Prenatal and Neonatal Diagnosis
With the improvement in echocardiographic technology, many children with congenital heart disease (CHD) can be diagnosed prenatally. In general, an anatomic ultrasound is performed at 18–20 weeks’ gestation. In the United States, prenatal detection rates of >50 percent have been reported for single ventricle lesions [1]. However, for two-ventricle lesions, the detection rate is usually less than 30 percent due to variations in quality of prenatal ultrasounds and access to prenatal care. Maternal and fetal risk factors such as first-degree relatives with CHD; in vitro fertilization; and chromosomal anomalies, extracardiac anomalies, or severe polyhydramnios may trigger fetal echocardiography to prenatally diagnose CHD [2].
Physical examination and pulse oximetry may be used to screen for CHD in the neonatal period [1]. In a recent meta-analysis, the overall sensitivity of pulse oximetry for detection of a critical heart defect was 76.5 percent (95 percent CI 57.7–83.5 percent) [3]. Despite advances in technology, some children will still present with new murmur, respiratory distress, decreased or absent peripheral pulses, or shock in the days and weeks following birth.
Neonates or infants who have been diagnosed with CHD should be reviewed with other tests, including an electrocardiogram for rhythm and a chest radiograph for the size and shape of the heart and the presence of pulmonary edema. Echocardiography is often performed in an unsedated neonate or infant; however, anesthesiologists may also be asked to provide sedation for a more thorough or complete transthoracic evaluation or for a transesophageal echocardiogram (TEE). Cardiac catheterization may be needed for diagnostic or therapeutic indications. A computed topography (CT) scan is often performed in infants in whom vascular anomalies may be present and may be contributing to airway compression. Cardiac magnetic resonance imaging (CMRI) is becoming more common, as it provides important information regarding vascular anomalies, as well as precise measurements of left ventricular and right ventricular size. Several of these diagnostic tests will require anesthesia, and many will require intubation due to one or more of patient factors, the length of the procedure, or the requirement for breath holds to attain adequate images.
Basic Principles of Management
It is important to recognize that despite the complexity of many congenital cardiac lesions, basic anesthetic principles remain a priority. Experienced congenital cardiac anesthesiologists are very much aware that the loss of an airway is still a major cause of morbidity or mortality in these infants. There are some general principles of anesthesia for neonates and infants with CHD that should be considered, although the specifics of the anesthetic plan are dictated by both the specific type of CHD and the severity of the disease. The patient’s condition should be optimized as much as possible prior to proceeding with anesthesia. In general, diuretics are held on the morning of operation, while beta blockade, antiarrhythmics, and pulmonary antihypertensive agents are continued. The risks and benefits of continuing or stopping anticoagulation such as warfarin or aspirin should be discussed with cardiology and cardiac surgery services, as both thrombosis and bleeding can be life-threatening. Prevention of endocarditis is also an important aspect of anesthesia for CHD. These issues are discussed in more detail in Chapter 29.
All patients with intracardiac shunts are at risk of systemic air emboli. Even if shunting patterns are reported as left-to-right, transient right-to-left shunting may occur during the normal cardiac cycle and also during periods of anesthesia, such as intubation or extubation, when the pulmonary vascular resistance (PVR) might be elevated. Air traps or intravenous lines are advisable, although careful attention to detail and constant vigilance to purge air bubbles is the most important intervention to avoid a devastating systemic air embolus.
The preoperative period is an anxious time for parents and adequate preoperative discussion about anesthesia and the induction plan is essential. This discussion may take place in the preoperative clinic or in the hospital when the children are admitted. Clear fluids such as Pedialyte may be given up to two hours prior to operation. Breast milk may be given up to four hours prior to induction. Cyanotic or small infants may be admitted preoperatively for intravenous placement and hydration prior to cardiac catheterization or surgery.
In the majority of neonates, premedications are not administered until the patient is safely placed in the operating room or cardiac catheterization laboratory. A small dose of intravenous midazolam or fentanyl may facilitate monitor placement and avoid periods of extreme cyanosis due to agitation. Infants may receive premedication based on anticipation of a difficult separation from parents in the preoperative area or in patients who have limited hemodynamic reserve and who are unsuited for an inhalational induction. Oral premedication, such as midazolam, can act as an anxiolytic and may be combined with oral ketamine. Intramuscular premedication with midazolam and ketamine is very effective and is often more reliable due to difficulties some children have taking a premedication. Patients who receive a premedication should be carefully monitored, as the effect can be quite variable, especially in cyanotic patients who might have a decreased hypoxic drive to breath [4] and in children with decreased reserve.
Careful attention must be paid to detail at all times when giving anesthesia to patients with CHD. The cardiovascular system in the neonate and infant is primarily dependent on heart rate and preload for adequate cardiac output. While an intravenous induction is preferable, many neonates and infants may present without intravenous access or have very difficult intravenous access. The younger the patient, the more desirable is an intravenous induction. Neonates should be induced intravenously, but in older infants some cardiac lesions may tolerate a low-dose inhalational anesthetic for intravenous placement, but care should be taken with aggressive inhalational inductions in all but the most straightforward of cardiac lesions. Other strategies such as intramuscular induction may need to be considered in order to be able to fully manage the airway, which may be necessary to avoid issues with prolonged inhalational induction. Following intravenous placement, consideration should be given to a fluid bolus with either crystalloid or a colloid such as albumin, prior to proceeding with induction, especially in patients who have a large fluid deficit, those on multiple diuretics, and children with left ventricular or right ventricular outflow tract obstructive lesions. Some patients may require low-dose inotropic support such as dopamine infusions or boluses of ephedrine, phenylephrine, or calcium gluconate in order to maintain adequate blood pressure and cardiac output during induction and the transition to positive pressure ventilation.
Concomitant Issues
Chromosomal abnormalities have been reported with various frequencies in patients with CHD. DiGeorge syndrome, which has now been identified as a deletion in 22q11.2, deserves specific mention due to its prevalence in patients with CHD, specifically interrupted aortic arch and conotruncal defects such as truncus arteriosus, transposition of the great arteries, double outlet of the right ventricle, and Tetralogy of Fallot. 22q11.2 syndrome is also known as velocardiofacial syndrome. Patients who have DiGeorge syndrome have a small or absent thymus and need irradiated blood products to prevent graft-versus-host disease. These patients have hypoparathyroidism with resultant hypocalcemia. Also important for the anesthesiologist are the associated dysmorphic features of the head and oropharynx, which may make either bag-mask ventilation or intubation challenging.
Children with heterotaxy can have abnormal situs and the majority will have congenital cardiac anomalies. It is important to understand that these patients have many different cardiac anomalies, including single ventricles and complex venous anatomy [5]. When taking care of these patients, the anesthesiologist should also be aware of the multiple systemic manifestations of heterotaxy, including midline defects such as: cleft lip or palate; abnormal ciliary function which may result in impaired pulmonary function; and asplenia or polysplenia, which may result in abnormal immune function.
Technical Procedures
For cardiac surgical procedures, including many cardiac catheterization or other diagnostic procedures required in neonates and infants, the anesthesiologist will be required to place an endotracheal tube. These endotracheal tubes may be placed either nasally, with potential benefits of decreased sedation requirements in the ICU and more stability; or orally, which are generally more easily placed.
Ventilation is critical to the successful management of children with CHD. Reductions or increases in PVR caused by mechanical ventilation should be appropriately managed with careful attention to the administration of oxygen and the degree of hyper- or hypocarbia in relation to the specific cardiac anatomy and physiology. In general, we aim for a slower respiratory rate and ventilate at tidal volumes of 8–10 ml kg–1. Small endotracheal tubes may be susceptible to mucous plugging. However, suctioning of the airway should be done carefully in any patient who has systemic or suprasystemic right ventricular pressures, and it is often best to ensure adequate sedation and/or paralysis prior to doing so.
Venous and arterial access in neonates and infants can be a challenge. Many patients will be on prostaglandin infusion, or admitted for prehydration, and will have intravenous access in place before the procedure. It is important to distinguish patients who may not tolerate either any inhalational induction or a prolonged inhalational induction. Patients who present to the operating room without intravenous access, an oral or intramuscular premedication might be considered. If the child is insufficiently calm for placement of intravenous access, an intramuscular induction may be performed as long as the anesthesiologist feels comfortable about the ability to bag-mask ventilate and intubate without an intravenous line in place. There is much variation across different centers in terms of central venous access. Because of previous procedures, some vessels may be occluded. With ultrasound, the internal jugular vein is often the preferred location, although others prefer subclavian or femoral venous access. Some neonates may have an umbilical venous catheter, but these are usually removed after surgery due to the risk of infection. Patients who undergo primary sternotomy may not need central access if the surgeon provides a right or common atrial line prior to separation from bypass. Arterial access may be obtained in the radial or femoral arteries.
Blood Products and Cardiopulmonary Bypass
The majority of neonates and infants with CHD will need blood or blood products at some point during their hospitalization. The risks of transfusion must be balanced with concerns related to systemic oxygen delivery in the setting of patients who may have cyanotic lesions or have poor cardiac output.
The bypass temperature, use of deep hypothermic circulatory arrest versus low-flow antegrade perfusion, the acceptable degree of hemodilution, the use of antifibrinolytics, the use of steroids, the choice of myocardial cardioplegic solution, the ideal arterial oygenation, and the flow on cardiopulmonary bypass remain controversial [6]. Some centers use miniaturized bypass circuits to reduce hemodilution and many use ultrafiltration to achieve the highest possible hematocrit following cardiopulmonary bypass. However, the vast majority of infants and neonatal patients will still require blood on cardiopulmonary bypass. In addition, many patients will require platelets and/or cryoprecipitate following cardiopulmonary bypass. Some institutions practice blood product administration algorithms, while others do not. It is beyond the scope of this chapter to discuss all the pertinent issues of bypass and blood products, as there is much variability between centers.
Disposition
The majority of neonates undergoing cardiac catheterization or cardiac surgery will return to the intensive care unit postoperatively. In some cases, neonates or infants may be extubated in the cardiac catheterization laboratory or, less commonly, in the operating room. However, this practice should be based on multiple factors, including the comfort level of the intensivists and support staff in managing neonatal or infant airways.
Neurodevelopmental Outcomes
There has been much interest in the last decade regarding the neurodevelopmental outcomes of patients with CHD. Of specific interest are those patients who had surgery in the neonatal period requiring deep hypothermic circulatory arrest. Variations in practice between surgical sites include duration of circulatory arrest, use of selective antegrade cerebral perfusion, cooling strategy, acid–base management during cardiopulmonary bypass (alpha versus pH stat), and hematocrit levels on cardiopulmonary bypass [7]. The effects of anesthetics on the neurodevelopmental outcome of patients with CHD are unknown, but are a subject of interest and research [8].
Lesion Types
Congenital cardiac disease is a very complex topic and can be thought of in many different ways. We have chosen to classify cardiac lesions into six major categories; these are not mutually exclusive and patients may have combinations of these various lesions (Table 28.1). An understanding of the anatomy and physiology, along with the anesthetic implications of each type of lesion, will allow the anesthesiologist to safely care for any neonate or infant with CHD. However, this chapter should not be considered to be an exhaustive review.
Table 28.1 Cardiac lesion divided by physiologic effect
Left-to-right shunts Atrial septal defects (ASDs) Ventricular septal defects (VSDs) Atrioventricular septal defect (AVSDs) Truncus arteriosus and aortopulmonary window Patent ductus arteriosum (PDA) Anomalous left coronary artery from the pulmonary artery |
Left ventricular or systemic outflow obstruction Aortic coarctation Critical aortic stenosis Interrupted aortic arch Mitral stenosis |
Inadequate pulmonary blood flow Critical pulmonary stenosis Pulmonary atresia, intact ventricular system Pulmonary atresia, ventricular septal defect Tetralogy of Fallot Ebstein’s anomaly |
Mixing lesions and single ventricles Transposition of the great arteries Hypoplastic left heart syndrome Tricuspid atresia Other single ventricles |
Pulmonary venous obstruction Total anomalous pulmonary venous return Pulmonary venous obstruction |
Airway obstructive lesions Tetralogy of Fallot with absent pulmonary artery Vascular rings Pulmonary slings |
Left-to-Right Shunts
The degree of left-to-right shunt depends on the size of the defect, the compliance of the right and left ventricles, and the vascular resistance in the pulmonary and systemic circulations. Thus, as PVR begins to fall in the neonatal period, these lesions may present with heart failure symptoms due to an increase in the left-to-right shunt, excessive pulmonary blood flow, and the volume load imposed on the left side of the heart. If unrepaired, these patients may eventually develop pulmonary hypertension. Initial medical therapy consists of standard heart failure management including diuretic therapy. However, surgical repair may be required due to failure to thrive. Surgical treatment of a left-to-right shunt may include complete repair of the defect, or palliation with a pulmonary artery band to control pulmonary blood flow.
Atrial Septal Defect
Atrial septal defects (ASDs) are divided into four standard types. The most common is the ostium secundum or fossa ovalis defect. The second most common is the ostium primum, which occurs with an atrioventricular septal defect (AVSD) or endocardial cushion defect. This type of ASD will be discussed more in detail under AVSD. The third type of ASD is a sinus venosus type which is associated with partial anomalous pulmonary venous drainage. Superior sinus venosus defects often involve communication between the upper and/or middle pulmonary veins and the superior vena cava, and inferior sinus venosus defects involve the right lower pulmonary vein and inferior vena cava. The fourth is the coronary sinus defect type which is a defect of the wall between the coronary sinus and the left atrium. There is often a persistent superior vena cava draining into the coronary sinus in these patients.
Patients with ASDs tend to be asymptomatic at birth. The degree of left-to-right shunting is determined by the relative right and left ventricular compliances. Patients are generally asymptomatic and the lesion is usually incidentally discovered. If left untreated, these patients will develop right atrial and right ventricular dilation due to volume overload on the right cardiac chambers. Longstanding atrial-level shunts can result in the development of atrial arrhythmias and pulmonary hypertension. Isolated secundum ASDs rarely need to be closed in infancy. Repair is undertaken when there is evidence of right ventricular volume overload, and usually at preschool age.
Surgical closure remains the gold standard for large secundum ASDs, sinus venosus ASDs, and coronary sinus ASDs. This is generally performed through a sternotomy with cardiopulmonary bypass. Considerations for anesthesia include potential for residual defects, atrial arrhythmias, and pulmonary hypertension. Pulmonary venous obstruction may occur with repair of venosus defects. Redirection of the IVC flow to the LA may occur with repair of secundum defects. Careful post-bypass assessment should be made using TEE.
Percutaneous closure of secundum ASD in infancy has been reported. This procedure generally requires general anesthesia. Transesophageal echocardiography in conjunction with cardiac catheterization imaging techniques are required to both size and place these devices. In a recent series of 128 patients with a mean age of 1.9 years (range 3 months to 4.9 years) there were five major complications, including stroke/seizure, complete heart block, mitral regurgitation, emergency surgery, and cardiac arrest [9].
Ventricular Septal Defects
Ventricular septal defects (VSDs) are classified based on their anatomic location and have various nomenclatures. There are four types. Perimembranous is the most common VSD and is in the membranous region of the septum, under the aortic valve, closely associated with the tricuspid valve. These types of VSD rarely close when they are large, and do not restrict flow. The aortic valve leaflet may prolapse into the defect and result in aortic regurgitation. Muscular VSDs are located in the muscular septum. They may be multiple and can be Swiss-cheese-like. Small muscular VSDs may spontaneously close. An atrioventricular canal VSD is associated with an atrioventricular canal defect and is located in the inlet septum. The AVSD will be discussed more below. Lastly, the doubly committed subarterial VSD, is found between and just under the aortic and pulmonary valves. Subarterial VSDs do not close and the aortic valve may prolapse into the defect, leading to aortic insufficiency.
Children with large and unrestrictive VSD will present in infancy with congestive heart failure (CHF) and failure to thrive. There is evidence of left ventricular volume overload on echocardiography. Diuretics are usually prescribed and enteral tube feeding may be needed. Repair is recommended in the first year of life to prevent the development of pulmonary vascular disease. In patients without heart failure, and prolapse of the aortic valve into the VSD, developing aortic insufficiency will be an indication for surgery. Endocarditis is an uncommon indication. In a subarterial VSD, the presence generally indicates surgical repair.
Anesthetic considerations of nonrestrictive VSDs include the potential for excessive flow to the pulmonary vasculature. After induction, a significant drop on PVR should be avoided by decreasing inspired oxygen to room air and avoiding hypocarbia. Patients will generally have good ventricular function and if complete repair is planned, the ventricle postoperatively will have a decreased volume load. Rarely, inotropic support will be needed unless a ventriculotomy was required to close the VSD.
Ventricular septal defects are usually closed through a sternotomy on cardiopulmonary bypass. The approach to a VSD depends on location. Most perimembranous VSDs can be adequately accessed through an incision in the right atrium and visualized through the tricuspid valve. In some instances, the tricuspid valve may need to be detached to allow for adequate inspection. Other VSDs may require an incision in the right ventricle itself. Subarterial VSDs may be repaired through an incision in the right ventricle, but more commonly through an incision in the pulmonary artery. Occasionally an aortotomy may be required to repair both a subarterial VSD, as well as the aortic valve, depending on the degree of insufficiency.
Muscular VSDs may be closed in the cardiac catheterization laboratory. A combination of transesophageal imaging and cardiac angiography is required to adequately delineate anatomy and the relationship between the VSD and cardiac valves. If possible, the device is delivered from the venous side, but this requires a large sheath. VSDs are often technically easier to cross from the left side due to the presence of trabeculations in the right ventricle, which make wire placement difficult. Thus, the wire path can be either from femoral vein to right atrium to left atrium across the atrial septum. The wire then courses from the left ventricle through the VSD to the right ventricle, then through the tricuspid valve to the right atrium to the femoral vein or internal jugular vein. Alternatively, the wire can course from the aorta through the aortic valve, into the left ventricle, across the VSD, into the right ventricle, through the tricuspid valve and into the right atrium, where it can either exit to the femoral vein or internal jugular vein. A large sheath is then placed over the wire from the right ventricle to the left ventricle across the VSD and a device is deployed. Anesthetic concerns in this procedure include multiple access sites, arrhythmias, and low cardiac output caused by multiple wires and sheaths stenting valves open, necessitating inotropic support. There is also a high incidence of blood transfusion due to bleeding. Devices can also be embolized and can impinge on other cardiac structures, including valves [10].
In cases with multiple VSDs or in very small neonates, a pulmonary arterial band may be placed to control pulmonary blood flow. Banding of the pulmonary artery to reduce pulmonary blood flow is a difficult palliative procedure. If the band is too tight, severe cyanosis may occur; if the band is too loose, the increase in pulmonary blood flow will contribute to CHF and possibly increased PVR. Distortion, stenosis, and migration of the band may complicate later surgery, cause right ventricular hypertrophy, subaortic stenosis, and pulmonary valve stenosis. Determining the correct size of a band at the time of surgery is difficult and there are no accurate formulae for band size. Echocardiographic or direct pressure measurements may be used to adjust the band. At a subsequent operation to repair the VSD, the band will be taken down.
Transesophageal echocardiography is often used intraoperatively to assess the adequacy of repair. Epicardial echocardiography may also be used when TEE is contraindicated. Intraoperative calculation of any residual shunt is performed by identifying a “step-up” in the saturation measured from the superior vena cava and the main pulmonary artery. A shunt fraction (Qp:Qs) of greater than 1.5 is generally considered significant. Residual shunts are more commonly encountered when tackling multiple muscular VSDs. There is also a risk to the conduction system with perimembranous VSD repairs, with a risk of up to 2 percent requiring a permanent pacemaker [11].
Atrioventricular Septal Defects
A common atrioventricular (AV) canal, complete ASD, or endocardial cushion defect consists of inlet atrial and ventricular septal defects and a common AV valve. There is shunting at both the atrial and ventricular levels. The ventricular defect may be large and nonrestrictive. Clinically, the patients present in infancy with symptoms of CHF, as the physiology is determined by the degree of shunting at the ventricular level. In the spectrum of AVSD, the VSD may be restrictive or absent, making the primum ASD determine the clinical presentation. These are the transitional or partial AVSDs.
Patients with complete AVSDs may be medically managed with diuretic therapy and repaired at 3–6 months of age, prior to the development of pulmonary vascular disease. The surgical approach to this includes both closure of the ASD and VSD, but also division of the common AV valve into right- and left-sided valves. This may be accomplished with several different techniques, including a single patch, a modified single patch (Australian technique), or a two-patch technique. Closure of a cleft in the left AV valve is a routine part of the operation [12].
Anesthetic concerns in patients with AVSD include a large left-to-right shunt that may result in CHF and/or pulmonary hypertension. Maneuvers such as induction of anesthesia and mechanical ventilation, which further decrease PVR, or agents which act as myocardial depressants, may be poorly tolerated in these patients. These patients are at risk for residual ASDs or VSDs, and AV valve stenosis or regurgitation. Left ventricular outflow tract obstruction may also occur. Patients with trisomy 21 have an increased association with endocardial cushion defects and anesthetic concerns in these patients should include potential large tongues and c-spine instability, among others [13].
Truncus Arteriosus
Truncus arteriosus is a relatively rare cardiac anomaly and is defined as a single arterial vessel arising from the heart, receiving blood from both ventricles, and supplying blood to the aorta, lung, and coronary arteries. The embryonic truncus fails to develop into two great arteries and straddles a large VSD. There is complete mixing of blood in the single great artery. One or two pulmonary arteries may arise from the single truncus. There is a single truncal valve which may be regurgitant or stenotic. As the PVR drops in the neonatal period, blood flow increases to the pulmonary circulation, which may result in increased arterial oxygen saturation and symptoms of CHF. Increasing pulmonary blood flow may create a steal phenomenon resulting in decreased systemic blood flow and cardiac output associated with low diastolic pressure causing insufficient blood flow to the coronary circulation, resulting in ischemia and ventricular fibrillation.
In a neonate or infant with truncus arteriosus, anesthetic management must be done carefully with attention paid to balancing the systemic and pulmonary circulation. Induction, specifically preoxygenation and hyperventilation, will decrease PVR, causing excessive pulmonary blood flow, systemic hypotension, and myocardial ischemia. In general, the patient is maintained on room air to increase the PVR and a narcotic-based technique is employed; agents that may cause myocardial depression should be avoided or administered very carefully, especially in those patients with truncal valve regurgitation or preexisting ventricular dysfunction. If measures to increase PVR do not decrease pulmonary blood flow, the cardiac surgeon may need to occlude a branch pulmonary artery to restore systemic perfusion pressures until cardiopulmonary bypass can be instituted. Repair of this lesion involves VSD closure, establishing right ventricle to pulmonary artery continuity with a homograft conduit, and repair of truncal valve regurgitation.
Following the repair of truncus arteriosus, measures should be taken to avoid any further pulmonary arterial hypertension and right ventricular dysfunction. Pulmonary hypertension can be anticipated in infants who present beyond the neonatal period and have preoperative elevation of pulmonary arterial pressures. Precautions against pulmonary arterial hypertensive crises should be taken, including hypoxia, hypercarbia, acidosis, pain, airway stimulation, and left ventricular failure. Any residual VSD will result in a volume and pressure load on the right ventricle, which may be a significant problem in the postoperative period. The majority of patients will require inotropic support for the right ventricle in the early postoperative period. Truncal valve stenosis or regurgitation may significantly impair left ventricular function and may necessitate re-repair or replacement with a homograft. Neonates with a right ventricle to pulmonary artery conduit are at risk for conduit compression and often undergo delayed sternal closure.
Aortopulmonary Window
Aortopulmonary (AP) windows are caused by failure of fusion of separation of the truncus arteriosus in the developing fetus. This results in a connection between the aorta and pulmonary artery. These patients may be diagnosed after birth with a left-to-right shunt that increases as the PVR drops. The connection between the aorta and pulmonary artery can be small and restrictive, or can be large and result in tachypnea, poor feeding, and diaphoresis. If decreased systemic perfusion develops, one should consider measures to attempt to control shunting, including increasing PVR. If the connection is very large, bidirectional shunting can result in systemic desaturation. In the vast majority of cases, surgical closure remains the procedure of choice in which the aorta and pulmonary artery are divided and a patch placed between to eliminate the shunt. There are case reports of closure of anatomically favorable AP windows, closed in the cardiac catheterization laboratory using devices usually used for PDA or ASD closure [14]. Like most patients with large left-to-right shunts, they should do very well postoperatively [15].
Patent Ductus Arteriosus
A PDA may be found in both the premature neonate, as well as in older infants. The PDA is a fetal vascular communication between the descending, usually just below the origin of the left subclavian artery, and the main pulmonary artery. During fetal circulation the ductus arteriosus is a conduit of blood for lower body systemic perfusion. As the PVR increases immediately at birth with the first breath, blood flow through the ductus may reverse right-to-left. The degree of shunting through the PDA is determined by both the resistance of the pulmonary and systemic vasculatures, as well as the diameter of the duct itself. Normally, the ductus will close shortly after birth. However, it may remain patent. As PVR falls in the neonate, a left-to-right shunt will ensue. If the PDA is large and nonrestrictive there will be excessive pulmonary blood flow, causing a volume load on the left ventricle and CHF. In addition, the left-to-right shunt will result in a low diastolic blood pressure due to the flow of blood to the pulmonary circulation in diastole. These “steal” effects of blood flow in systole and diastole may result in decreased end-organ perfusion.
The management of PDAs remains somewhat controversial, especially in premature neonates [16]. Indications to close a PDA include prolonged ventilation, evidence of left ventricular dilation suggesting volume overload, and gastrointestinal complications felt related to the left-to-right shunt. Indomethacin or ibuprofen may be used to medically close the duct. However, the use of nonsteroidal anti-inflammatory medications may be associated with risks including renal dysfunction, intracranial hypertension, and gastrointestinal complications [17].
When an isolated PDA is surgically closed, it usually consists of a left thoracotomy with ligation of the duct using clips. Cardiopulmonary bypass is not required. A PDA may also be ligated through a sternotomy when other procedures are necessary. In infants, a video-assisted thoracoscopic surgical (VATS) approach may be used to decrease pain postoperatively and improve cosmesis [18].
Some institutions prefer to perform this procedure in the neonatal intensive care unit, while others prefer to perform this in the operating room due to better lighting and suction in the event of any disastrous bleeding or inadvertent ligation of the descending aorta or left pulmonary artery. Anesthetic issues in the very premature infant include those of severe lung disease and the surgical requirement for lung retraction to identify and ligate the PDA. A decrease in lung volume, as well as an increase in lung compliance, often increases oxygen and ventilatory requirements. In general, a combination of narcotic and a paralytic are all that are required. Arterial pressure monitoring is rarely required. However, ligation of a significant PDA will often cause an immediate increase in the diastolic blood pressures and the sudden increase in afterload may cause some left ventricular dysfunction [19]. There is also the potential for transient or permanent injury to the recurrent laryngeal nerve, and this should always be considered in any patient who has stridor postoperatively [20].
In the interventional suite, PDAs may be percutaneously closed using a coil or other occlusion device [21]. This may be done under sedation or general anesthesia based on concomitant comorbidities and the preference of the interventional cardiologist.
Anomalous Left Coronary Artery from the Pulmonary Artery (ALCAPA)
The left (or right) coronary artery arises anomalously from the pulmonary artery. In infant cases there is little to no development of coronary collaterals [22]. Due to the low diastolic pressures in the pulmonary arteries, these patients will have early onset of myocardial ischemia, which leads to left ventricular dysfunction and dilation, and is associated with mitral valve regurgitation. Any infant with left ventricular dysfunction should undergo diagnostic imaging to rule out ALCAPA. These patients may present incidentally and be profoundly ill and in shock, needing intubation and high-dose inotropic support. If these children are not intubated, induction should proceed very carefully with inotropic support readily available and often prophylactically initiated to avoid further cardiac ischemia. Repair usually involves transfer of the anomalous coronary artery to the aorta. Ventricular function will not improve immediately and these patients may require temporary mechanical circulatory support (ECMO). If patients with this anomaly present late, and ventricular function does not recover, they may be considered for longer-term mechanical support or cardiac transplantation.
Decreased Systemic Blood flow
Aortic Coarctation
Aortic coarctation occurs as a narrowing of the descending aorta near the insertion of the ductus arteriosus. Patients may have a concomitant hypoplastic aortic arch, aortic and mitral valve abnormalities, or VSDs. In the neonate who has severe aortic coarctation, the increased left ventricular afterload, and therefore left ventricular end-diastolic pressure, is not well tolerated in the noncompliant neonatal heart and will result in pulmonary hypertension. This increase in pulmonary arterial pressure will permit flow to the lower body through a patent ductus arteriosus. As the PDA closes, the lower limb pulses will become weak and may disappear. The baby may present with shock as the PDA closes. In neonates who present with this diagnosis, prostaglandins are usually started to maintain ductal perfusion to the lower body. In the most ill patients, left ventricular function may be depressed, but will likely recover with opening of the ductus by a reduction in the left ventricular end-diastolic pressure.
In general, neonates are operated on soon after the diagnosis is made. The optimal treatment is felt to be complete excision of all ductal tissue and an end-to-end or extended end-to-end repair. Alternatively, in extremely small children or long segment coarctation, the subclavian artery can be used as a flap to augment the aorta. This is well tolerated, but will result in decreased or absent pulses in the left arm. In general, patches, including Dacron, are avoided when possible, as patch material has been associated with late aneurysm formation [23].
Repair of isolated aortic coarctation is usually performed through a left thoracotomy incision, off-bypass. In these cases, there is a 10–25 minute period of aortic cross-clamping while the coarctation is excised and the repair is performed; during this period, the spinal cord is at risk of ischemic injury. Although the time of cross-clamp is probably the most important factor, it is extremely important to have careful anesthetic management, including maintenance of blood pressure through monitoring of right radial arterial pressures. Invasive monitoring of arterial pressures in the lower extremities may be helpful, but it is impractical in the neonate or infant with aortic coarctation, as they do not usually have sufficient collateral development. Patients who present with preoperative left ventricular dysfunction may require inotropic support during the repair. Hyperthermia is to be avoided, but mild hypothermia (33–35 °C) may be beneficial for spinal cord protection.
After aortic cross-clamp, hypertension proximal to the area of coarctation may develop. This can cause an abrupt increase in the left ventricular end-diastolic pressure and result in left ventricular dysfunction. Younger patients may not have collateral blood flow to the lower body, and in these patients care must be taken to avoid excessive vasodilation prior to repair, as it may result in decreased lower body perfusion.
Following repair and after removal of the cross-clamp, rebound hypertension may be a problem. Patients are also at risk for bowel ischemia due to insufficient flow in the mesenteric artery if hypertension is poorly controlled. Sodium nitroprusside, along with beta blockade, may be required in the early postoperative period until patients can be started on oral afterload-reducing agents such as an ace inhibitor.
Neonates or infants who present with coarctation may undergo balloon dilation of the descending aorta. Sometimes this may be done in the sickest neonates or those with other comorbidities, in order to palliate before surgical repair. However, neonates and infants have a high rate of reocclusion [24]. These procedures are generally performed under a general anesthetic as the balloon dilation can be very stimulating. It is important in these patients to monitor blood pressures in the right arm, and to pay careful attention to blood pressure control during and after the procedure.
Critical Aortic Stenosis
Neonates with critical aortic stenosis will generally have fusion of various valvular commissures and it is often associated with left ventricular endocardial fibroelastosis. In addition, some of these patients may have associated mitral valve dysplasia or other types of left ventricular outflow tract obstruction. This lesion is poorly tolerated, and neonates will develop left ventricular dysfunction, which may even result in a right-to-left shunt at the PDA level and left-to-right shunting through an interatrial communication.
Medical management of these patients includes initiation of prostaglandin infusion to try to improve systemic perfusion and therefore decrease acidosis. Percutaneous balloon angioplasty is often the preferred treatment strategy, but may result in multiple complications, including residual aortic stenosis, persistence of left ventricular dysfunction, or aortic regurgitation. Aortic insufficiency is poorly tolerated [25].
Surgical management for these patients may include a surgical valvotomy, which must be performed on cardiopulmonary bypass. The risks of valvotomy are similar to those performed in the catheterization laboratory. In those cases where the left ventricle is small or if the mitral valve is sufficiently dysplastic, a decision may be made to proceed with a single ventricle palliation.
Anesthetic considerations in these patients are those of a neonate who has little myocardial reserve. A high-dose narcotic-based anesthetic is advantageous to maintain hemodynamic stability. If the child is not on inotropic support, it is advisable to initiate it prior to proceeding with induction of anesthesia. These patients may be dependent on ductal flow, and as such significant reductions in PVR will result in poor systemic perfusion and acidosis (avoid hyperventilation, hyperoxia, alkalosis, and hyperthermia). These patients will generally have a prolonged ICU course with need for inotropic support initially following even successful procedures.
Interrupted Aortic Arch
In patients with an interrupted aortic arch (IAA), the aorta is completely interrupted at one or more points in the aortic arch. The most common site of interruption is between the left common carotid and the left subclavian artery, but the interruption may occur after the subclavian artery or, more rarely, between the innominate and carotid arteries. A nonrestrictive VSD, which is almost always present, and the PDA supply systemic blood flow below the interruption. Patients with interruption may have concomitant left ventricular outflow tract obstruction [26].
Patients with IAA at birth will appear well perfused due to the presence of a PDA and the high PVR of the newborn. If the diagnosis has not been made prenatally, the patient may present in shock as the PDA closes. A prostaglandin infusion is necessary to maintain lower body perfusion. If prostaglandins are not initiated, these neonates will begin to develop sequelae of inadequate lower body perfusion including lactic acidosis, hepatic and renal dysfunction, and gut ischemia. Patients presenting in shock are stabilized prior to surgical repair. Operative repair consists of a patch closure of the VSD and a direct anastomosis of the descending and the underside of the transverse arch.
A prostaglandin infusion should be continued in the operating room until the onset of cardiopulmonary bypass. Upper and lower body perfusion is monitored by cerebral and flank near-infrared spectroscopy (NIRS) and by nasopharyngeal and rectal temperature probes. If the flank NIRS is low and the rectal temperature does not decrease after cooling on cardiopulmonary bypass, these are indications of poor lower body perfusion. The surgeon may elect to additionally cannulate and perfuse through the PDA. This procedure requires a period of deep hypothermic circulatory arrest or antegrade cerebral perfusion. Anesthetic management is the same as in patients with critical aortic stenosis.
Residual lesions after repair include residual VSD, subaortic obstruction, or aortic arch gradient. These patients may later develop recurrent arch obstruction necessitating cardiac catheterization in later infancy or childhood.
Mitral Stenosis
Mitral stenosis can occur at multiple levels, including supravalvular, valvular, and/or subvalvular. Supramitral rings are part of the Shone’s complex (supravalvular mitral membrane, parachute mitral valve, subaortic stenosis, and coarctation of the aorta). Parachute mitral valves may have subvalvular obstruction.
In neonates or infants with severe congenital mitral stenosis, balloon dilation of the mitral valve may be an effective palliation, but there are risks for mitral regurgitation, arrhythmia, and stroke. Mitral stenosis will result in left atrial hypertension and associated pulmonary artery hypertension; if there is no ASD or PFO present decompression with an atrial septostomy may be required. The objective is to palliate the child to allow somatic growth, as there are no durable valve replacement options in neonates and infants.
There are few good options for surgical management of mitral stenosis. Repairs are possible, but difficult in neonates and infants due to the delicate valve tissue. Repairs must address all valvular levels of obstruction, but may not be durable. In general, prostheses are too large for these small patients. There has been some success in implanting an externally stented bovine jugular vein graft (Melody valve) in the mitral position, as it can be progressively dilated in the catheterization laboratory as the patient grows [27].
Anesthetic considerations of mitral valve stenosis include avoidance of tachycardia and reductions in afterload, as in any patient with left-sided obstruction. These patients may be at risk for atrial arrhythmias due to enlarged left atriums. Some patients with mitral stenosis will not tolerate any loss of atrioventricular synchrony and care should be taken when placing central venous access. In addition, these patients may have developed pulmonary hypertension associated with right ventricular hypertension and dysfunction, and avoidance of further precipitants is important. Inotropic support is often necessary from induction of anesthesia. After intervention on the mitral valve, there may be residual mitral stenosis and/or regurgitation. Implantation of a mitral prosthesis may lead to heart block or ischemia due to compression of the circumflex coronary artery. Sometimes, the prosthesis may need to be placed in the supra-annular position, within the left atrium.
Decreased Pulmonary Blood flow
Critical Pulmonary Stenosis
Infants with critical pulmonary stenosis have only a very small orifice across the pulmonary valve. In these patients pulmonary blood flow is dependent on an atrial-level right-to-left shunt and pulmonary blood flow with left-to-right flow through a PDA. Therefore, if the PDA closes after birth, they will become very cyanotic due to the absence of any other source of pulmonary blood flow. A prostaglandin infusion is used to maintain PDA patency. In general, these patients are reasonably stable and are taken for percutaneous pulmonary balloon dilation in the catheterization laboratory the first few days of life. Following the balloon dilation, it may take some time for improvement in the right ventricular hypertrophy to allow for antegrade flow through the right ventricular outflow tract.
Anesthetic considerations of critical pulmonary stenosis include those of a patient who has a ductal-dependent lesion including continuation of prostaglandins. The right ventricle may require inotropic support during the catheterization procedure. Pulmonary valve balloon dilation may result in regurgitation, which is generally well tolerated.
Those patients who fail percutaneous balloon dilation may undergo surgical valvotomy. Alternatively, the right ventricular outflow tract may be reconstructed with a transannular patch or a right ventricle-to-pulmonary artery homograft conduit. If there is a contraindication, a modified Blalock–Taussig (BT) shunt can be placed to maintain pulmonary blood flow. Considerations of a modified BT shunt are discussed below.
Pulmonary Atresia, Intact Ventricular System
Neonates born with pulmonary atresia and intact ventricular septum (PA, IVS) often have a very small right ventricle and tricuspid valve. There is generally a plate-like obstruction between the right ventricle and the pulmonary arteries. These neonates are cyanotic and dependent on an adequate atrial-level shunt and pulmonary blood flow across a PDA. The size of the right ventricle and tricuspid valve will determine whether the patient is eligible for a two-ventricle repair, or needs single-ventricle palliation.
After prostaglandin initiation, these patients will undergo echocardiography and cardiac catheterization in order to assess the size of the tricuspid valve (TV), right ventricle, and the coronary anatomy. Some patients will have fistulous connections between the small hypertensive right ventricle and the coronary arteries. The coronary circulation may be dependent on these fistulous connections, so-called right ventricle-dependent coronary circulation. If the right ventricle is decompressed, with resultant decrease in pressures, flow through these fistulae will be impaired. The resultant myocardial ischemia can lead to cardiac arrest.
In the cardiac catheterization laboratory, attempts can be made to open the right ventricular outflow tract (RVOT). This may be done by either a stiff wire or a radiofrequency ablation catheter, followed by balloon dilation of the RVOT. In patients in whom the right ventricle and TV are clearly too small, the PDA may be stented to allow a reliable source of pulmonary blood flow. Alternatively, these patients may undergo a modified BT shunt in the operating room [27].
If the right ventricle and TV appear adequate for a two-ventricle repair and attempts to open the RVOT in the catheterization laboratory are unsuccessful, patients may go to the operating room for a transannular patch across the RVOT. A modified BT shunt may be added if there is concern for adequacy of pulmonary blood flow. Alternatively, a right ventricle to pulmonary artery conduit may be used for reconstruction of the RVOT.
These neonates are often intubated and in the intensive care unit. Like any patient with a patent PDA, there must be care taken to not cause excessive pulmonary blood flow. Most patients will be on room air and should remain on it, especially those patients with a patent PDA when a secondary source of pulmonary blood flow is created through the RVOT. The right ventricle is hypertrophied and may have resultant systolic and diastolic dysfunction. In patients with right ventricle-dependent coronary fistulae, right ventricular pressures must be maintained, as any drop will lead to resultant myocardial ischemia. These patients are especially at high risk during angiography, because, for several cardiac cycles there will be contrast medium, rather than blood, returning to the myocardium. If there is a suspicion of right ventricle-dependent coronaries on transthoracic echocardiography, the anesthesiologist should be adequately prepared with resuscitation medications, as well as plans for potential extracorporeal mechanical oxygenation (ECMO).
Pulmonary Atresia With Ventricular Septal Defect
Pulmonary atresia with VSD is a spectrum of conditions in which there can be confluent, well-sized, central pulmonary arteries supplied by a PDA, with a single conoventricular VSD. These patients will present with cyanosis and require prostaglandins to secure pulmonary blood flow. Their repair involves VSD closure with reconstruction of the RVOT, usually with a homograft conduit. If there are contraindications to cardiopulmonary bypass, then a modified BT shunt can be used to palliate the patient.
At the other end of the spectrum, the central pulmonary arteries may be absent and the pulmonary vasculature is diffusely hypoplastic. These patients have major aortopulmonary collaterals (MAPCAs), which are fibromuscular collateral arteries that supply multiple or all segments of the lungs. These patients may present with only very mild cyanosis. There is usually no PDA present, or pulmonary blood flow may not be PDA-dependent. If the MAPCAs are significant in number and size, there may be CHF.
There are generally two repair strategies for these patients. The strategy chosen will depend on the size of any existing central pulmonary arteries and the institutional philosophy. In the staged approach, if there are accessible central hypoplastic pulmonary arteries, an aortopulmonary shunt can be constructed to promote pulmonary arterial growth. The same can be achieved with a right ventricle to pulmonary artery conduit. The right ventricle to pulmonary artery conduit has the advantage of allowing catheter-based imaging and interventions on the distal pulmonary vasculature. This will prevent systemic pressure in the pulmonary vasculature. This intermediate stage can require multiple operations through thoracotomies or sternotomies. The final stage will bring together the MAPCAs to the central pulmonary vasculature, so-called unifocalization, and reconstitute the right ventricle to the centrally reconstructed pulmonary artery using a conduit. Depending on the anatomic state of the distal pulmonary vasculature and the pulmonary artery pressures, the VSD may or may not be closed at this time.
Alternatively, the single-stage approach involves bringing together all the MAPCAs, and using existing pulmonary arteries to create a central pulmonary arterial confluence. A right ventricle to pulmonary artery conduit is used to reconstruct the RVOT. The VSD is closed, if pulmonary artery pressures permit. This single stage unifocalization has been advocated by Hanley at Stanford [29].
During unifocalization the anesthesiologist will be challenged during the dissection of the MAPCAs, as these are in the posterior mediastinum. The dissection is done prior to cardiopulmonary bypass. If the MAPCAs are not controlled, there will be a low perfusion pressure on bypass. After complete repair, the condition of the right ventricle should be carefully followed. VSD closure in the face of significant pulmonary vascular disease can precipitate right ventricular failure and right-to-left shunting at the atrial level. Single-stage unifocalizations can be long and complex procedures, followed by low cardiac output and bleeding from multiple suture lines.
Anesthesiologists may encounter these patients at different stages of their disease. It is important to know the details of the repair, the right ventricular function, source of pulmonary blood flow, and presence of ASDs or VSDs to formulate a detailed anesthetic plan.

Full access? Get Clinical Tree
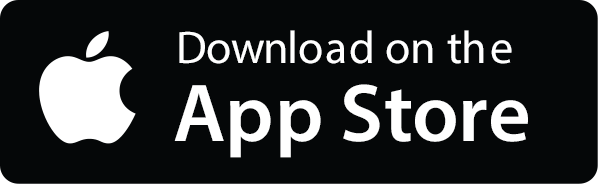
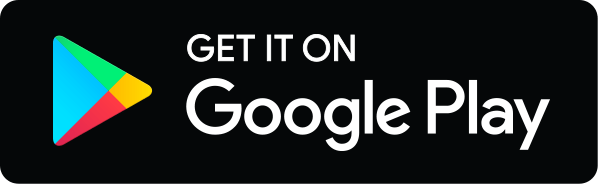
