1903
The conventional modes of ventilation described in the last chapter are appropriate for most patients with acute respiratory failure. However, there are occasions when conventional mechanical ventilation is either unable to support gas exchange in the lungs, or is not necessary as a means of ventilatory support. This chapter describes alternate modes of ventilation that are available when conventional mechanical ventilation is either not sufficient, or not necessary, for patients with acute respiratory failure. Included are rescue modes of ventilation (i.e., high frequency oscillatory ventilation and airway pressure release ventilation) and noninvasive modes of ventilation (i.e., continuous positive airway pressure, bilevel positive airway pressure, and pressure support ventilation).
RESCUE MODES OF VENTILATION
A small percentage (10–15%) of patients with acute respiratory distress syndrome (ARDS) develop hypoxemia that is refractory to oxygen therapy and conventional mechanical ventilation (1). In this situation, the appropriate strategy for ventilatory support is defined by the concept described next.
Open Lung Concept
Alveolar collapse (atelectasis) in ARDS not only impairs alveolar ventilation, it also promotes ventilator-induced lung injury. There are two mechanisms for the lung injury produced by atelectasis. First, if the alveolar collapse is extensive, like that shown in Figure 23.4 (on page 454), the tidal volumes delivered by the ventilator will distribute in the uninvolved (normal) areas of the lungs, and this will overdistend normal alveoli and promote volutrauma. Secondly, if alveolar collapse occurs only at end-expiration, repetitive opening and closing of alveoli promotes atelectrauma by creating excessive shear forces that damage the airway epithelium. (See pages 494–497 for more information on ventilator-induced lung injury.)
The adverse consequences of alveolar collapse can be mitigated by a mode of ventilation that both prevents alveolar collapse and opens collapsed alveoli. This is the open lung concept of mechanical ventilation, where the goal is to: “open up the lung and keep the lung open” (2). The modes of ventilation described next are designed to achieve this goal.
High Frequency Oscillations
High frequency oscillatory ventilation (HFOV) uses high-frequency, low volume oscillations like the ones shown in Figure 27.1. These oscillations create a high mean airway pressure, which improves gas exchange in the lungs by opening collapsed alveoli (alveolar recruitment) and preventing further alveolar collapse. The small tidal volumes (typically 1–2 mL) limit the risk of alveolar overdistension and volutrauma. For optimal results, an alveolar recruitment maneuver (e.g., with high PEEP levels, as shown in Figure 26.5, page 514) is recommended just prior to switching from conventional mechanical ventilation (CMV) to HFOV (3).
FIGURE 27.1 Airway pressure oscillations during high frequency oscillatory ventilation (HFOV), with a superimposed lung inflation during conventional mechanical ventilation (CMV). Dotted line represents mean airway pressure. From Reference 3.
Ventilator Settings
HFOV requires a specialized ventilator (Sensormedics 3100B, Viasys Healthcare, Yorba Linda, CA) that allows the following adjustments: (a) the frequency and amplitude of the oscillations, (b) the mean airway pressure, (c) the bias flow rate (similar to an inspiratory flow rate), and (d) the inspiratory time (time of the bias flow). Some recommendations for these settings are listed in Table 27.1.
OSCILLATIONS: The frequency range for the oscillations is 4–7 Hz (1 Hz is one oscillation per second or 60 oscillations/min, so a range of 4–7 Hz is 240–420 oscillations/min), and the specific frequency selected is determined by the arterial pH (which represents the CO2 burden). The pulse amplitude (tidal volume) determines CO2 removal, and pulse amplitude is inversely related to the frequency of oscillations; i.e., lower oscillation frequencies result in higher tidal volumes and more effective CO2 removal. The initial pulse amplitude is set at 70–90 cm H2O.
MEAN AIRWAY PRESSURE: The end-inspiratory alveolar pressure (e.g., the plateau pressure during volume control ventilation) should be measured just prior to switching from CMV to HFOV. This pressure is a reflection of: (a) alveolar volume, and (b) the risk of alveolar overdistension and volutrauma (see Chapter 25). The mean airway pressure is usually set 5 cm H2O higher than the end-inspiratory alveolar pressure recorded during CMV (3), but should not exceed 30 cm H2O (to prevent volutrauma).
Table 27.1 Suggested Initial Settings for HPOV and APRV
Advantages
Clinical trials comparing HFOV with CMV, primarily conducted in patients with ARDS, have shown a 16–24% increase in the PaO2/FIO2 ratio associated with HFOV (4). Early studies of HFOV showed no impact on mortality rate (3), but a very recent meta-analysis of all available studies shows a significant survival benefit with HFOV (4). However, HFOV has not been compared with lung protective ventilation (which also shows a survival benefit) so it is not known if HFOV represents an advance over lung protective ventilation in patients with ARDS.
Disadvantages
The disadvantages of HFOV are listed below (3,4):
1. A special ventilator is needed, along with trained personnel to operate the device.
2. Cardiac output is often decreased during HFOV because of the high mean airway pressures. This effect requires augmentation of the intravascular volume during HFOV.
3. Aerosolized bronchodilators are ineffective during HFOV.
Airway Pressure Release Ventilation
Airway pressure release ventilation (APRV) employs prolonged periods of spontaneous breathing at high end-expiratory pressures, which are interrupted by brief periods of pressure release to atmospheric pressure. APRV is a variant of continuous positive airway pressure (CPAP), which is spontaneous breathing at a positive end-expiratory pressure. The similarities between APRV and CPAP are demonstrated in Figure 27.2. The upper panel in this figure shows an airway pressure profile for CPAP; in this case, the changes in inspiratory and expiratory pressures during spontaneous breathing revolve around an end-expiratory pressure of 5 cm H2O. The airway pressure profile for APRV (in the middle panel) shows CPAP at a much higher end-expiratory pressure (30 cm H2O), with a brief period where the airway pressure is allowed to decrease to zero (pressure release). The high CPAP level in APRV improves arterial oxygenation by opening collapsed alveoli (alveolar recruitment) and preventing further alveolar collapse (similar to the effects of the high mean airway pressure in HFOV). The pressure release phase is designed to facilitate CO2 removal (5).
Ventilator Settings
APRV is available in many of the modern critical care ventilators, and the variables that must be selected when initiating APRV include the high and low airway pressures, and the time spent at each pressure level. Some suggestions for the initial settings are listed in Table 27.1.
HIGH AIRWAY PRESSURE: As mentioned for HFOV, the end-inspiratory alveolar pressure (e.g., the plateau pressure during volume control ventilation) should be measured just prior to switching from CMV to APRV. (This pressure is a reflection of alveolar volume, and the risk of alveolar overdistension and volutrauma, as mentioned for HFOV). The high airway pressure should equal the end-inspiratory alveolar (plateau) pressure, but should not exceed 30 cm H2O (to limit the risk of volutrauma).
LOW AIRWAY PRESSURE: The low pressure level is set to zero (atmospheric pressure), to maximize the driving pressure for rapid pressure release. However, the pressure never reaches the zero level because the pressure release phase is so brief, and the residual positive pressure helps to prevent alveolar collapse.
TIMING: The time spent at the high airway pressure is usually 85–90% of the total cycle time (time at high pressure plus time at low pressure). Recommended times are 4 to 6 seconds for the high pressure level and 0.6 to 0.8 seconds for the low pressure level.
Advantages
APRV can achieve nearly complete recruitment of collapsed alveoli by maintaining high airway pressures for prolonged periods of time; this not only improves arterial oxygenation, it also reduces lung compliance. As a result of the latter effect, peak airway pressures are lower during APRV than during CMV (at equivalent tidal volumes) (3). The improvement in arterial oxygenation with APRV occurs gradually over 24 hours (6).
FIGURE 27.2 Related modes of pressure-regulated spontaneous ventilation. CPAP=continuous positive airway pressure, APRV=airway pressure release ventilation, BiPAP=bilevel positive airway pressure, IPAP=inspiratory positive airway pressure, EPAP=expiratory positive airway pressure, Pmean=mean airway pressure, I=inspiration, E=expiration. See text for explanation.
Disadvantages
The benefits of APRV are lost if the patient has no spontaneous breathing efforts. Additional disadvantages of APRV are listed below.
1. Severe asthma and COPD are relative contraindications to APRV because of the inability to empty the lungs rapidly during the pressure release phase of APRV (3).
2. Cardiac output is often decreased during APRV because of the high mean airway pressures, but this effect is less pronounced than the cardiac depression during HFOV (3).
NONINVASIVE VENTILATION
Noninvasive ventilation (NIV) is intended for patients with acute respiratory failure who will benefit from ventilatory support, but may not need conventional mechanical ventilation (which requires endotracheal intubation). NIV is delivered via tight-fitting face masks, which obviates the need (and the complications) of endotracheal intubation. Although NIV has applications in the outpatient and inpatient setting, the following description is limited to the use of NIV in acute respiratory failure (7,8).
Modes of Ventilation
There are three modes of ventilation available for NIV: (a) continuous positive airway pressure (CPAP), (b) bilevel positive airway pressure (BiPAP), and (c) pressure support ventilation (PSV). The latter two modes of ventilation (i.e., BiPAP and PSV) are also referred to as noninvasive positive pressure ventilation (NPPV).
Continuous Positive Airway Pressure
Continuous positive airway pressure (CPAP) is spontaneous breathing at a positive end-expiratory pressure, as illustrated in the upper panel of Figure 27.2. CPAP is simple in design, and requires only a source of oxygen and a face mask with an expiratory valve that maintains a positive end-expiratory pressure (i.e., a CPAP mask). The principal effect of CPAP is to increase the functional residual capacity (i.e., the volume in the lungs at the end of expiration). CPAP is usually set at 5–10 cm H2O.
LIMITATIONS: CPAP is a limited form of ventilatory support because it does not augment the tidal volume, and this limits its use in acute respiratory failure. The principal use of CPAP in acute respiratory failure is for patients with cardiogenic pulmonary edema, and the benefits of CPAP in this condition may be the result of hemodynamic support more than ventilatory support (see later).
Bilevel Positive Airway Pressure
Bilevel positive airway pressure (BiPAP) is CPAP that alternates between two pressure levels. This is illustrated in the lower panel of Figure 27.2. BIPAP is actually a variant of airway pressure release ventilation (APRV), which is shown in the middle panel of Figure 27.2. The only difference between BiPAP and APRV is the amount of time allotted for the high pressure level and the low pressure level; i.e., with APRV, most of the time is spent at the high pressure level, and with BiPAP, most of the time is spent at the low pressure level. The high pressure level in BiPAP is called the inspiratory positive airway pressure (IPAP), and the low pressure level is called the expiratory positive airway pressure (EPAP).
BiPAP results in higher mean airway pressures than CPAP, and this helps to promote alveolar recruitment. BIPAP does not directly augment tidal volumes, but the effect of BiPAP on alveolar recruitment will increase lung compliance (lung distensibility), and this will result in larger tidal volumes at the same changes in intrathoracic pressure . Therefore, BiPAP can indirectly enhance tidal volumes.
VENTILATOR SETTINGS: BiPAP (which requires a specialized ventilator) can be started with the following initial settings: IPAP = 10 cm H2O, EPAP = 5 cm H2O, inspiratory time (the duration of IPAP) = 3 sec. (The IPAP is added on to the EPAP, so an IPAP of 10 cm H2O with an EPAP of 5 cm H2O corresponds to a peak pressure of 15 cm H2O.) Further adjustments in pressure are determined by the resultant changes in gas exchange (i.e., PaO2/FIO2 ratio and PaCO2), and signs of respiratory distress (e.g., respiratory rate). Peak pressures above 20 cm H2O are not usually advised because they are poorly tolerated by patients, and also promote leaks.
Pressure Support Ventilation
Pressure support ventilation (PSV) is described in the last chapter (see pages 511–512). PSV provides patient-triggered inspirations and pressure-augmented tidal volumes. The inspiratory flow rate in PSV has a decelerating flow pattern, and the pressure-augmented tidal volume is terminated when the inspiratory flow rate decreases to 25% of the peak level (see Figure 26.3 on page 511). CPAP is usually combined with PSV to increase the functional residual capacity. The combination of tidal volume augmentation and resting lung volume augmentation makes PSV with CPAP the preferred method of noninvasive ventilation (with a few exceptions, described later).
VENTILATOR SETTINGS: PSV is usually initiated with an inflation pressure of 10 cm H2O and a CPAP level of 5 cm H2O. (The inflation pressure is added to the CPAP level, so an inflation pressure of 10 cm H2O with CPAP of 5 cm H2O results in a peak pressure of 15 cm H2O.) As described for BiPAP, further adjustments in pressures are determined by the resultant changes in gas exchange and signs of respiratory distress, but peak pressures above 20 cm H2O are not usually advised because they are poorly tolerated by patients, and promote leaks.
Patient Selection
Patient selection is the single most important factor in determining the success or failure of NIV (7,8). The patient selection criteria for NIV are presented as a checklist in Table 27.2.
1. The first step is to identify patients who might need ventilatory support. These patients have signs of respiratory distress (e.g., tachypnea, use of accessory muscles of respiration, abdominal paradox) plus either severe hypoxemia (PaO2/FIO2 <200) or hypercapnia (PaCO2 >45 mm Hg).
2. The next step is to identify patients who are candidates for NIV. While some causes of acute respiratory failure are more successfully managed with NIV than others (see later), all patients should be considered candidates for NIV if all of the following conditions are present: (a) the acute respiratory failure is not an immediate threat to life, (b) there is no life-threatening circulatory disorder (e.g., circulatory shock), (c) the patient is awake or arousable and cooperative, (d) airway protective mechanisms are intact (e.g., gag and cough reflexes), (e) there is no hematemesis or recurrent vomiting, (f) there is no facial anomaly that will prevent the use of a tight-fitting face mask (e.g., recent facial trauma), and (g) there is no obstruction that will prevent effective ventilatory support through a face mask (e.g., laryngeal edema).
3. Progression of the respiratory failure can limit the success of NIV (7,8), so there should be no delays in initiating NIV for appropriate candidates.
Table 27.2 Checklist for Noninvasive Ventilation
Efficacy
The success of NIV in avoiding endotracheal intubation varies in different clinical disorders, which can be organized according to the major blood gas abnormality associated with the respiratory failure; i.e., hypercapnia or hypoxemia.
Hypercapnic Respiratory Failure
ACUTE EXACERBATION OF COPD: The greatest benefit with NIV in acute respiratory failure is in patients with acute exacerbation of COPD and CO2 retention (9,10). The benefits of NIV in patients with acute exacerbation of COPD are demonstrated in Figure 27.3 (9). The use of NIV is associated with a marked decline in both the rate of tracheal intubation and the mortality rate. These results are corroborated in 14 clinical studies (10); as a result, noninvasive ventilation is considered a first-line therapy for acute exacerbations of COPD associated with hypercapnia (7,8). The preferred mode of ventilation in this condition is PSV with CPAP.
FIGURE 27.3 Impact of noninvasive ventilation (NIV) on rate of intubation and in-hospital mortality rate in patients with hypercapnic respiratory failure secondary to acute exacerbation of COPD. Patients assigned to NIV received pressure support ventilation for at least 6 hrs each day until intubation or recovery. N = number of patients in each study group. Data from Reference 9.
OBESITY HYPOVENTILATION SYNDROME: NIV reduces the severity of hypercapnia in outpatients with obesity hypoventilation syndrome (11) and, despite few studies in ICU patients, NIV is recommended as a routine measure for patients with obesity hypoventilation syndrome who are admitted with acute respiratory failure (12). Either CPAP or BiPAP can be used in this condition.
ASTHMA: NIV has not been adequately evaluated in status asthmaticus, but the available evidence shows that NIV hastens the resolution of the acute illness and decreases the length of stay in both the ICU and the hospital (13).
FIGURE 27.4 Failure rate of noninvasive ventilation for preventing endotracheal intubation in relation to the etiology of hypoxemic respiratory failure. CPE=cardiogenic pulmonary edema, Pulm Cont=pulmonary contusion, NP=nosocomial pneumonia, CAP=community-acquired pneumonia, ARDS=acute respiratory distress syndrome. Data from reference 14.
Hypoxemic Respiratory Failure
The failure rate of NIV for avoiding endotracheal intubation in disease states associated with hypoxemic respiratory failure is summarized in Figure 27.4 (14). The failure rate is lowest in patients with cardiogenic pulmonary edema, and highest in patients with community-acquired pneumonia and acute respiratory distress syndrome (ARDS).
CARDIOGENIC PULMONARY EDEMA: NIV is successful in reducing the need for intubation, and reducing the mortality rate, in a large majority of patients with cardiogenic pulmonary edema (15,16). Most of the experience in this condition has been with CPAP (at 10 cm H2O), but BiPAP produces equivalent results (17). The improved outcomes may be related to improved cardiac performance because NIV can increase cardiac output in patients with systolic heart failure (17). This effect is attributed to the afterload-reducing effects of positive intrathoracic pressure (see page 500).
ARDS: NIV has had limited success in patients with ARDS. The success rate is greater with PSV plus CPAP (17) than with CPAP alone (18), and success is more likely when ARDS has an extrapulmonary source (e.g., septicemia) (14). If NIV is attempted in patients with ARDS, the preferred mode is PSV with CPAP, while CPAP alone should be avoided (8).
Monitoring
The success or failure of NIV in any individual patient should not be predetermined by the etiology of the respiratory failure, but rather by the response of the patient in the first hour after the onset of NIV. This is illustrated in Figure 27.5 for patients with hypercapnic and hypoxemic respiratory failure (14, 19). Failure to improve gas exchange significantly after one hour of NIV is evidence that NIV is failing as a support modality; at this point, the appropriate course of action is immediate endotracheal intubation. Delaying intubation only invites problems (see A FINAL WORD).
FIGURE 27.5 Responses to the first hour of noninvasive ventilation for predicting success or failure in patients with hypercapnic and hypoxemic respiratory failure. Data from References 14 and 19.
Adverse Events
Adverse events during NIV include gastric distension, pressure ulcers on the bridge of the nose from tight-fitting masks, and nosocomial pneumonia.
Gastric Insufflation
The principal concern during NIV is gastric distension from insufflated gas. However, this does not seem to be a common problem, and studies measuring upper esophageal opening pressures indicate that pressures less than 30 cm H2O should not cause gastric insufflation (20). Although nasogastric tubes are commonly placed for gastric decompression during NIV, withholding nasogastric tubes is a safe practice in patients who do not develop abdominal distension during NIV (21).
Nosocomial Pneumonia
The application of positive pressure to the airways can retard mucociliary clearance and predispose to nosocomial pneumonia during NIV. In studies comparing NIV to endotracheal intubation, the incidence of nosocomial pneumonia during NIV was 8 – 10%, but this was less than half the incidence of nosocomial pneumonia during endotracheal intubation (19–22%) (22,23).
A FINAL WORD
Don’t Forget to Intubate
The rising popularity of noninvasive ventilation tends to overshadow the value of endotracheal intubation. The following simple rules about endotracheal intubation deserve mention.
Rule 1: Hesitation invites trouble. There is a tendency to rely on noninvasive ventilation and delay intubation as long as possible in the hopes that it will be unnecessary. However, delays in intubation create unnecessary dangers for the patient because emergency intubations in patients who are in extremis can be troublesome as well as dangerous. As soon as intubation becomes a serious consideration, you should intubate the patient and get control of the airway without delay.
Rule 2: Endotracheal intubation is not the ‘kiss of death’. The perception that “once on a ventilator, always on a ventilator” is a fallacy that should never influence the decision to intubate a patient for full ventilatory support. Being on a ventilator does not create ventilator dependence, having a severe cardiopulmonary or neuromuscular disease does.
REFERENCES
Rescue Modes of Ventilation
1. Pipeling MR, Fan E. Therapies for refractory hypoxemia in acute respiratory distress syndrome. JAMA 2010; 304:2521–2527.
2. Lachmann B. Open up the lung and keep the lung open. Intensive Care Med 1992; 18:319–321.
3. Stawicki SP, Goyal M, Sarini B. High-frequency oscillatory ventilation (HFOV) and airway pressure release ventilation (APRV): a practical guide. J Intens Care Med 2009; 24:215–229.
4. Sud S, Sud M, Freiedrich JO, et al. High frequency ventilation versus conventional ventilation for treatment of acute lung injury and acute respiratory distress syndrome. Cochrane Database Syst Rev 2013; Feb 28:CD004085.
5. Kallet RH. Patient-ventilator interaction during acute lung injury, and the role of spontaneous breathing: Part 2: airway pressure release ventilation. Respir Care 2011; 56:190–206.
6. Sydow M, Burchardi H, Ephraim E, et al. Long-term effects of two different ventilatory modes on oxygenation in acute lung injury. Comparison of airway pressure release ventilation and volume-controlled inverse ratio ventilation. Crit Care Med 1994; 149:1550–1556.
Noninvasive Ventilation
7. Hill NS, Brennan J, Garpestad E, Nava S. Noninvasive ventilation in acute respiratory failure. Crit Care Med 2007; 35:2402–2407.
8. Keenan SP, Sinuff T, Burns KEA, et al, as the Canadian Critical Care Trials Group /Canadian Critical Care Society Noninvasive Ventilation Guidelines Group. Clinical practice guidelines for the use of noninvasive positive-pressure ventilation and noninvasive continuous positive airway pressure in the acute care setting. Canad Med Assoc J 2011; 183:E195–E214.
9. Brochard L, Mancero J, Wysocki M, et al. Noninvasive ventilation for acute exacerbations of chronic obstructive pulmonary disease. N Engl J Med 1995; 333:817–822.
10. Ram FSF, Picot J, Lightowler J, Wedzicha JA. Non-invasive positive pressure ventilation for treatment of respiratory failure due to exacerbations of COPD. Cochrane Database Syst Rev 2009; July 8:CD004104.
11. Piper AJ, Wang D, Yee BJ, et al. Randomised trial of CPAP vs. bilevel support in the treatment of obesity hypoventilation syndrome without severe nocturnal desaturation. Thorax 2008; 63:395–401.
12. BaHamman A. Acute ventilatory failure complicating obesity hypoventilation: update on a ‘critical care syndrome’. Curr Opin Pulm Med 201l 16:543–551.
13. Gupta D, Nath A, Agarwal R, Behera D. A prospective randomised controlled trial on the efficacy of noninvasive ventilation in severe acute asthma. Respir Care 2010; 55:536–543.
14. Antonelli M, Conti G, Moro ML, et al. Predictors of failure of noninvasive positive pressure ventilation in patients with acute hypoxemic respiratory failure: a multi-center study. Intensive Care Med 2001; 27:1718–1728.
15. Masip J, Roque M, Sanchez B, et al. Noninvasive ventilation in cardiogenic pulmonary edema: systematic review and meta-analysis. JAMA 2005; 294: 3124–3130.
16. Vital FM, Saconato H, Ladeira MT, et al. Non-invasive positive pressure ventilation(CPAP or bilevel NPPV) for cardiogenic pulmonary edema. Cochrane Database Syst Rev 2008; July 16:CD005351.
17. Acosta B, DiBenedetto R, Rahimi A, et al. Hemodynamic effects of noninvasive bilevel positive airway pressure on patients with chronic congestive heart failure with systolic dysfunction. Chest 2000; 118:1004–1009.
18. Delclaux C, L’Her E, Alberti C, et al. Treatment of acute hypoxemic nonhypercapnic respiratory insufficiency with continuous positive airway pressure delivered by a face mask. JAMA 2000; 284:2352–2360.
19. Anton A, Guell R, Gomez J, et al. Predicting the result of noninvasive ventilation in severe acute exacerbations of patients with chronic airflow limitation. Chest 2000; 117:828–833.
20. Wenans CS. The pharyngoesophageal closure mechanism: a manometric study. Gastroenterology 1972; 63:769–777.
21. Meduri GU, Fox RC, Abou-shala N, et al. Noninvasive mechanical ventilation via face mask in patients with acute respiratory failure who refused endotracheal intubation. Crit Care Med 1994; 22:1584–1590.
22. Girou E, Schotgen F, Delclaux C, et al. Association of noninvasive ventilation with nosocomial infections and survival in critically ill patients. JAMA 200; 284:2361–2367.
23. Carlucci A, Richard J-C, Wysocki M, et al. Noninvasive versus conventional mechanical ventilation: an epidemiological study. Am J Respir Crit Care Med 2001; 163:874–880.

Full access? Get Clinical Tree
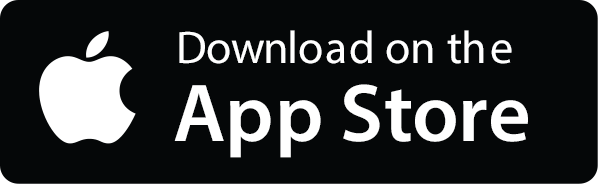
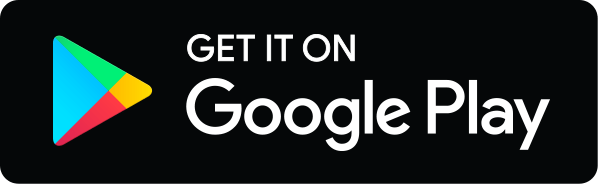