Lisa A. Caplan1, Ehrenfried Schindler2, and David F. Vener3 1 Staff Cardiovascular Anesthesiologist, Arthur S. Keats Division of Pediatric Cardiovascular Anesthesiology, Department of Anesthesiology, Baylor College of Medicine, Houston, TX, USA 2 Department of Anesthesiology and Intensive Care Medicine, University Hospital, Bonn, Germany 3 Arthur S. Keats Division of Pediatric Cardiovascular Anesthesiology, Texas Children’s Hospital, Department of Anesthesiology, Baylor College of Medicine, Houston, TX, USA Patient safety in the operating room (OR) and beyond has long been a driving force in anesthesia care. Technical innovations such as pulse oximetry and capnography, combined with better trainee and practitioner education have dramatically increased safety for our patients and the quality of our anesthesia care. Additionally, newer medications, technologies, and monitoring modalities continue to advance the field. As a result of these systematic changes, anesthesia‐related patient morbidity and mortality have steadily declined across all patient populations. Non‐technical attempts to reduce perioperative complications such as perioperative time‐outs and checklists have become another major focus of various organizations such as the World Health Organization (WHO) and the Joint Commission, formerly the Joint Commission for the Accreditation of Hospital Organizations (JCAHO). Efforts to delineate the frequency of complications related to anesthesia in patients undergoing congenital cardiac surgery and procedures in the cardiac catheterization laboratory and elsewhere have been difficult. This is due to the relatively low frequency of this surgery compared with other surgeries on children and the uncommon incidence of anesthesia‐related complications today. Busy cardiac anesthesia services at major North American pediatric institutions will each have contact with 2,000–3,000 congenital cardiac patients/year, and the majority of these cases are non‐surgical, such as diagnostic and therapeutic catheterizations and radiology procedures. The recognition of the need to systematically quantify and study outcomes in pediatric cardiac surgery, anesthesiology, cardiac critical care, and cardiology has led to the development of a variety of multi‐institutional and multinational efforts to systematically document and study this population. Educational content reviewed by this chapter will include: (i) A discussion of errors and outcomes in surgery and anesthesia, emphasizing communication and teamwork; (ii) A discussion of systems for prospective risk assessment in pediatric cardiac surgery; (iii) An analysis of closed malpractice claims in anesthesia focusing on pediatric cardiac anesthesia, morbidity, and mortality; and (iv) Database initiatives in congenital cardiac anesthesia, surgery, and interventional catheterization. The perioperative management of patients with congenital heart disease (CHD) is fraught and there are occasions when even small decision‐making errors can have catastrophic outcomes. James Reason has popularly described the “Swiss cheese model” for evaluating patient complications due to human errors [1]. For a complication to occur, all the “holes” in the cheese have to line up – that is, there is a sequential failure of various defense mechanisms in place to prevent, recognize, and/or treat unwanted physiologic changes (Figure 3.1). Many “anesthesia” complications are multifactorial in origin, and it can be difficult to assign the relative contributions of different clinical services. For example, failure to successfully separate from cardiopulmonary bypass may be due to issues such as technical difficulty (surgery and perfusion), residual lesions or bleeding (surgery), inotrope management or mistaken infusion pump programming (anesthesia and surgery), ventilator management (anesthesia), or underlying patient physiology such as intractable pulmonary hypertension (patient). These system factors are further exacerbated if there are communication difficulties between the various parties, including surgeons, anesthesiologists, perfusionists, cardiologists, and the pre‐ and post‐operative medical and nursing teams [2]. In a landmark study, de Leval and colleagues investigated the impact of human factors and teams on surgical outcomes in congenital cardiac patients, focusing on the neonatal arterial switch operation (ASO) as the prototype for complex, high‐risk surgery [3]. Patient and procedural data were collected on 243 operations performed by 21 cardiac surgeons in the United Kingdom in 16 centers over 18 months. Of these 243 patients, case study data were collected on 173 ASOs by two human factors researchers who followed each case from the time of induction of anesthesia until care was transferred to the intensive care team. The observed adverse events were subsequently divided into major and minor events depending on their impact on the safety of the patient. Analyses determined that, after adjustment for patient factors, the total number of minor and major events per case were both strong predictors of the probability of major morbidity or death or “near‐misses” (P < 0.001). The authors concluded that minor events go largely unnoticed by the OR team and are therefore left uncompensated. A subsequent examination of the same data suggests that minor events impede the OR team’s ability to compensate for future major events [4]. This investigation only included perioperative care and did not begin to address patient care prior to surgery or after handoff to the intensive care team, which would have added even more events into the calculations. In addition to the organizational factors, pediatric cardiac surgery procedures have a low error tolerance. The Bristol Royal Infirmary Inquiry and the Manitoba Inquiry reports both recognized the importance of human factors and systems research in improving pediatric cardiac surgical outcomes [5, 6]. The report of the Manitoba Pediatric Cardiac Surgery Inquest found that “serious organizational and personnel problems experienced by the Health Sciences Center’s Pediatric Cardiac Surgery Program during 1993 and throughout 1994 contributed to the deaths of these children.” Figure 3.1 The “Swiss cheese” model of accident causation. (Source: Reason [1]. Reproduced with permission of Cambridge University Press.) Galvan et al. published an observational study on complications in this complex patient population and recorded an average of 1.8 major compensated and nine minor compensated complications per case [7]. These complications were observed during the surgeries but were all recognized and treated by the medical team before injury resulted – all the “holes” in the Swiss cheese did not line up because one or more of the various systems in place to prevent patient injury worked appropriately. Barach et al. reported on a comprehensive process map in which they outlined the multiple steps involved in congenital cardiac anesthetic care and identified the potential sites for safety interventions [8]. They observed 108 open cardiac surgeries and found that communication failures were the most common underlying cause of major events. Examples of the organizational and human factors challenges that Barach et al. observed include: “Near‐misses” are important as they are 10–100 times more common than documented adverse events and yet share the same organizational and cognitive sources of error as major adverse events. Determining the frequency and nature of these events is potentially far more important than just looking at system failures resulting in patient injury, a process typically conducted in various quality improvement forums after the events. However, “near miss” analysis is difficult as it requires a trained, independent observer to accompany the patient through the entire care continuum – something that is clearly impractical except in very specific research settings. Outcome transparency has become a key component of programmatic evaluation. For example, news articles have either disclosed or triggered congenital cardiac surgical program closures possibly related to adverse patient outcomes compared with their cohorts, while other institutions have made the decision to publicly release their pediatric heart surgery outcome data on a regular basis in an attempt to provide the most accurate data possible and fend off any accusations of a program intentionally obscuring their outcomes [9–11]. Although there is currently no mandatory reporting for congenital cardiac surgical or anesthesia programs in the United States, the Society of Thoracic Surgeons (STS) provides “Star” ratings on its website for adult heart surgery outcomes in isolated coronary artery bypass grafting or aortic valve replacement operations [12]. One “Star” programs have risk‐adjusted outcomes greater than expected, two “Stars” have risk‐adjusted outcomes equal to expected, and three “Star” programs have outcomes better than expected based upon the procedure being performed and the patient’s underlying preoperative risk factors. Attempts to utilize this same “Star” system in the STS Congenital Heart Surgery Database (STS‐CHSD) have not been as successful because of a large number of factors relating to appropriate risk adjustments and the wide variety of case types in CHD. The STS‐CHSD has implemented a voluntary public reporting system that the vast majority of participants in the U.S. participate in. The STS‐CHSD public site reports 4‐year outcomes stratified by age grouping and adjusted for center case‐mix and complexity [13]. In Great Britain, congenital cardiac program mortality is reported publicly for all programs through the central cardiac audit database [14]. To date, there has been no similar effort to publish anesthesia morbidity and mortality, both because it is so infrequent and because of the absence of a national clearing house and the lack of risk adjustment. In complex environments like pediatric cardiac ORs or catheterization laboratories, errors cannot entirely be avoided. In his publication, Normal Accidents: Living With High‐risk Technologies, Perrow has postulated that even the best teams cannot eliminate every error [15]. What they can achieve at best is to prolong the time interval between errors. Therefore, it is of great importance for successful hospitals to create a culture of safety similar to the culture that the aviation industry promulgated to minimize the risk of human errors. More than a decade was required before hospitals were able to embed the concepts of checklists, team time‐outs and sign in/sign out procedures as components of daily safety procedures. In commercial aviation, one must realize that computers in modern airplanes have taken over the human function of checking lists. These computers are checking the lists themselves automatically because analysis of the human practice of using a checklist a hundred times reveals that this is a source of error in itself. The effort in the aviation industry now is focused more on team performance, simulation, and reducing errors due to hierarchy and authority issues. The six “Cs” – Communication, Cooperation, Coordination, Cognition (simulation and cross‐training), Conflict (managing disruptive behavior), and Coaching (team‐training) – are the key goals for successful working interdisciplinary teams. A comprehensive summary of this topic is presented by Wahr et al. [16]. Figure 3.2 Closed‐loop communication. (Source: Agency for Healthcare Research and Quality [18].) The Joint Commission and WHO have both advocated instituting a time‐out system prior to procedures to minimize the risk of preventable complications such as wrong‐site surgery and failure to administer antibiotics in a timely manner [17]. In addition, “closed‐loop” communication has been encouraged to minimize system errors (Figure 3.2) [18]. This particular technique is very helpful in the OR environment, where there can be a wide variety of distractions from background noise, music, cellular telephones, computers, monitors, conversations, and alarms. Shaw and colleagues reviewed much of this literature in their chapter, “Operating room safety, communication and teamwork” in Gregory’s Pediatric Anesthesia [19]. There are a variety of additional techniques to maximize communication in the OR (or any other procedural location) as well as documenting the flow patterns that may lead to adverse events [17]. Pediatric and congenital cardiac care databases are among the most commonly utilized multicenter areas of study and are in place worldwide in surgery, cardiology, anesthesia, critical care medicine, hospital‐based care, outpatient care, echocardiography, and more [20]. These take the form of either clinical datasets, administrative datasets, or a combination of the two. Many, if not most, of the databases have settled upon a universal set of terms to define the spectrum of congenital heart defects and repairs, the International Paediatric and Congenital Cardiac Code (IPCCC) [21]. The IPCCC has been widely adopted and is now incorporated into the World Health Organization’s International Classification of Diseases (ICD), version 11 [22]. In order to better quantify both the incidence of adverse events and the outcomes of surgical procedures, the Society of Thoracic Surgeons’ database committee established a nationwide (and now international) voluntary and anonymized registry of congenital cardiac cases and outcomes in the 1990s [23]. This includes almost every congenital cardiac center in the United States and three in Canada. As of the Spring 2019 report, the STS Congenital Heart Surgery Database (STS‐CHSD) held information on over 515,659 patient surgical procedures. The European Association for Cardio‐Thoracic Surgery (EACTS) has developed a transnational system for congenital cardiac surgery throughout the European continent and the UK. The EACTS utilizes a shared nomenclature for both lesions and complications with the STS that allows pooling of the two datasets to create an even larger picture of worldwide congenital cardiac surgery and outcomes. Other databases in pediatric cardiac surgery worldwide either use the common STS‐EACTS nomenclature or similar tools. The STS‐CHSD serves as an important resource for determining United States outcomes on a given congenital cardiac lesion and benchmarks by which individual hospitals and surgeons can compare their results against aggregate results on a lesion‐by‐lesion, complexity, risk, and age‐adjusted basis. Both public and private payers have begun to incorporate these data in evaluating programs. The state of Florida, for example, has mandated participation in this type of database as a requirement for participation in state‐run insurance programs such as Medicaid [24]. In New York and Pennsylvania states, the Departments of Health publish outcomes data on every program in their respective states, including whether they fall above or below 95% confidence intervals for expected outcomes after risk adjustment [25]. The private health insurer, United Healthcare, has established “Centers of Excellence” to facilitate referrals within their systems and to maximize their patient outcomes and satisfaction while minimizing the added expense of complications [26, 27]. Demonstration of superior outcomes through benchmarking is one element of the requirements for consideration as a preferred referral center, and the popular US News and World Report Hospital and Specialty annual rankings include database participation and benchmarking in their ranking algorithm [28]. Other important efforts that have come out of the STS‐CHSD include working groups that have established the consensus guidelines for defining lesion nomenclature, morbidity, and mortality [29]. All of these efforts have been coordinated internationally with other groups such as the EACTS to allow the free flow of comparative data across national boundaries. Unlike many countries around the world that utilize a national insurance program and patient registry, the U.S. has not developed a patient identification system that accurately allows tracking of an individual throughout their lifetime and across both state and hospital boundaries. Utilization of something like a Social Security number is impractical due to privacy restrictions and, in the case of the Social Security number, availability at the time of admission, particularly for newborns or for international patients being treated within the United States. At this time, efforts to link the various congenital cardiac surgical and non‐surgical databases are relatively limited and rely upon post‐hoc linkage of various unique identifiers such as date and place of birth, patient gender at birth, and maternal name. Cardiac Networks United is a consortium of various specialty groups attempting to bridge this gap but its functionality is currently limited by the absence of surgical data from the STS‐CHSD [30]. Denmark might serve as a role model because the combined health registers with social registers on an individual level by a unique personal identification number called CPR which is used in all Danish national registers. In 2009, they established The National Centre for Register‐based Research [31]. This institution set up a register database in 2010 combining all available registers in Denmark open to researchers. Up to now, the database contains a detailed description of over 45 Danish registries [32]. One well‐recognized weakness of single‐site reporting systems is that a patient undergoing a procedure at one institution is completely separated from the same individual being cared for at a different institution subsequently, a circumstance which happens frequently with congenital heart care. In the case of an adverse event, the receiving facility becomes the center that reports the morbidity in their statistics, while the initial treating institution may not necessarily report the event. The STS‐CHSD is attempting to address this flaw by extending the mandatory follow‐up period from 30 days to 1‐year post‐procedure and through changes in the data collection form which reflect transfers and “salvage” care. The Congenital Cardiac Anesthesia Society (CCAS) was incorporated in 2005 and one of its first initiatives was to approach the STS about developing anesthesia information to be included in the STS dataset [33]. The first iteration of this collaboration went “live” in January 2010 and has been updated regularly since then. The CCAS work with the STS subsequently served as a model for the Society of Cardiovascular Anesthesiologists (SCA) Adult Cardiothoracic Anesthesia Database, which is now working in conjunction with the appropriate STS dataset. The adult SCA database that began entering patient data in 2013 tends to focus more on patient diagnostic and therapeutic elements rather than outcomes or adverse events [34]. The CCAS is now working with the American College of Cardiology’s Improving Pediatric and Adult Congenital Treatment (IMPACT) registry, which collects information about pediatric interventional cardiology and electrophysiology procedures, to include an expanded number of data elements relative to anesthesia including anesthesia type and anesthesia‐related adverse events in its next update. A major benefit of utilizing an annual data submission process across multiple institutions is that it allows for a more contemporaneous examination of patient outcomes. Publications from two centers with a long history of anesthesia data collection, the Boston Children’s Hospital and the Mayo Clinic, illustrate the difficulties with single‐center record‐keeping [35, 36]. Their data, critically important as it is, represents time periods ranging from 6 years (Boston Children’s Hospital) to 17 years (Mayo Clinic). During these time spans multiple factors may shift that significantly alter patient outcomes and potential complications. For example, personnel changes and experience (physician, nursing, and ancillary staff), surgical technique modifications, pharmacology, technical advances with better monitoring and equipment, and more sophisticated complication detection and tracking all impact patient outcome statistics. In examining low‐frequency events, the only way in which to accurately estimate their occurrence is to investigate large numbers of patients. No single center can provide sufficient patients as a denominator in a relatively short period of time; therefore, it is necessary to either lengthen the epoch studied (with the weaknesses mentioned earlier) or increase the denominator by expanding the patient base by making the data multi‐institutional, recognizing that by increasing the sites participating one may decrease the relative consistency and accuracy of the data collected. One goal of the STS–CCAS collaboration is specifically to do the latter. It is intuitive that different pediatric cardiac surgical procedures will have radically different long‐term outcomes related to the underlying severity of the defect(s) (including in‐utero effects on blood flow patterns), the complexity of the operative repair, and the co‐morbidities found in a given patient as well as non‐defect related elements such as patient socio‐economic factors, racial disparities, parental education levels and insurance status [37]. In order to best estimate these potential outcomes, initial efforts were made at developing risk categories based upon “best guess” techniques in which groups of cardiac surgeons and cardiologists sat down in a room and assigned each individual operative procedure to a risk category pool based upon their collective years of experience. The most widely adopted categorization schema utilizing this technique is the Risk Adjustment for Congenital Heart Surgery (RACHS‐1) scoring system developed by Jenkins and colleagues [38, 39]. The Aristotle Basic Complexity (ABC) score is another attempt at preoperative risk assessment utilizing expert consensus based upon values being assigned to three components: the potential for perioperative mortality, the potential for perioperative morbidity, and the technical difficulty of the proposed repair [40]. The ABC system expanded the number of procedures evaluated, as compared with the RACHS‐1 system, but the RACHS‐1 appears to better discriminate at predicting mortality when the two are compared against each other [41]. The weakness of both these systems is that they are based upon a consensus estimation of experts in the field. The STS‐CHSD, in conjunction with the EACTS, subsequently developed a risk model developed from empirical outcome data from two large databases, the Society of Thoracic Surgeons–European Association for Cardio‐Thoracic Surgery Congenital Heart Surgery (STAT) mortality score. The initial STAT mortality scores were modeled utilizing over 70,000 records from the two societies and the pediatric cardiac surgical procedures were divided into five levels of mortality categories (“strata”); however, these are based upon the observed outcomes rather than a priori assignment by expert opinion and this process was then validated by comparison to a larger sample set of over 111,000 pediatric cardiac surgical cases in both the STS and EACTS registries [42]. This STAT mortality score has recently been updated, incorporating more procedures and frequent combination procedures and updated based upon more current outcome data solely from the STS‐CHSD [43]. Each STAT mortality category was created so that the inter‐category differences were sufficient to warrant assignment to either a lesser or a greater score category. Table 3.1 contains a listing of the newest STAT‐assigned procedures or procedure combinations and their respective categories. The STAT, RACHS‐1, and ABC scores are all based upon in‐hospital mortality rates and do not fully account for either in‐hospital morbidity or out‐of‐hospital mortality, such as inter‐stage deaths that occur more than 30 days after the procedure or either hospital discharge, whichever is later. Another major component that is not fully accounted for in these systems is preoperative morbidity, such as prematurity, genetic abnormalities, or co‐existing diseases. Post‐operative mortality after hospital discharge, and residual surgical lesions are also not captured. Work is progressing on each of these issues as they relate to patient outcomes. Concerns within the STS‐CHSD related to differences in surgical outcomes based upon the patient’s underlying diagnosis have also led to ongoing research examining the impact of diagnosis‐procedural (D‐P) combinations. For example, the modified Blalock‐Taussig shunt palliative procedure may be performed for a very heterogeneous group of underlying anatomic diagnoses with inadequate or abnormal pulmonary artery blood flow. Ongoing research efforts using complex large machine‐learning models are currently underway to determine if these D‐P combinations impact patient mortality estimates differently than a purely procedure‐based model such as the current STS‐CHSD utilizes. This work has now shown that except for helping discriminate between very high risk procedures, the addition of diagnosis to the procedure did provide additional benefit in predicting post‐operative mortality [44, 45]. Pasquali et al. have drawn extensively from work done in adult cardiovascular surgery to determine the relevance of “failure to resuscitate” (FTR) as a marker for programmatic quality [46]. The authors start with the hypothesis that complications and morbidity will always occur in any complex system such as the surgical repair of congenital heart defects. A single‐site retrospective analysis by Agarwal and colleagues found an incidence of identified adverse events of 43% (126 of 325 patients); this was in a retrospective chart review, not in the type of prospective observational study performed years earlier by de Laval and Barach, which might identify more subtle minor events that occur without being recorded [3, 8, 47]. What distinguishes high‐performing programs from lesser‐performing ones is not the incidence of adverse events per se, but the response to them. Table 3.1 Society of Thoracic Surgeons – European Association for Cardio‐Thoracic Surgery Congenital Heart Surgery (STAT) Mortality Score categories Source: Adapted from Jacobs et al. [43]. AICD, automated internal cardiac defibrillator; AP, aortopulmonary; ASD, atrial septal defect; ASO, arterial switch operation; AVC, atrioventricular canal; AVR, aortic valve replacement; ccTGA, congenitally corrected transposition of the great arteries; DCRV, double‐chambered right ventricle; DKS, Damus–Kaye–Stansel; DOLV, double outlet left ventricle; DORV, double outlet right ventricle; HLHS, hypoplastic left heart syndrome; IAA, interrupted aortic arch; LV, left ventricle; MAPCAs, major aortopulmonary collateral arteries; PA, pulmonary artery; PAPVC, partial anomalous pulmonary venous connection; PDA, patent ductus arteriosus; PFO, patent foramen ovale; PVR, pulmonary valve replacement; REV, reparation a l’étage ventriculaire (REV procedure); RV, right ventricle; RVOT, right ventricular outflow tract; TAPVC, total anomalous pulmonary venous connection; TCPC, total cavopulmonary connection; TOF, tetralogy of Fallot; TVR, tricuspid valve replacement; VSD, ventricular septal defect. Interventional cardiologists have also addressed predictive outcomes analysis. In 2007 the Congenital Cardiac Catheterization Project on Outcomes (C3PO) registry was created to establish standardized and comparable metrics across 15 pediatric cardiac catheterization laboratories, utilizing an internet‐based tool on all cardiac catheterization cases [48]. Post hoc analyses utilizing multivariate modeling then determined four discrete procedural risk categories based upon the age of the patient and the type of procedure being performed (diagnostic, valvuloplasty, device or coil closure, angioplasty, stent placement, stent redilation, and others). Adverse events were categorized on a scale of 1–5, with 1 being no adverse event noted, and 5 catastrophic (i.e. resulting in death or emergent surgical intervention to prevent death). The risk scoring method was called the Catheterization for Congenital Heart Disease Adjustment for Risk Method (CHARM). The purpose of the C3PO and CHARM project is to allow for appropriate risk adjustments to be made when comparing outcomes at a given center with expected outcomes for their specific patient population. Over a 2‐year‐period approximately 10,000 cases were analyzed to develop predictors of adverse events which included hemodynamic parameters such as cardiac index, right ventricular pressures, and the right ventricular to systemic pressure ratio [49]. The C3PO‐QI database evolved from this endeavor in 2013 to address quality improvement issues in the catheterization laboratory. This prospective data registry includes over 13,000 cases and aims to improve standards of care in pediatric cath labs, including initiatives on radiation exposure [50]. This is a critical part of the quality improvement process that must occur at every institution. Similarly, the IMPACT Registry was created in 2011 with the aim of creating risk stratification among common pediatric cath lab cases, and tracking outcomes comparing high versus low performing centers [51]. In order to achieve this, IMPACT created learning collaboration with the National Pediatric Cardiology‐Quality Improvement Collaborative (NPC‐QIC). The NPC‐QIC’s focus is to reduce themortality rate and improve the quality of life for small children with hypoplastic left heart syndrome in the interstage period between the Norwood and Glenn Procedures. The key drivers of this quality improvement initiative extend beyond the pediatric cath lab and include factors such as nutritional status, discharge coordination, and home medical surveillance. This quality improvement collaborative was able to demonstrate a 44% reduction in interstage mortality amongst the participating centers.
CHAPTER 3
Quality, Outcomes, and Databases in Congenital Cardiac Anesthesia
Introduction
Errors and outcomes in surgery and anesthesia
The six “Cs”: communication and teamwork
Databases in pediatric cardiac surgery and anesthesiology
Prospective risk assessment in pediatric cardiac surgery and cardiology
STS expected hospital discharge mortality rate (95% confidence interval)
Mortality category 1(0.20–1.33%)
Mortality category 2(1.43–2.94%)
Mortality category 3(3.01–6.82%)
Mortality category 4(6.88–13.00%)
Mortality category 5(13.45–38.68%)
Aortic stenosis, subvalvar
Valvuloplasty, mitral
Coarctation + VSD repair
Unifocalization, bilateral complete
ASO + VSD + aortic arch repair
Valve replacement, pulmonic
Valvuloplasty, Aortic – converted to AVR
Pulmonary artery aneurysm repair
TAPVC repair
Unifocalization, bilateral, incomplete
ASD repair, patch +PAPVC
Atrial fenestration closure
MAPCA unifocalization
ASD + aortic arch + VSD
Shunt, Potts’ type
ASD repair, Patch
Arrhythmia surgery, ventricular
Systemic venous stenosis repair
Ross–Konno
Valve replacement, common AV valve
AVC repair, intermediate
Annular enlargement procedure
ASD partial closure
Fontan revision or conversion
Truncus + interrupted aortic arch
ASD repair, primary
PA reconstruction, Branch, Central
ccTGA, VSD closure
Ross–Konno, valvuloplasty converted to aortic valve replacement
Norwood
Conduit reoperation
Valve closure, semilunar
DORV repair
Senning
Unifocalization MAPCAs + Central shunt
PAPVC, baffle (Warren)
Glenn (unidirectional)
MAPCA occlusion
TOF – AVC repair
Hybrid stage 1
AAOCA repair
Glenn (bidirectional)
Aortic arch repair
Valvuloplasty converted to a replacement, common AV valve
ASD partial closure + pulmonary venous repair
DCRV repair
PA band adjustment
Hepatic vein to azygous graft
Valve closure, tricuspid
Hybrid stage 1 + bilateral PA bands + PDA stent
Vascular ring repair
Arterial switch + aortic arch repair
TOF, RV‐PA conduit
Glenn + AV valvuloplasty
Ebstein’s repair + PDA closure
TOF, ventriculotomy, nontransannular
Shunt, ligation and takedown
Fontan, atrio‐pulmonary
PDA + modified BT shunt
Shunt, modified BT + DKS
Fontan, lateral tunnel, fenestrated
ASD + TOF repair, no ventriculotomy
ASD repair, common atrium
Yasui
Ross–Konno + valve replacement, mitral
PFO, primary
Mitral stenosis, supravalvar ring
Hybrid approach, transcardiac device placement
Aortic root replacement, homograft
Valvuloplasty, tricuspid + Norwood + RV‐PA conduit
VSD repair, patch
Conduit placement, RV to PA
Mustard procedure
VSD + ASD + PAPVC
PA Banding + valvuloplasty, common AV valve
PAPVC repair
PAPVC, Scimitar
Valvuloplasty converted to a replacement, Pulmonic
Cardiac tumor resection
Hybrid stage 1 + bilateral PA bands
TOF, no ventriculotomy
Coronary artery fistula ligation
Aortic root translocation
Pulmonary venous stenosis repair
TAPVC + shunt, systemic – pulmonic
Aortopexy
Anomalous coronary from the pulmonary artery
Coronary artery bypass
PA reconstruction (central) + modified BT shunt
TAPVC + shunt, systemic – pulmonic + PDA closure
Valvuloplasty, pulmonic
VSD creation/enlargement
Pericardial drainage procedure
Valvuloplasty converted to a replacement, tricuspid valve
Ross procedure
LV to aorta tunnel repair
Valve replacement, aortic, homograft
Central shunt
AVC repair, partial
PDA closure, surgical
TOF, ventriculotomy, transannular patch + vascular ring repair
Shunt, reoperation
Pacemaker procedure
Pulmonary atresia‐VSD repair s/p unifocalization
Transplant, heart
PA banding
VSD repair, primary
ASD repair, device
Valve replacement, mitral
Central shunt, Mee type
Aortic root replacement, bioprosthetic
Bilateral bidirectional Glenn
Valvuloplasty converted to replacement, mitral
PA reconstruction + heart transplant
RVOT procedure
ASD + PV replacement
Interrupted aortic arch repair
Transplant, lungs
Valve replacement, Aortic
ASD + PAPVC
Valvuloplasty, aortic + valvuloplasty, mitral
Aortic dissection repair
Valvuloplasty, Tricuspid
VSD repair + conduit
VSD patch + AVC repair
Truncus arteriosus repair
AS repair, subvalvar with myectomy
PA reconstruction, Branch, peripheral
Coarctation, subclavian flap
ASD + pulmonary atresia/VSD repair, including TOF pulm atresia
Valvuloplasty, aortic
VSD multiple, repair
Ventricular septation
Unifocalization + conduit placement
Pulmonary sling repair
Glenn + PA plasty
Ventricular septum fenestration
TOF – absent PV
Sinus of Valsalva aneurysm repair
VSD + valve replacement, pulmonic
REV
HLHS biventricular repair
PA reconstruction, main trunk
Conduit placement, Ventricle to aorta
Pulmonary embolectomy, chronic
Conduit, RV – PA + aortic root replacement
Valvuloplasty, Truncal valve
Removal of transcatheter delivered device from heart
Valve replacement, Tricuspid
AVC repair, complete + coarctation end‐end
Rastelli procedure
Batista procedure
Arterial switch + VSD
RV – PA conduit + PDA closure
Explantation of pacemaker
Valvuloplasty, common AVV
Unifocalization, MAPCAs
Hybrid stage 2 + aortic arch repair
Coarctation repair, end‐to‐end
PA debanding
Fontan, external, hepatic veins to PA, fenestrated
Pulmonary artery sling + tracheal repair
Aortic aneurysm repair
Anomalous systemic venous connection
Tricuspid valve excision without replacement
Hemi‐Fontan
VSD repair, device
Hybrid stage 2 without aortic arch repair
ASD, primary + VSD repair, patch
Arterial switch operation
Fontan, external conduit, fenestrated + pacemaker
Aortic root replacement, valve sparing
Valve excision, pulmonary
Fontan, external, hepatic veins to PA, nonfenestrated
Coarctation repair, patch
Acute pulmonary embolectomy
Hybrid, transcardiac
Valve replacement, Aortic, mechanical
Ligation, pulmonary artery
Damus‐Kaye‐Stansel
1 1/2 Ventricular repair
Sternotomy wound drainage
ccTGA, atrial switch + Rastelli
AICD Implantation
VSD – MAPCA, single stage repair
Shunt, modified BT
Coarctation repair, interposition graft
Atrial baffle procedure
AVC + vascular Ring
Arrhythmia surgery
Pericardiectomy
Fontan, external conduit, nonfenestrated
Aortic stenosis repair, supravalvar
Aneurysm repair, right ventricle
ccTGA, double switch repair
Pacemaker implantation, permanent
cc TGA, LV to PA conduit
Fontan + AV Valve repair
DOLV repair
Hemitruncus repair
Pulmonary AV fistula repair
Fontan, external conduit, fenestrated
Conduit reoperation + AVR
Kawashima operation
Pulmonary atresia – VSD repair
Cor triatratum repair
Fontan, intra/extracardiac repair, nonfenestrated
AP window repair
Cardiac tumor resection
Fontan, intra/extracardiac, fenestrated
Aortic arch + VSD repair
Valve replacement, truncal
Fontan takedown
Glenn takedown
Vascular stent removal
ASD creation/enlargement
Konno procedure
Aneurysm repair, LV
Ebstein’s repair
Conduit, LV to PA
Atrial baffle, Mustard or Senning
Pulm valve replacement + pacing explant
Pulmonary atresia – VSD MAPCA s/p unifocalization
VSD + PAPVC

Full access? Get Clinical Tree
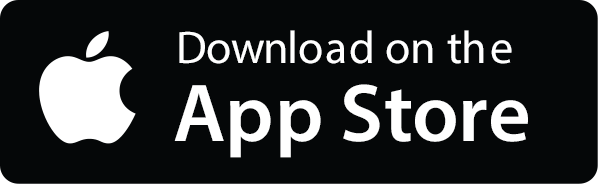
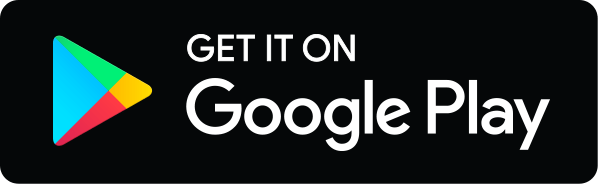