postoperative ECMO were necessary to ensure successful outcome.
CHAPTER 31
Anesthesia for Miscellaneous Cardiac Lesions
Introduction
Vascular rings
Classification, anatomy, and incidence
Vascular ring
No. of patients
Double aortic arch
155
Right aortic arch/left ligamentum
172
Pulmonary artery sling
46
Total
373
Diagnosis of vascular rings
Vascular rings due to double and right aortic arches: anatomy and natural history
N = 92
Current age years, mean ± SD
9 ± 7
RAA‐ALS, n (%)
73 (79)
DAA, n (%)
17 (19)
Pulmonary artery sling, n (%)
2 (2)
Isolated VR, n (%)
75 (82)
RAA‐ALS, n
58
DAA, n
15
Pulmonary artery sling, n
2
Dysmorphic syndrome, n (%)
13 (17)
Prenatal detection 2004‐2015, n PNDX/n total DX (%)
23/53 (43)
VR with intracardiac CHD, n (%)
17 (18)
RAA‐ALS, n
15
DAA, n
2
Dysmorphic syndrome, n (%)
9 (53)
Prenatal detection 2004‐2015, n PNDX/n total DX (%)
3/7 (43)
All dysmorphic syndromes and other malformations, n (%)
22 (24)
Down syndrome, n
4
22q11.2 deletion syndrome, n
6
Miscellaneous noncardiac malformations, n
12
Abbreviations: CHD, congenital heart disease; DAA, double aortic arch; DX, diagnosis; PNDX, prenatal diagnosis; RAA‐ALS, right aortic arch, aberrant left subclavian artery and patent ductus arteriosus or ligamentum; SD, standard deviation; VR, vascular ring.
Pathophysiology of vascular rings due to double or right aortic arches
Surgery for right and double aortic arch
Anesthesia for division of vascular rings related to double or right aortic arches
Double aortic arch (n = 80) b
Right aortic arch (n = 78) b
Stridor
46 (57%)
18 (23%)
Recurrent upper respiratory tract infections
22 (27%)
18 (23%)
Cough
17 (21%)
8 (10%)
Dysphagia
12 (15%)
12 (15%)
Respiratory distress
8 (10%)
13 (17%)
Ventilator preoperatively
7 (9%)
3 (4%)
Innominate artery compression of the trachea: anatomy, diagnosis, and natural history
Pathophysiology of innominate artery compression of the trachea
Surgery for innominate artery compression of the trachea
Anesthesia for the repair of innominate artery compression of the trachea
Pulmonary artery sling with tracheal stenosis: anatomy and natural history
Pulmonary artery sling with tracheal stenosis: pathophysiology
Surgery for pulmonary artery sling with tracheal narrowing
Anesthesia for the repair of pulmonary artery sling and tracheal stenosis
Anomalies of the coronary arteries
Total cohort
Ages 11–14 years
Ages 15–18 years
N = 5169
Frequency (%)
95% CI
n = 4310
Frequency (%)
n = 859
Frequency (%)
Total hr‐CVC
76
1.47
1.16–1.84
62
1.44
14
1.63
hr‐ACAOS
23
0.44
0.28–0.67
20
0.46
3
0.35
L‐ACAOS‐IM
6
0.12
0.04–0.25
6
0.14
0
0
RSV
2
0.04
0.01–0.10
NC
2
0.04
0.01–0.10
HO
2
0.04
0.01–0.10
R‐ACAOS
17
0.33
0.19–0.53
14
0.32
3
0.35
hr‐CMP
14
0.27
0.15–0.45
6
0.14
8
0.93
DCM
11
0.21
0.11–0.38
5
0.12
6
0.70
HCM
3
0.06
0.01–0.17
1
0.02
2
0.23
ECG hr‐CVC
39
0.75
0.54–1.03
36
0.84
3
0.35
Brugada
1
0.02
0.00–0.11
0
0
1
0.12
WPW
4
0.08
0.02–0.20
4
0.09
0
0
QTc ≥ 470 ms
34
0.66
0.46–0.92
32
0.74
2
0.23
NCLV
959
18.55
17.50–19.64
810
18.79
149
17.35
Anomalous pulmonary origins of the coronary arteries: incidence, anatomy, and natural history
Pathophysiology of ALCAPA
Surgery for ALCAPA
Anesthetic considerations for ALCAPA

Full access? Get Clinical Tree
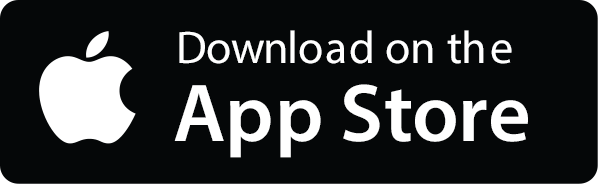
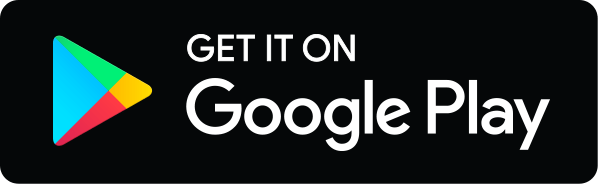