The Lion incubator was invented in 1891, but its approval for use in hospitals lagged behind. The first public use of an incubator for the care of an infant occurred in a side show of the Dreamland amusement park on Coney Island, NY and warmed a set of triplets born early to the owners of the show. Herein lies the birth of the specialized care of the premature infant in a world of modern medicine and technology [1]. A series of advancements, including portable incubators, ventilators, and antibiotics eventually led to the creation of the first official neonatal intensive care unit (NICU) at Vanderbilt University in 1961, when a ventilator was used (off-label) for the first time to help a struggling neonate.
While neonatology and pediatric anesthesia may not intuitively seem the closest of medical cousins, their developments have both relied heavily on technological advancements in order to care for ever sicker and more precarious neonates. The care of premature infants has continually fallen squarely into the hands of these two divisions, and an understanding of one another is paramount to the optimal care of patients in this unique population. The collaboration between the two groups is most notably recognized in the personage of Virginia Apgar (Figure 3.1), an anesthesiologist who developed the APGAR scoring system in 1952, to objectively assess the overall status of a newborn during the first moments of transition from fetal life [2].
Figure 3.1 Virginia Apgar, MD.
Population of the NICU
Patients
The National Perinatal Information System collects data on admissions to special care nurseries. “Special care” nurseries (SCNs) are defined as either NICUs or neonatal intermediate care units (NINTs), and include data on children born at hospitals with these units on site as well as children transported into these units. These data are prepared by the March of Dimes into a report titled “Special Care Nursery Admissions.” The most salient statistics include the following regarding infants born during the study period (July 1, 2009 to June 30, 2010):
14.4 percent of all infants were admitted to an SCN;
49.1 percent of admissions were preterm (defined as <37 weeks gestational age [GA]);
70 percent of these premature infants were admitted to the NICU, not NINT;
23.6 percent of infants admitted to the SCN had a primary diagnosis of “prematurity”;
11.9 percent of infants admitted to the SCN had a diagnosis of “respiratory distress syndrome”;
90 percent of infants born less than 34 weeks GA were admitted to an SCN [3].
Providers
As in other intensive care environments, the providers in the NICU are highly trained and varied in skillsets. The physician specialist associated with the NICU is the neonatologist (a pediatrician with subspecialty training in neonatology), though many NINTs are immediately staffed by pediatricians with neonatologist supervision. Other direct providers include neonatal nurse practitioners as well as neonatal clinical nurse specialists. In addition to these, and depending on the hospital environment, there may also be neonatology fellows and pediatric residents caring for the neonates. The success of an NICU depends on the collaboration of multiple providers working together as a team. Members of the team often include respiratory therapists, physical therapists, occupational therapists, speech therapists, registered dietitians, lactation consultants, pharmacists, and social workers.
Common Medical Issues in the NICU by Systems
The NICU population is unique in the medical system, and their problems and diagnoses are equally unusual. While other chapters in this book will look more exhaustively at each system described below, it is prudent in a discussion of the NICU to address the major conditions, abnormalities, and diagnoses found in the average NICU. We will address these conditions by systems.
Neurologic
Neurodevelopment is a process that is ongoing throughout childhood and adolescence, but it is particularly dynamic in the neonate, and especially so in the preterm neonate. A more detailed review of neonatal response to pain, development, and drug toxicity can be found in other chapters of this book. Below, we briefly discuss the major neurologic conditions often uniquely found in the neonatal population: intraventricular hemorrhage (IVH), hypoxic-ischemic encephalopathy (HIE), retinopathy of prematurity (ROP), and the neonatal abstinence syndrome (NAS).
Intraventricular Hemorrhage
The neonatal brain is a rapidly developing organ, with neuronal migration originating in the highly vascularized germinal matrix, which is a particularly delicate region, exquisitely sensitive to physiologic perturbations. With such changes, bleeding can develop within the germinal matrix; staging of IVH is based on the degree of blood spread into the periventricular parenchyma and adjacent lateral ventricles. Smaller infants are more susceptible to hemorrhage, especially within the first few days of life and since the presentation is often subtle, screening head ultrasounds have become routine for low birth weight neonates in most NICUs [4].
Hypoxic-Ischemic Encephalopathy
While HIE remains a relatively rare occurrence in neonates (1–3 per 1000 live full-term births), the morbidity and mortality remains high. Fifteen to twenty percent of infants with HIE will die during the postnatal course and another 25 percent will develop significant and permanent neurologic sequelae, such as cerebral palsy, neurodevelopment impairment, and epilepsy. Currently there is much research into neuroprotective strategies that can be employed following a hypoxic insult. The only proven strategy is that of hypothermia (cooling the infant by 3–5 °C), although many other strategies (e.g., free radical scavengers and erythropoetin) are being investigated [5].
Retinopathy of Prematurity
Premature infants are born with incompletely vascularized retinas. When subsequent vascularization does not proceed normally, ROP develops, which if left untreated could lead to retinal detachment and blindness. ROP generally occurs in two phases, with retardation in vessel growth occurring first at around 30–32 weeks post-GA and then a proliferative phase occurring at approximately 32–34 weeks post-GA. The most recognized risk factors are prematurity, low birth weight, and hyperoxygenation (including the use of unnecessary supplemental oxygen in the operating room), although infants exposed to very little supplemental oxygen have developed ROP. Improved screening, early diagnosis, and treatment (with bevacizumab [an angiogenesis inhibitor] or LASER treatment by an ophthalmologist) have improved the overall morbidity, despite the fact that the overall incidence of ROP has not declined [6].
Neonatal Abstinence Syndrome
As the number of people dependent on opiates (both prescribed and illicit) and other psychotropic medications has increased in the last few decades, so has the incidence of intrauterine exposure and the number of infants born dependent on these same substances. The signs and symptoms displayed by neonates as they withdraw from these medications are collectively referred to as the “neonatal abstinence syndrome” or NAS. The timing of first symptoms is dependent on the type and dosage of the exposed medication, with heroin and oxycodone withdrawal occurring rapidly and methadone and buprenorphine withdrawal sometimes not occurring for several days. Treatment generally consists of the controlled replacement and weaning of opiate (usually morphine or methadone), with or without adjuncts such as phenobarbital or clonidine, while carefully monitoring for signs of withdrawal. Various scoring systems and weaning strategies are used, and are quite unit-specific. Many units are utilizing nonpharmacologic management techniques such as occupational and massage therapy [7].
Cardiac
During fetal life, roughly 90 percent of combined ventricular output is directed toward the systemic circulation, with only 10 percent entering the pulmonary vasculature. This feat is accomplished mainly via two shunts: the intracardiac foramen ovale and the extracardiac ductus arteriosus; these two shunts close postnatally as the infant’s pulmonary vascular resistance falls and the infant transitions away from the fetal circulation. Excluding congenital heart disease (CHD) and the hypotension associated with sepsis, the bulk of cardiac pathology in the NICU concerns an abnormal transition to postnatal circulation.
Patent Ductus Arteriosus
The ductus arteriosus is a large conduit that links the fetal main pulmonary artery to the descending aorta, which usually closes early in postnatal life as oxygen tension increases and prostaglandin input decreases. Its closure is usually welcome, except in the case of so-called ductal-dependent cardiac lesions, but it often remains open in preterm neonates, leading to the unwanted shunting of blood. In fact, in infants born at <28 weeks GA, a majority (60–70 percent) will require either medical or surgical closure of a patent ductus arteriosus (PDA). There is considerable research into when a PDA is significant enough to warrant intervention, but two of the most important considerations are those of diastolic flow reversal and cerebral steal and pulmonary overcirculation. Once the decision has been made to intervene, a trial of medical closure is generally attempted, using cyclooxygenase inhibitors like indomethacin or ibuprofen. If medical treatment is unsuccessful or inappropriate, a surgical PDA ligation can be performed via a left thoracic approach [8].
Persistent Pulmonary Hypertension of the Newborn
As its name implies, persistent pulmonary hypertension of the newborn (PPHN) occurs when the normal transition from fetal to postnatal circulation does not occur. Normally, shortly after birth, the systemic vascular resistance increases (due largely to the clamping of the umbilical cord), the pulmonary vascular resistance drops precipitously (due to multiple factors), and the major fetal shunts (the ductus arteriosus and foramen ovale) experience a functional followed by anatomical closure. When this drop in pulmonary vascular resistance does not occur, the closure of the shunts cannot occur, and the neonate will remain in a fetal circulation characterized by a circuit in parallel instead of a mature circuit in series. In other words, if a neonate has PPHN, shunting and variable systemic oxygen saturation and perfusion can result. The causes of PPHN are varied, but are generally due to abnormal parenchyma with resultant vasoconstriction (e.g., meconium aspiration syndrome, respiratory distress syndrome, pneumonia), abnormally deficient vasculature (e.g., pulmonary stenosis, lung hypoplasia), or are idiopathic. Treatment focuses on overall physiologic stability and efforts to decrease pulmonary vascular resistance through interventions such as inhaled nitric oxide, phosphodiesterase inhibitors, sedation, and methods to optimize oxygenation. When conventional therapies are unsuccessful, extra corporeal life support (ECLS) is offered to a select population [9].
Congenital Heart Disease
An exhaustive discussion of CHD is beyond the scope of this section. We will instead focus on just those conditions that will present within the first few hours or days of life. The immediate neonatal period is marked by a transition from fetal circulation to adult circulation and can often bring to light major congenital cardiac lesions. Certain lesions, such as total anomalous pulmonary venous return and critical pulmonary stenosis, are not even apparent on fetal surveys due to the significantly decreased pulmonary blood flow in the fetus. Other lesions, such as hypoplastic left heart syndrome and transposition of the great arteries, require a functional ductus arteriosus in order to supply oxygenated blood to the systemic circulation. In ductal-dependent cardiac lesions (see Table 3.1), an infusion of prostaglandin E1 can maintain patency of the ductus arteriosus and even open a functionally closed ductus arteriosus if anatomical closure has not occurred. Airway control is an important consideration when using prostaglandins, given associated apnea.
Table 3.1 Ductal-dependent cardiac lesions
• Critical coarctation of the aorta (decreased systemic perfusion) |
• Interrupted aortic arch (decreased systemic perfusion) |
• Critical aortic stenosis (decreased systemic perfusion) |
• Hypoplastic left heart syndrome (decreased systemic perfusion) |
• Tricuspid atresia (decreased pulmonary perfusion) |
• Pulmonary atresia (decreased pulmonary perfusion) |
• Transposition of the great vessels (inability to deliver oxygenated blood to the systemic circulation) [10] |
Pulmonary
Lung immaturity is a predictable biochemical, physiologic, and anatomical phenomenon in premature infants, and this unique situation has led to the development of a different set of treatment and ventilation strategies than in adults and children.
The threshold of viability is accepted by many to occur at approximately 24 weeks GA, and this time corresponds with the embryological development of respiratory bronchioles and alveolar ducts and signifies the initiation of the ability to oxygenate and ventilate. At approximately 26 weeks GA, type II pneumocytes begin to populate the alveoli and begin the production of surfactant, which will ultimately prevent the collapse of alveoli with exhalation and dramatically improve pulmonary compliance [11].
Antenatal steroids are a mainstay in the treatment of women with preterm labor (less than 32–34 weeks GA) because of the known effect on fetal lung maturation and surfactant expression. The efficacy of antenatal steroids has been strongly validated, with multiple meta-analyses demonstrating a clear benefit [12].
One aspect of neonatal ventilation often not seen in older children is the relative immaturity of respiratory control that can lead to irregular breathing patterns and even life-threatening apnea. This is multifactorial, with causes ranging from altered response curves to hypoxia and hypercapnea, to immature brainstem and peripheral receptor networks. Furthermore, neonates do not seem to be able to handle an oxidant stress and are particularly vulnerable to oxygen toxicity. In fact, the 2010 American Heart Association Guidelines for neonatal resuscitation recommend initiating resuscitation for term infants with room air and blending in oxygen as needed to achieve an acceptable oxygen saturation [13].
Respiratory Distress Syndrome
Respiratory distress syndrome (RDS), previously known as hyaline membrane disease, is best understood as difficulty ventilating due to the lack of surfactant and subsequent decrease in lung compliance and propensity for alveolar collapse. It is most commonly observed in premature neonates due to their natural surfactant-deficient state, but can be observed in full-term neonates whose surfactant, while present, might be inactivated by a variety of causes. Treatment depends on the degree of distress and prematurity and can include exogenous surfactant administration, noninvasive ventilatory support, and invasive ventilation [14].
Chronic Lung Disease and Bronchopulmonary Dysplasia
Chronic lung disease (CLD) is a fairly generic term that refers to pulmonary disease that follows respiratory disorders of the neonate. Bronchopulmonary dysplasia (BPD) is a form of CLD with a specific definition: The need for supplemental oxygen at least 28 days after birth or the need for supplemental oxygen after 36 weeks corrected GA, depending on the definition being used. As one can imagine, this does not have a high predictive value for long-term respiratory dysfunction, as it does not take into account GA at birth or oxygen delivery method (e.g., nasal cannula versus endotracheal intubation). Bronchopulmonary dysplasia is a condition of prematurity, almost always occurring in infants born at less than 30 weeks GA and with low birth weight (under 1500 g). As improvements have been made to the treatment of RDS, fewer infants have developed BPD characterized by non-homogeneous parenchymal damage due to ventilator-associated trauma. Instead, as smaller and younger infants are now surviving, infants are now developing a more homogeneous BPD characterized by the reduced development of alveoli. Treatment is centered first on prevention. Frequent strategies employed may include the occasional use of diuretics to improve respiratory mechanics, optimizing nutrition, palivizumab for respiratory syncitial virus prophylaxis, short courses of steroids for exacerbations outside of the neonatal period, and inhaled bronchodilators for obstructive exacerbations [15,16].
Transient Tachypnea of the Newborn
As discussed in the section on cardiac physiology of the neonate, the newborn infant must undergo a dramatic transition in order to move from a liquid environment reliant on maternal ventilation and oxygenation to one where ventilation and oxygenation are paramount to survival. The fetal lung is filled with fetal lung fluid up to a volume that is close to what will ultimately become the functional residual capacity. A small portion of this fluid is expelled due to external forces during labor, but the majority is reabsorbed by the lung epithelium once labor commences and levels of epinephrine increase. This is likely the reason that infants born via cesarean section without labor have a significantly increased incidence of transient tachypnea of the newborn (TTN) compared to those born by cesarean section after the beginning of labor [17]. When this fluid is not reabsorbed quickly enough, retained lung fluid will cause the infant to display symptoms of tachypnea, grunting, retractions, and variable hypoxia. This is usually a self-limited condition that resolves within 2–3 days [18].
Ventilation Modes in the NICU
Given the physiologic challenge that incompletely developed neonatal lungs present, it is not surprising that neonatal ventilation strategies is an area of active research. There are multiple modes of noninvasive (i.e., without an endotracheal tube) as well as multiple modes of invasive ventilation utilizing endotracheal intubation. Because of the very small tidal volumes in the range of 4–6 ml kg–1 (sometimes less than 5 ml in extremely low birth weight infants), the ventilators and circuitry have to operate in a precise manner with highly sensitive flow and pressure sensors [19].
In recent years, controversy has emerged regarding the use of cuffed versus uncuffed endotracheal tubes. In the past, the thought was to not use cuffed tubes in children younger than eight years because of the natural narrowing at the level of the cricoid cartilage, and because of the potential morbidity of subglottic trauma being caused by the cuff. With newer, lower profiled cuffs, this does not seem to be as much of an issue, and many practitioners are moving toward cuffed endotracheal tubes for neonates. The advantages of a cuffed tube include a good fit with fewer intubations, as well as a good seal with a minimized leak. Despite this movement, subglottic edema and remodeling continues to be a major concern of neonatologists and many units continue to use uncuffed endotracheal tubes as their standard of care [20].
Noninvasive Modes
Nasal continuous positive airway pressure (NCPAP) has been well established as a method of noninvasive airway support in neonates, often used following extubation or in the attempt to prevent the need for endotracheal intubation. Recently, newer methods have developed using sensitive triggers and processors that can provide more advanced and timed support. Synchronized noninvasive positive pressure ventilation (SNIPPV) and non-synchronized noninvasive positive pressure ventilation (nsNIPPV) have also been studied. There is a Cochrane Database review showing a benefit of NIPPV over simple NCPAP for success in extubation when used as a bridge [21].
Finally, high-flow nasal cannula can deliver a small degree of CPAP and improvement in oxygenation, but the flow must always be humidified as this modality can quickly lead to significant mucosal drying and bleeding as well as a surprising degree of dehydration [22].
Invasive Modes
Once the decision has been made to provide invasive ventilation utilizing endotracheal intubation, there are a wide variety of ventilation options that can be utilized, with the ultimate goal of preventing damage to premature, underdeveloped lungs when exposed to prolonged positive pressure ventilation. Generally, the first choice ventilation strategy is the use of conventional ventilation, but often this proves difficult due to either the need for high inspiratory pressures, hypoxemia, or both. When this modality is not adequate, the two main alternatives are high-frequency oscillatory ventilation (HFOV) and high-frequency jet ventilation (HFJV).
A recent meta-analysis in Neonatology investigated the use of conventional ventilation (CV) versus HFOV, specifically looking at short-term mortality, chronic lung disease, and other forms of morbidity. They found no change in short-term (~30-day) mortality. They found more pulmonary air leaks with HFOV as well as increased rates of significant IVH with certain modes of HFOV, although this particular finding was not consistent. There was, however, a decrease in the risk of ROP in the HFOV group [23].
Conventional Ventilation
Conventional ventilation in neonates is not terribly different than CV in adults. Many of the same principles are utilized, such as lung-protective strategies and basic initial settings. One point that should be remembered is that lung-protective measures, such as low tidal volumes and peak inspiratory pressures, should be utilized on all premature neonates.
A recent meta-analysis suggests that using volume-targeted ventilation (as opposed to pressure-targeted ventilation) leads to decreased days on the ventilator, BPD, and significant IVH (grade 3 or 4) [24].
Time-cycled, pressure-limited ventilation (TCPL) is often utilized in neonates and is also known as intermittent positive pressure ventilation (IPPV). In this mode, the inspiratory and expiratory times are set, allowing many variables to affect the tidal volume with each breath, while providing a continuous background flow and limited pressure [20].
High-Frequency Oscillatory Ventilation
Instead of utilizing conventional ventilation to provide close to physiologic tidal volumes using large pressure differences, HFOV relies on a completely different strategy. HFOV starts by providing a constant distending pressure (mean airway pressure, or MAP) about which a rapidly oscillating pressure difference, or amplitude, occurs. In fact, this occurs so rapidly that the oscillating pressure wave is attenuated and the alveoli ultimately are exposed only to the MAP, or a very small pressure perturbation. This is thought to decrease the sheer stress, volutrauma, and barotrauma caused by large changes in pressure and distention experienced by alveoli during CV. Also, given the higher MAPs tolerated during HFOV, oxygenation is often vastly improved. Because the pressure difference is created by a piston driving an oscillating membrane back and forth to create the pressure change around the MAP, this is the only ventilation mode that utilizes an active expiratory phase.
With minimal changes in pressure seen by alveoli during HFOV, it is often not intuitive how gas exchange is accomplished. Gas exchange during HFOV seems to be accomplished via a variety of mechanisms, instead of the fairly simple bulk gas dispersion utilized by CV. A paper in Critical Care Medicine in 2005 sought to clarify these mechanisms, which include but are not limited to: molecular diffusion, turbulence, pendelluft, and laminar flow with Taylor dispersion [25].
The basic initial settings for HFOV involve setting a MAP, an amplitude (change in pressure around the MAP), a frequency (usually 8–15 Hz), and an FiO2. Unlike in conventional ventilation, where oxygenation and ventilation are somewhat coupled, when utilizing HFOV, oxygenation and ventilation are mostly determined by different variables. The MAP and FiO2 are the major determinants of oxygenation and the frequency and amplitude will largely determine the ventilation (with lower frequencies leading to increased ventilation and CO2 elimination). It is important to appreciate a “wiggle,” which is the subjective vibratory movement of the chest wall that occurs when there is an adequate pressure difference about the MAP. This is in fact how the amplitude is titrated to effect in neonatal HFOV. High-frequency oscillatory ventilation utilizes active exhalation, which means a negative pressure is applied to force volume out of the lungs.
High-Frequency Jet Ventilation
High-frequency jet ventilation is another form of high-frequency ventilation, which uses a combination of conventional ventilation and high-frequency pulses. The general setup is to have a conventional ventilator deliver positive end-expiratory pressure (PEEP) as well as a low respiratory rate of physiologic tidal volumes referred to as “sigh breaths.” Coupled to this is a jet injector that pulses short and rapid jets of gas into the trachea at a rate of 240–660 breaths per minute, although the usual operating range is more in the range of 320–450 breaths per minute. These jets entrain additional gas by creating an area of negative pressure, but do not employ active exhalation as seen in HFOV.
A Cochrane Database review looked at the elective use of HFJV versus CV in the population of preterm infants with RDS and found that those babies treated with HFJV had a lower incidence of chronic lung disease, but there was an unclear impact on the incidence of IVH [26].
Gastrointestinal
The gastrointestinal system undergoes a complex developmental process to ready the fetus for enteral nutrition at birth. Despite this, a disproportionate amount of the oxygen delivery in a fetus is directed toward the head, leaving the gut vulnerable to ischemic insults in general. In addition to this, development does not always proceed perfectly and can lead to congenital defects noted at birth. For more in-depth and comprehensive information, please read the chapter on gastrointestinal disorders.
Necrotizing Enterocolitis
Necrotizing enterocolitis (NEC) is bowel necrosis that occurs postnatally and is a significant source of morbidity for small and preterm infants, often carrying high mortality rates. NEC will classically present in a neonate with a variety of often subtle signs and symptoms, including feeding intolerance, apnea/bradycardia/desaturation events, abdominal distention, discoloration of the abdomen, peritonitis, and bloody stools. Abdominal radiographs may reveal pneumatosis intestinalis, free air, and portal venous gas in cases of severe or advanced NEC. The pathophysiology is not fully understood, but likely involves a combination of an immature gut with a delicate vascular supply, abnormal microbial colonization, immunoreactive mucosa, and a genetic predisposition. Treatment depends on the severity of illness and whether or not an intestinal perforation has occurred. The mainstay of treatment regardless of severity is a combination of bowel rest and intravenous antibiotics. For more severe cases a peritoneal drain may be placed at the bedside or an exploratory laparotomy with bowel resection may be performed, either at the bedside or in the operating room [27].
Omphalocele and Gastroschisis
Omphalocele (Figure 3.2) and gastroschisis (Figure 3.3) are both congenital abdominal wall defects that must be addressed early in the neonatal course and are often diagnosed prenatally. Beyond the fact that significant evaporative losses can occur with both, the details most important to the anesthesiologist regarding these conditions are the associated comorbidities. Associated congenital abnormalities are far more common in patients with omphaloceles than those with gastroschisis. In fact, cardiac anomalies occur in 18–24 percent of infants with an omphalocele, making a preoperative (and often fetal) echocardiogram an essential part of the evaluation of an infant with an omphalocele. The bowel in gastroschisis, however, does not have the benefit of a protective sac, as in omphalocele, so patients with gastroschisis often have significant degrees of bowel inflammation and subsequent strictures. Depending on the size of the defects, they may require staged closures, allowing the abdominal cavity time to grow and accept the gut without significant diaphragmatic competition and respiratory compromise [28].
Figure 3.2 Gastroschisis.
Figure 3.3 Omphalocele.
Jaundice
Given the typical hematologic and hepatic state of the normal newborn, it is not surprising that neonatal jaundice occurs, to some degree, in every infant. Neonatal jaundice refers to an elevated level of indirect (or unconjugated) bilirubin that is manifest by the yellow discoloration of the skin in a level-dependent progression from the tip of the nose, down the body (the further caudad the spread, the higher the level). Left untreated, severe hyperbilirubinemia can lead to an acute bilirubin encephalopathy, also known as kernicterus. The factors leading to significant jaundice include the large erythrocyte volume, the faster turnover of erythrocytes, and the decreased activity of uridine diphosphate glucuronosyl transferase, which is responsible for the conjugation of bilirubin into a water-soluble form that can be excreted by the kidneys.
Often, phototherapy is utilized to provide conjugation via ultraviolet energy so that excess bilirubin can be conjugated and excreted (Figure 3.4). This condition is self-resolving once liver enzymes become upregulated and red cell turnover diminishes. ABO incompatibility (where maternal antibodies attack neonatal erythrocytes) is one of the more common situations leading to severe hemolysis and jaundice in the newborn. Routine screening and surveillance has significantly decreased the morbidity of this “setup” for jaundice [29].
Fluid Management in the NICU
Perhaps the first thing a newcomer to the NICU will notice regarding fluid management is that it is reported as ml kg–1 day–1 and referred to as “total fluids” (TF). To the inexperienced, the selection of TF can seem random or arbitrary, but is based on a set of guiding principles.
Extremely premature infants are composed of almost entirely water, with a composition of water nearing 90 percent. These premature infants actually lose much more water transepidermally than their more mature counterparts, and this loss can quickly lead to dehydration. In fact, they can lose more water this way than through urine output. Many of the newer incubators incorporate variable humidification to counteract these losses in the first few days of life. Luckily, after birth, infants rapidly attain mature degrees of transepidermal losses and this is less of a concern with time.
The choices of TF as well as glucose and electrolyte additives must be managed carefully with frequent adjustments, at least on a daily basis. Smaller and earlier gestational age infants will require more fluids on a ml kg–1 day–1 basis, and this will often increase over the first few days of life. Modi suggests that a good starting point for an infant born at less than 1000 g is 100 ml kg–1 day–1, making adjustments based on strict inputs and outputs as well as weight and electrolyte measurements [30].
As infants progress through their first few days of life, they have a natural diuresis as well as tremendous metabolic requirement needed for growth and their high basal metabolic rate. Because of this, infants will need more and more fluid as the days progress. O’Brien and Walker outlined general guidelines for low birth weight (LBW) (<2.5 kg), very low birth weight (VLBW) (<1.5 kg), and extremely low birth weight infants (ELBW) (<1 kg). They suggest starting with 50–60 ml kg–1 day–1 and 80–90 ml kg–1 day–1 for LBW and VLBW/ELBW infants, respectively, on day of life 0–1, and increasing progressively to 150–180 ml kg–1 day–1 by age five days, although many units stop at the lower end of this range [31].
Many NICUs will use mild fluid restriction as a strategy to decrease the incidence of PDA, NEC, BPD, and IVH. A Cochrane meta-analysis published in 2008 found a significant decrease in incidence of PDAs and NEC in premature infants and a trend toward a decreased incidence of BPD formation and IVH. There was, however, a greater weight loss in premature infants who were fluid restricted and many of these infants required higher-density caloric feeds in order to achieve adequate growth. In addition, many NICUs will also treat patients with BPD with diuretic therapy, which has been shown to be helpful in BPD exacerbations. Many of these infants will require electrolyte replacement, especially if they are treated with loop diuretics. Anesthetizing these infants is a challenge because of their relatively dehydrated state. Carefully crafting an anesthetic to minimize intraoperative hypotension in order to decrease the need for fluid boluses is helpful. Educating NICU providers about the cardiac depressant and hypotensive effects of most general anesthetics and the resultant need for fluid resuscitation is essential to avoid conflicts about the fluid management of these vulnerable patients in the operating room.
Temperature Regulation
For the lay public, there is probably nothing more synonymous with premature infants than the incubator. As mentioned in the introduction, the invention of the neonatal incubator was the first step in the modern care of the neonate and in reality had a tremendous impact on neonatal mortality, especially in infants born at less than 1500 g. The neonate is accustomed to being in a warm intrauterine environment that is generally just a little warmer than the mother (a point often noted if a neonatal temperature is taken immediately after birth). Just like their adult counterparts, neonates attempt to maintain their core temperature within a narrow range, but they have far less ability to do so and have a massively increased potential for heat loss in comparison. However, unlike their older counterparts, neonates do not have the capability to shiver in their attempt to increase their temperature – they instead rely solely on the metabolism of brown fat, which is present in the neonate as early as 25 weeks GA. This vulnerability to temperature applies also to hyperthermia. In short, neonates depend on a neutral thermal environment, which is the range of temperatures in which a neonate must expend minimal energy in order to maintain an acceptable core temperature.
Infants, and especially LBW infants, have a much higher surface area to volume ratio, and therefore can quickly lose heat to the environment via radiation, convection, and conduction. Immediately following birth, the most important mechanism of heat loss is via evaporation – the newborn emerges wet and must be quickly dried to prevent these losses. In addition to this, the skin of a premature neonate is thin, with very little subcutaneous fat, both of which decrease the neonate’s insulating capacity. Small preterm infants are such poor insulators that simple measures such as placing the infant in a plastic bag or wrapping it in plastic wrap can greatly decrease heat loss.
As the infant’s temperature falls below 36°C, active rewarming should be initiated to prevent further drops in temperature. However, when the temperature falls below 32°C, one may anticipate severe metabolic, cardiac, and neurologic derangements and corrective actions should be taken immediately [32].
Neonatal Sepsis
Sepsis has been and continues to be a major source of morbidity and mortality for neonates, especially preterm neonates. Its presentation can be as overt or subtle as the presentation for NEC, and treatment must proceed immediately to prevent further insult or death. In fact, newborns will often react to bacteremia and sepsis with hypothermia, instead of pyrexia. Because neonatal sepsis can present in subtle ways and have devastating consequences, infants will often complete “rule-out sepsis” evaluations in which antibiotics are administered until surveillance body fluid cultures return negative at 48–72 hours. In addition, several biomarkers are gaining popularity to guide antibiotic treatment, including C-reactive protein and procalcitonin.
The organisms most commonly responsible for early-onset neonatal sepsis include, but are not limited to, group-B Streptococcus (GBS), Escherichia coli, Listeria, and Enterococcus. GBS infection was a significant pathogen in early neonatal sepsis that has been dramatically reduced with routine maternal GBS screening and treatment. GBS remains a concern in infants of inadequately treated mothers and later in infancy. Several neonatal sepsis evaluation algorithms exist, but most institutions in the United States utilize the guidelines distributed by the American Academy of Pediatrics in the Red Book [33].
Common Surgical Procedures in the NICU
The major surgical interventions for neonates are covered in great detail throughout this book. In this section, however, we will focus only on those procedures commonly performed in the NICU at the bedside. The procedure location is highly hospital-dependent, with some institutions opting to perform the majority of procedures within the NICU and others opting to transport patients to the operating room for the majority of procedures.
NEC Resection, Peritoneal Drain Placement
Exploratory laparotomies, intestinal resections, anastomosis, ostomy creations, and peritoneal drain placements are common in the NICU and, in fact, are the most common emergency surgical procedures performed on the neonatal population. It is more common among LBW and premature infants and presents in a myriad of subtle and overt ways. The choice of surgical procedure is highly dependent on the acuity of the infant, but it is well-recognized that infants requiring surgery of any kind have much higher morbidity and mortality [34].

Full access? Get Clinical Tree
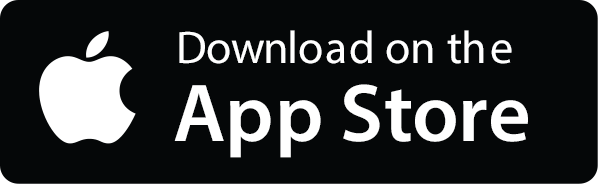
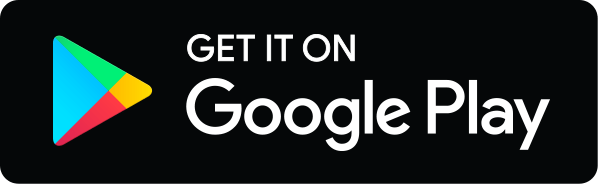
