FIGURE 30.1 The power of diaphragmatic contractions (equivalent to the product of contractile force and velocity) during spontaneous breathing (control) and after 3 days of assisted (i.e., patient-triggered) mechanical ventilation (AMV) or controlled mechanical ventilation (CMV). Note that CMV (but not AMV) was associated with a significant reduction in the power output of the diaphragm. Data from Reference 5.
Observations like those in Figure 30.1 indicate that allowing patients to trigger ventilator breaths (e.g., by avoiding controlled ventilation and neuromuscular paralysis) will help to preserve the strength of the diaphragm, and this should facilitate the transition from ventilatory support to spontaneous breathing. (The role of diaphragm weakness in weaning from the ventilator is described later in the chapter.)
Physical Rehabilitation
Prolonged bed rest and physical inactivity during mechanical ventilation often leads to deconditioning and generalized muscle weakness, and this is considered a contributing factor in ventilator-dependent patients who have difficulty in the transition to unassisted breathing. Supporting this contention are studies showing that early physical rehabilitation, including ambulation, is associated with a shorter duration of mechanical ventilation (7). Therefore, early and regular physical rehabilitation (including ambulation in patients who are awake and hemodynamically stable) is encouraged in selected patients to facilitate the transition to spontaneous breathing.
Sedation Practices
Several studies have shown that both deep sedation (where the patient is not arousable) and sustained use of benzodiazepines (midazolam and lorazepam) for sedation are associated with delays in discontinuing mechanical ventilation (8). As a result of these studies, the most recent guidelines on sedation in ventilator-dependent patients (8) include the following recommendations:
1. Maintain a light level of sedation, where patients are easily aroused.
2. Avoid or minimize the use of benzodiazepines for sedation. Non-benzodiazepine sedatives include propofol and dexmedetomidine, which are described in Chapter 51.
Table 30.1 Checklist for Identifying Candidates for a Trial of Spontaneous Breathing
Readiness Criteria
The management of ventilator-dependent patients requires constant vigilance for signs that ventilatory support may no longer be necessary. These signs are listed in Table 30.1. Candidates for possible removal of mechanical ventilation should have adequate gas exchange in the lungs (i.e., PaO2/FIO2 >150–200 mm Hg and a normal or baseline arterial PCO2) while breathing non-toxic concentrations of oxygen (FIO2 <50%) and at low levels of PEEP (<8 cm H2O). In addition, there should be no evidence of myocardial ischemia, severe tachycardia (>140 beats/min), circulatory shock, or ongoing sepsis (e.g., fever).
When the criteria in Table 30.1 have been satisfied, the patient should be removed from the ventilator briefly to obtain the measurements listed in Table 30.2. These measurements (which are called “weaning parameters”) are used to predict the likelihood of success or failure during a trial of spontaneous or unassisted breathing. However, as indicated by the range of likelihood ratios, each of these parameters can be a poor predictor of success or failure in individual patients. (Monitoring serial changes in these parameters during a trial of spontaneous breathing may have a greater predictive value than measurements obtained immediately after removing ventilatory support) (9). Because of the variable predictive value of the weaning parameters in Table 30.2, the emerging consensus is that trials of spontaneous breathing can begin when the readiness criteria in Table 30.1 are satisfied.
Table 30.2 Measurements Used to Predict a Successful Trial of Spontaneous Breathing
THE SPONTANEOUS BREATHING TRIAL
The traditional approach to discontinuing mechanical ventilation emphasized a gradual reduction in ventilatory support (over hours to days), and this created unnecessary delays in removing ventilatory support for patients who were capable of unassisted breathing. (This delayed approach is still evident in the practice of placing patients back on a ventilator at night to “rest them”.) In contrast, spontaneous breathing trials (SBTs) are conducted with no ventilatory support, so that patients capable of unassisted breathing can be identified quickly. There are two methods of conducting an SBT, as described next.
Using the Ventilator Circuit
SBTs are often conducted while the patient breathes through the ventilator circuit. The advantage of this method is the ability to monitor the tidal volume (VT) and respiratory rate (RR), since rapid, shallow breathing (indicated by an increase in the RR/VT ratio) is a common breathing pattern in patients who fail the SBT (9). The drawback of this method is the resistance to breathing through the ventilator circuit, which can increase the work of breathing (particularly in patients who are breathing rapidly).
Pressure Support
To counteract the resistance to breathing through the ventilator circuit, low levels of pressure support (5 cm H2O) are routinely used when SBTs are conducted through the ventilator circuit. (For a description of pressure support, see pages 511–512.) However, as demonstrated in Figure 30.2, the use of pressure support results in only a small and insignificant decrease in the work of breathing (10). These results suggest that the benefit of low-level pressure support is minimal, and clinically irrelevant.
Disconnecting the Ventilator
SBTs can also be conducted when the patient is disconnected from the ventilator, using the simple circuit design illustrated in Figure 30.3. A source of O2 (usually from a wall outlet) is delivered to the patient at a high flow rate (higher than the patient’s inspiratory flow rate), and this not only facilitates the inhalation of O2, it also carries exhaled CO2 out to the atmosphere, to prevent CO2 rebreathing. Because this circuit employs a T-shaped adapter, it is popularly known as a T-piece circuit.
FIGURE 30.2 The work of breathing (in joules per liter) during spontaneous breathing trials conducted with and without the aid of pressure support ventilation (PSV) at 5 cm H2O, and one hour after extubation. The asterisk indicates a significant difference at the p=0.05 level. Data from Reference 10.
The work of breathing is considered to be lower when breathing through a T-piece circuit compared to a ventilator circuit (although this is unproven). The major disadvantage of the T-piece circuit is the inability to monitor the respiratory rate and tidal volume.
FIGURE 30.3 Simple breathing circuit for spontaneous breathing trials that are independent of the ventilator. The T-shaped adapter in the circuit is responsible for the popular term T-piece that is used for this circuit.
Which Method Is Preferred?
There is no clinically proven advantage with either method of SBT (3). However, the T-piece method has the following theoretical advantages: (a) it is better suited for patients with increased ventilatory demands (which is a common cause of difficulty during an SBT), and (b) it is a closer approximation of the normal conditions for breathing than breathing through a ventilator circuit with low-level pressure support as an adjunct.
Success vs. Failure
Success or failure of a trial of unassisted breathing is judged by one or more of the following parameters:
1. Signs of respiratory distress; e.g., agitation, diaphoresis, rapid breathing, and use of accessory muscles of respiration.
2. Signs of respiratory muscle weakness; e.g., paradoxical inward movement of the abdominal wall during inspiration.
3. Adequacy of gas exchange in the lungs; e.g., arterial O2 saturation, PaO2/FIO2 ratio, arterial PCO2, and gradient between end-tidal and arterial PCO2.
4. Adequacy of systemic oxygenation; e.g., central venous O2 saturation.
A majority of patients (∼80%) who tolerate SBTs for 2 hours can be permanently removed from the ventilator (1,2). For patients with prolonged periods of ventilator dependence (e.g., 3 or more weeks), longer trials of spontaneous breathing may be necessary before claiming success. For patients who fail initial attempts at unassisted breathing, daily SBTs are advised to insure timely removal of ventilatory support.
Rapid Breathing
Rapid breathing during SBTs may be the result of breathlessness (dyspnea) provoked by anxiety rather than ventilatory failure (12). Monitoring the tidal volume can be useful in distinguishing anxiety from ventilatory failure; i.e., anxiety produces hyperventilation, where the respiratory rate and tidal volume are both increased, whereas ventilatory failure usually produces rapid and shallow breathing, where the respiratory rate is increased but the tidal volume is decreased. Therefore, for the patient who develops rapid respirations during a trial of unassisted breathing, an increased tidal volume suggests anxiety as the underlying problem, while a decreased tidal volume suggests ventilatory failure. Worsening of gas exchange may not distinguish between anxiety and ventilatory failure for the reasons described next.
Adverse Effects
Regardless of the cause, rapid breathing during SBTs can be detrimental in several ways, as summarized below.
1. In patients with asthma and COPD, rapid breathing promotes hyperinflation and intrinsic PEEP, which can: (a) decrease the cardiac output, (b) increase dead space ventilation, (c) decrease lung compliance, and (d) produce diaphragm dysfunction by flattening the diaphragm.
2. For patients with infiltrative lung disease (e.g., ARDS), rapid breathing reduces ventilation in diseased lung regions (where time constants for alveolar ventilation are prolonged), and this promotes alveolar collapse and hypoxemia.
3. For all patients with acute respiratory failure, rapid breathing can increase whole-body O2 consumption, which places an added burden on systemic O2 transport.
Management
If ventilatory failure is suspected as the cause of rapid breathing, the patient should be placed back on the ventilator. If anxiety is suspected as the culprit, administration of a sedative drug should be considered. Opiates may be preferred in this situation because they are particularly effective in curbing the sensation of dyspnea (13). Despite the fear of opiate use in patients with COPD, these drugs have been used safely for relief of dyspnea in patients with severe or end-stage COPD (13).
A failed trial of spontaneous breathing is usually a sign that the pathologic condition requiring ventilatory support needs further improvement. However, there are other conditions that create difficulties in discontinuing mechanical ventilation, and the principal ones are described next.
Cardiac Dysfunction
Cardiac dysfunction can develop during a trial of spontaneous breathing, and this condition has been identified in 40% of failed weaning trials (14). Potential sources of cardiac dysfunction in this situation include: (a) negative intrathoracic pressures, which increase left ventricular afterload (see pages 159–161), (b) hyperinflation and intrinsic PEEP, which impair venous return and restrict ventricular distensibility, and (c) silent myocardial ischemia (15). The adverse effects of cardiac dysfunction include pulmonary congestion, and a decrease in the contractile strength of the dia-phragm (16). This latter effect is explained by the fact that the diaphragm (like the heart) maximally extracts O2 under normal conditions, and thus is highly dependent on the cardiac output for its O2 supply.
Monitoring
The following approaches can be used to detect cardiac dysfunction in patients who fail repeated attempts at discontinuing mechanical ventilation.
CARDIAC ULTRASOUND: Cardiac ultrasound is the most useful tool for detecting changes in systolic and diastolic function during failed trials of unassisted breathing. In fact, cardiac ultrasound is responsible for the recent discovery that diastolic dysfunction is a major determinant in the inability to wean from mechanical ventilation (17).
CENTRAL VENOUS O2 SATURATION: A decrease in cardiac output is accompanied by a compensatory increase in peripheral O2 extraction and a subsequent decrease in venous O2 saturation. (See pages 183–184 for a description of the factors that influence venous O2 saturation.) Therefore, a decrease in central venous O2 saturation (ScvO2) during a failed SBT could signal the appearance of cardiac dysfunction. The changes in mixed venous O2 saturation (SvO2) during successful and failed trials of spontaneous breathing are shown in Figure 30.4 (18). The SvO2 decreased during the failed trials but not during the successful trials, suggesting that cardiac dysfunction may be responsible for the failure to sustain spontaneous breathing. The ScvO2 mimics the behavior of the SvO2, and is more easily obtained (see pages 183–184).
B-TYPE NATRIURETIC PEPTIDE: Clinical studies have shown that plasma levels of B-type natriuretic peptides are significantly increased when cardiac dysfunction develops during a trial of spontaneous breathing (14, 19). Therefore, it is possible that serial measurements of B-type natriuretic peptides can provide a simple and noninvasive method for detecting cardiac dysfunction during failed trials of spontaneous breathing. (See pages 242–243 for more information the use of B-type natriuretic peptides as biomarkers for heart failure.)
FIGURE 30.4 Mixed venous O2 saturation (SvO2) during successful and failed trials of spontaneous breathing. Data from Reference 17.
Management
There is surprisingly little information on methods for correcting the cardiac dysfunction that develops during spontaneous breathing trials. Patients who develop systolic dysfunction should benefit from continuous positive airway pressure (CPAP), which promotes cardiac output by cancelling the afterload-increasing effect of negative intrathoracic pressure (20,21). Since CPAP is delivered noninvasively, it will not prevent the removal of mechanical ventilation, including extubation.
Respiratory Muscle Weakness
Respiratory muscle weakness is always near the top of the list for potential causes of difficulty in removing ventilatory support. However, the role of respiratory muscle weakness in difficult-to-wean patients is not clear. The following are some potential sources of respiratory muscle weakness in ventilator-dependent patients.
Potential Sources
MECHANICAL VENTILATION: As mentioned earlier (and shown in Figure 30.1), mechanical ventilation is a recognized cause of diaphragm weakness (5), primarily when patients are not allowed to trigger a ventilator breath (6). However, the perception that ventilator-associated dia-phragm weakness can be a source of failed SBTs is not supported by a clinical study showing that the strength of the diaphragm can actually increase during failed trials of spontaneous breathing (22). Furthermore, the notion that diaphragm weakness can be a source of inadequate ventilation is not supported by observations in patients who lack functioning diaphragms (from paralysis or injury), who show no evidence of inadequate ventilation, and have normal levels O2 and CO2 in their blood (23). These findings indicate that diaphragm weakness is not deserving of its reputation as an important factor in prolonging ventilator dependency.
CRITICAL ILLNESS NEUROMYOPATHY: The conditions known collectively as critical illness polyneuropathy and myopathy are inflammatory conditions involving peripheral nerves and skeletal muscle that typically appear in patients with severe sepsis and multiorgan failure, and are recognized only when patients fail to wean from mechanical ventilation (24). There is no specific treatment for these conditions, and the weakness can persist for months. A more detailed description of these conditions is included in Chapter 45.
ELECTROLYTE DEPLETION: Magnesium and phosphorous depletion can promote respiratory muscle weakness (24,25), but the clinical relevance of this effect is unproven. Nevertheless, deficiencies in these electrolytes should be corrected in patients who fail repeated attempts to discontinue mechanical ventilation.
Monitoring
Uncertainty about the role of respiratory muscle weakness in failure to remove ventilatory support is partly a reflection of the lack of reliable and readily obtained measures of respiratory muscle strength.
MAXIMUM INSPIRATORY PRESSURE: The standard clinical measure of respiratory muscle strength is the maximum inspiratory pressure (PImax), which is the negative pressure that is generated by a maximum inspiratory effort against a closed airway (27,28). The normal values of PImax vary widely, but mean values of -120 cm H2O and -84 cm H2O have been reported for adult men and women, respectively (28). Ventilation at rest is threatened when the PImax drops to -15 to -30 cm H2O, which are the threshold values for predicting successful trials of spontaneous breathing (see Table 30.2). Unfortunately, patients with acute respiratory failure have difficulty performing the maneuvers involved in the measurement of PImax. As a result, the PImax is not measured regularly in patients who require ventilatory support.
ULTRASOUND: Ultrasound has recently emerged as a potential method of assessing diaphragm strength. The ultrasound measures of diaphragm strength include the thickness of the diaphragm, and the length of excursion of the diaphragm during inspiration (29). In a preliminary study that used ultrasound assessments of diaphragm strength in patients who were weaned from mechanical ventilation, there was a significant correlation between failed SBTs and diaphragm weakness identified by ultrasound (29).
The reliability of ultrasound measurements for detecting diaphragm weakness is uncertain at the present time because the criteria for diaphragm weakness are arbitrary, and have not been validated. (This will require ultrasound measurements in large numbers of normal subjects to determine the range of normal measurements.)
Management
When respiratory muscle weakness is strongly suspected, trials of spontaneous breathing should continue, but should be terminated before patients show evidence of respiratory distress (to avoid aggravating the weakness). Strategies designed to promote muscle strength, such as patient-triggered ventilation and physical rehabilitation (described earlier), are considered particularly important in patients with documented muscle weakness.
EXTUBATION
Once there is evidence that mechanical ventilation is no longer necessary, the next step is to remove the artificial airway. This section focuses on removing endotracheal tubes, although some of the principles also apply to removing tracheostomy tubes. (The removal of tracheostomy tubes is a more gradual process, and often occurs after patients leave the ICU.)
Extubation should never be performed to reduce the work of breathing, because the work of breathing can actually increase after extubation, as demonstrated in Figure 30.2. (The increased work of breathing can be the result of an increased respiratory rate or breathing through a narrowed glottis, but it occurs in patients who tolerate extubation, so it is not always a cause for concern.) The considerations that must be addressed prior to extubation include: (a) the patient’s ability to clear secretions from the airways, and (b) the risk of symptomatic laryngeal edema following extubation.
Airway Protective Reflexes
The ability to protect the airway from aspirated secretions is determined by the strength of the gag and cough reflexes. Cough strength can be assessed by holding a piece of paper 1–2 cm from the end of the endotracheal tube and asking the patient to cough. If wetness appears on the paper, the cough strength is considered adequate (30). Diminished strength or even absence of cough or gag reflexes will not necessarily prevent extubation, but will identify patients who need special precautions to prevent aspiration.
Laryngeal Edema
Upper airway obstruction from laryngeal edema is the major cause of failed extubations, and is reported in 5–22% of patients who have been intubated for longer than 36 hours (3,31,32). Contributing factors include difficult and prolonged intubation, endotracheal tube diameter, and self-extubation.
The Cuff-Leak Test
The cuff-leak test measures the volume of inhaled gas that escapes through the larynx when the cuff on the endotracheal tube is deflated. This test is designed to determine the risk of symptomatic upper airway obstruction from laryngeal edema after the endotracheal tube is removed. According to a recent analysis of the cuff leak test (33), the absence of an air leak indicates a high risk of upper airway obstruction following extubation, but the presence of an air leak does not indicate a low risk of upper airway obstruction following extubation, regardless of the volume of the leak.
The value of the cuff leak test has been debated for years, and the test is not universally accepted. Since the results of a cuff leak test do not alter patient management, including the decision to extubate, the clinical relevance of the test is unproven.
Pretreatment with Steroids?
Two clinical studies have shown that pretreatment with intravenous corticosteroids ( methyl- prednisolone, 20–40 mg every 4–6 hrs) for 12 to 24 hours prior to extubation results in fewer cases of laryngeal edema and upper airway obstruction following extubation, and fewer reintubations (33,34). The results of one of these studies is shown in Figure 30.5. Steroid pretreatment in this study consisted of three doses of intravenous methylprednisolone (20 mg every 4 hours), with the first dose given 12 hours prior to a planned extubation. Note that this pretreatment was associated with about a 7-fold decrease in the incidence of symptomatic laryngeal edema following extubation, and a 50% drop in reintubation rate.
Although the use of corticosteroids for “anything that swells” is questionable (35), the results of the study shown in Figure 30.5 are compelling enough to consider a brief period (12 to 24 hrs) of corticosteroid therapy prior to planned extubations, particularly in patients who have a high risk of post-extubation laryngeal edema (e.g., from prior self-extubations). A single dose of methylprednisolone (40 mg IV) given one hour prior to extubation did not reduce the incidence of post-extubation laryngeal edema in one study (36), so there is no reason to administer steroids only at the time of extubation.
Postextubation Stridor
The first sign of a significant laryngeal obstruction may be stridorous breathing (noisy breathing), also called stridor. The sounds may be high-pitched and wheezy, or low-pitched and harsh, but they are always audible without a stethoscope, and they are always most apparent during inspiration. This inspiratory prominence is due to the extrathoracic location of laryngeal obstruction because negative intrathoracic pressures generated during inspiration are transmitted to the upper airways outside the thorax, and this results in a narrowing of the extrathoracic airways during inspiration. Therefore, extrathoracic obstructions are always magnified during inspiration.
FIGURE 30.5 Results of a large, multicenter study showing the effects of pretreatment with a corticosteroid (methylprednisolone, 20 mg IV every 4 hrs for the 12 hours prior to extubation) on the incidence of post-extubation laryngeal edema and the rate of reintubation. Data from Reference 30.
Post-extubation stridor is apparent within 30 minutes of extubation in a large majority (∼80%) of cases (30), but delays in appearance of up to 2 hours can occur (personal observation). Reintubation is not always re-quired, but close scrutiny is required because there is no proven method for reducing laryngeal edema after extubation.
Aerosolized Epinephrine
Inhalation of an epinephrine aerosol (2.5 mL of 1% epinephrine) is a popular practice for post-extubation stridor. However, while effective in children (36), this practice is unproven in adults. Aerosol therapy with a racemic mixture of epinephrine (which has equal amounts of the levo- and dextro- isomers) is also popular for post-extubation stridor, but clinical studies in children have shown no advantage with racemic epinephrine over standard (l-isomer) epinephrine (37).
Noninvasive Ventilation
Noninvasive ventilation (which is described on pages 526–531) is effective in reducing the rate of reintubation when used immediately after extubation in patients with a high risk of laryngeal edema (38), but similar success has not been demonstrated in patients who develop post-extubation respiratory failure (39). Thus, the benefit of noninvasive ventilation occurs when it is used as a preventive measure early after extubation.
A FINAL WORD
Be Vigilant
The ultimate goal of mechanical ventilation is to no longer need it, and the introductory quote by Sir William Osler is intended to emphasize that vigilance is required to identify when you have reached this goal in a timely manner. Vigilance involves early recognition of candidates for trials of unassisted breathing (with daily assessments using the readiness criteria in Table 30.1), and early recognition that the candidates can sustain spontaneous ventilation (with trials of spontaneous breathing). This approach will free patients from mechanical ventilation without delay, and end the misfortune of being tethered to a machine.
REFERENCES
Clinical Practice Guidelines
1. MacIntyre NR, Cook DJ, Ely EW Jr, et al. Evidence-based guidelines for weaning and discontinuing ventilatory support: a collective task force facilitated by the American College of Chest Physicians, the American Associa-tion for Respiratory Care, and the American College of Critical Care Med-icine. Chest 2001; 120(Suppl):375S–395S.
Reviews
2. MacIntyre NR. Evidence-based assessments in the ventilator discontinuation process. Respir Care 2012; 57:1611–1618.
3. McConville JF, Kress JP. Weaning patients from the ventilator. New Engl J Med 2012; 367:2233–2239.
4. Thille AW, Cortes-Puch I, Esteban A. Weaning from the ventilator and extubation in ICU. Curr Opin Crit Care 2013; 19:57–64.
Preliminary Concerns
5. Petrof BJ, Jaber S, Matecki S. Ventilator-induced diaphragm dysfunction. Curr Opin Crit Care 2010; 16:19–25.
6. Sassoon CSH, Zhu E, Caiozzo VJ. Assist-control mechanical ventilation attenuates ventilator-induced diaphragm dysfunction. Am J Respir Crit Care Med 2004; 170:626–632.
7. Mendez-Tellez PA, Needham DM. Early physical rehabilitation in the ICU and ventilator liberation. Respir Care 2012; 57:1663–1669.
8. Barr J, Fraser GL, Puntillo K, et al. Clinical practice guidelines for the management of pain, agitatiom, and delirium in adult patients in the intensive care unit. Crit Care Med 2013; 41:263–306.
9. Kreiger BP, Isber J, Breitenbucher A, et al. Serial measurements of the rapid-shallow breathing index as a predictor of weaning outcome in elderly medica l patients. Chest 1997; 112:1029–1034.
Spontaneous Breathing Trials
10. Mehta S, Nelson DL, Klinger JR, et al. Prediction of post-extubation work of breathing. Crit Care Med 2000; 28:1341–1346.
11. Ely W, Baker AM, Dunagen DP, et al. Effect of duration of mechanical ventilation of identifying patients capable of breathing spontaneously. N Engl J Med 1996; 335:1864–1869.
12. Bouley GH, Froman R, Shah H. The experience of dyspnea during weaning. Heart Lung 1992; 21:471–476.
13. Raghavan N, Webb K, Amornputtisathaporn N, O’Donnell DE. Recent advances in pharmacotherapy for dyspnea in COPD. Curr Opin Pharmacol 2011; 11:204–210.
Failure of Spontaneous Breathing
14. Grasso S, Leone A, De Michele M, et al. Use of N-terminal pro-brain natriuretic peptide to detect acute cardiac dysfunction during weaning failure in difficult-to-wean patients with chronic obstructive pulmonary disease. Crit Care Med 2007; 35:96–105.
15. Srivastava S, Chatila W, Amoateng-Adjepong Y, et al. Myocardial ischemia and weaning failure in patients with coronary artery disease: an update. Crit Care Med 1999; 27:2109–2112.
16. Nishimura Y, Maeda H, Tanaka K, et al. Respiratory muscle strength and hemodynamics in heart failure. Chest 1994; 105:355–359.
17. Papanickolaou J, Makris D, Saranteas T, et al. New insights into weaning from mechanical ventilation: left ventricular diastolic dysfunction is a key player. Intensive Care Med 2011; 37:1976–1985.
18. Jubran A, Mathru M, Dries D, Tobin MJ. Continuous recordings of mixed venous oxygen saturation during weaning from mechanical ventilation and the ramifications thereof. Am Rev respir Crit Care Med 1998; 158:1763–1769.
19. Zapata L, Vera P, Roglan A, et al. B-type natriuretic peptides for prediction and diagnosis of weaning failure from cardiac origin. Intensive Care Med 2011; 37:477–485.
20. Naughton MT, Raman MK, Hara K, et al. Effect of continuous positive airway pressure on intrathoracic and left ventricular transmural pressures in patients with congestive heart failure. Circulation 1995; 91:1725–1731.
21. Bradley TD, Holloway BM, McLaughlin PR, et al. Cardiac output response to continuous positive airway pressure in congestive heart failure. Am Rev Respir Crit Care Med 1992; 145:377–382.
22. Swartz MA, Marino PL. Diaphragm strength during weaning from mechanical ventilation. Chest 1985; 88:736–739.
23. LaRoche CM, Carroll N, Moxham J, Green M. Clinical significance of severe isolated diaphragm weakness. Am Rev Respir Dis 1988; 138:862–866.
24. Hudson LD, Lee CM. Neuromuscular sequelae of critical illness. N Engl J Med 2003; 348:745–747.
25. Benotti PN, Bistrian B. Metabolic and nutritional aspects of weaning from mechanical ventilation. Crit Care Med 1989; 17:181–185.
26. Malloy DW, Dhingra S, Solren F, et al. Hypomagnesemia and respiratory muscle power. Am Rev Respir Dis 1984; 129:427–431.
27. Mier-Jedrzejowicz A, Brophy C, Moxham J, Geen M. Assessment of diaphragm weakness. Am Rev Respir Dis 1988; 137:877–883.
28. Bruschi C, Cerveri I, Zoia MC. et al. Reference values for maximum respiratory mouth pressures: A population-based study. Am Rev respir Dis 1992; 146:790–793.
29. Kim WY, Suh HJ, Hong S-S, et al. Diaphragm dysfunction assessed by ultrasonography: Influence on weaning from mechanical ventilation. Crit Care Med 2011; 39:2627–2630.
Extubation
30. Khamiees M, Raju P, DeGirolamo A, et al. Predictors of extubation outcome in patients who have successfully completed a spontaneous breathing trial. Chest 2001; 120:1262–1270.
31. François B, Bellisant E, Gissot V, et al, for the Association des Réanimateurs du Centre-Quest (ARCO). 12-h pretreatment with methylprednisolone versus placebo for prevention of postextubation laryngeal oedema: a randomized double-blind trial. Lancet 2007; 369:1083–1089.
32. Jaber S, Chanques G, Matecki S, et al. Post-extubation stridor in intensive care unit patients. Risk factors evaluation and importance of the cuff test. Intensive Care Med 2003; 29:63–74.
33. Ochoa ME, del Carmen Marín M, Frutos-Vivar F, et al. Cuff-leak test for the diagnosis of upper airway obstruction in adults: a systematic review and meta-analysis. Intensive Care Med 2009; 35:1171–1179.
34. Cheng K-C, Hou C-C, Huang H-C, et al. Intravenous injection of methylprednisolone reduces the incidence of post-extubation stridor in intensive care unit patients. Crit Care Med 2006; 34:1345–1350.
35. Shemie, S. Steroids for anything that swells: Dexamethasone and postextubation airway obstruction. Crit Care Med: 1996; 24:1613–1614.
36. Gaussorgues P, Boyer F, Piperno D, et al. Do corticosteroids prevent postintubation laryngeal edema? A prospective study of 276 adults. Crit Care Med 1988; 16:649–652.
37. Nutman J, Brooks LJ, Deakins K, et al. Racemic versus l-epinephrine aerosol in the treatment of postextubation laryngeal edema: results from a prospective, randomized, doubleblind study. Crit Care Med 1994; 22:1591–1594.
38. Nava S, Gregoretti C, Fanfulla F, et al. Noninvasive ventilation to prevent respiratory failure after extubation in high-risk patients. Crit Care Med 2005; 33:2465–2470.
39. Hess D. The role of noninvasive ventilation in the ventilator discontinuation process. Respir Care 2012; 57:1619–1625.

Full access? Get Clinical Tree
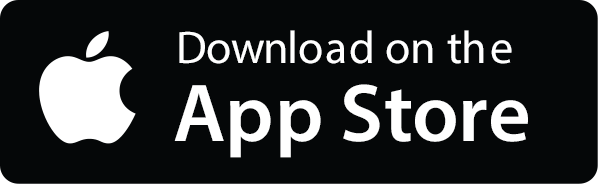
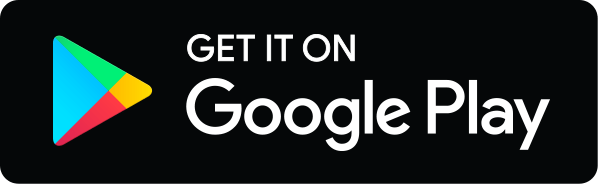