CHAPTER 22. Cardiovascular Emergencies
Christopher Manacci and Stephanie L. Steiner
Competencies
Get Clinical Tree app for offline access
1. Perform a detailed cardiovascular assessment before, during, and after transport.
2. Recognize potential for lethal events, and institute appropriate interventions and therapeutic modalities.
3. Identify patients with acute cardiac events, including acute myocardial infarction, heart failure, cardiogenic shock, primary arrhythmias, and hemodynamic instability.
4. Use invasive monitoring during transport, as indicated, for the purpose of clinical management.
5. Provide treatment for patients with acute cardiac events and hemodynamic abnormalities.
One of the most challenging aspects for the transport team is the care of critically ill patients who need medical transport because of an acute cardiovascular event. The demand for medical transport of patients who are dependent on invasive devices and new sophisticated technology continues to increase. 33 This chapter describes advances in clinical care for patients with angina, acute myocardial infarction (AMI), congestive heart failure (CHF), aortic dissection, and other manifestations of cardiovascular disease, with a focus on assessment and management relative to the medical transport environment.
Cardiovascular disease is the leading cause of death in adults in the United States, accounting for about one third of all deaths in subjects over age 35 years. 49 In 2005, cardiovascular disease was responsible for approximately 4,036,000 emergency department visits. Cardiovascular disease is estimated to cost the healthcare system 448.5 billion dollars each year. 2 Recent data suggest that 13.7 million persons in the United States have coronary heart disease; half have myocardial infarctions (MIs), and half have angina. 49 Each day, 2400 deaths occur from cardiovascular disease alone, with one death every 37 seconds. 3 Cardiovascular disease, including hypertension, coronary artery disease (CAD), rheumatic heart disease, and stroke, affects more than 80 million Americans. 2,21
A patient with an acute cardiac event, decompensated CHF, or an aortic dissection in a community-based limited-resource hospital often needs rapid critical care transfer to a tertiary care facility for further evaluation and emergent intervention. The transport of critically ill cardiovascular patients involves a number of issues, which include but are not limited to the potential effects of altitude and the difficulty associated with initiation of resuscitative interventions in a limited space in an uncontrolled environment.
Early research during the 1980s showed the safety and efficacy of interfacility air medical transport of patients with evolving MI who had undergone thrombolytic therapy under the care of a critical care clinician. 4,46 Current research has established the safe transport of patients who need advanced mechanical circulatory support. Interfacility transfer by air and surface critical care teams of patients dependent on intraaortic balloon pumps (IABPs), ventricular assist devices (VADs), and extracorporeal membrane oxygenation (ECMO) can now be accomplished. 23 Previous logistic restrictions, primarily because of the size of these devices and a lack of familiarity by crew members with this new technology, did not permit routine use of these circulatory support devices during transport.
ALTERATIONS OF CARDIOVASCULAR PHYSIOLOGY AT HIGH ALTITUDES
The potential for hypoxia poses one of the greatest risks to a patient with coronary artery disease. Decompensation of patients with acute cardiovascular disease during transport at high altitudes is generally caused by hypoxic hypoxia, which is defined as an oxygen deficiency in the body tissue sufficient to cause impaired function. 9,22 Individual patient tolerances vary, but the patient with cardiovascular compromise may be at risk if cabin pressure is above 6000 ft. For example, at an altitude of 10,000 ft, the barometric pressure decreases from 760 mm Hg at sea level to 523 mm Hg, which results in a lower partial pressure of oxygen. This reduction in the amount of oxygen in the blood decreases available oxygen to the tissues. As altitude increases, oxygen saturation decreases. If a patient has 98% saturation (PaO 2, 103) at sea level, they have 93% saturation (PaO 2, 68.9) at 8000 ft (the level at which commercial airlines routinely pressurize cabins). 9,22
Healthy individuals can easily tolerate the effects of altitude without supplemental oxygen, but the patient with cardiovascular compromise can have clinical manifestations develop related to cellular hypoxia with even a change from 0 to 4000 ft. Compensatory mechanisms that occur to maintain adequate oxygen supply to the tissues include increases in respiratory rate, heart rate, and cardiac output. The increased workload on the heart increases myocardial oxygen consumption and necessitates increased blood flow to the heart muscle. In healthy people, cardiac reserve allows the body to compensate and meet the demand for increased blood flow to the tissues by altering heart rate, stroke volume, or both and increases blood flow to the heart muscle by dilating the coronary artery microvasculature. Cardiovascular heart disease and cardiac events limit the compensatory mechanisms available to increase cardiac output in response to increased myocardial oxygen demand. Patients with coronary artery disease may be unable to compensate for the increased workload imposed on the heart by the decreased oxygen tension experienced at high altitude. These patients may have development of chest pain, congestive heart failure, pulmonary edema, cardiac arrhythmias, or even cardiac arrest. 9,14,22
Patients in cardiogenic shock on sodium nitroprusside therapy for afterload reduction and susceptible patients on nitroglycerin therapy can have development of histotoxic hypoxia because of the potential for cyanide toxicity. Carbon monoxide, cyanide, or alcohol intake can all cause histotoxic hypoxia, which results in the inability of the body to use available oxygen because of poisoning of the cytochrome oxidase enzyme system. Tissue hypoxia occurs because of the formation of methemoglobin (which has increased oxygen affinity when compared with normal hemoglobin); therefore, the ability for oxygen to be unloaded at the tissue level is decreased. This situation can be made worse in the presence of a lower partial pressure of oxygen caused by high altitudes. 9,15,22,25 A patient with adequate available oxygen, who is profoundly anemic, may have such a low hemoglobin concentration that oxygen-carrying capability is reduced to the point that tissue hypoxia develops. This condition is referred to as hypemic hypoxia, which, again, can be aggravated by the low partial pressure of oxygen that can develop with increases in altitude. This situation is of special interest to the clinician transporting a patient with a recently placed VAD because of the potential for blood loss during surgical insertion. The hemoglobin concentration in these patients must be evaluated and transfused if necessary before departure from the referral institution. 9,22,25
All patients with cardiovascular disease should receive supplemental oxygen when transported via air. The potential effects of altitude can be minimized if the aircraft is pressurized at or below 4000 ft and supplemental oxygen is delivered. In fixed-wing transport, limiting cabin altitude to a maximum of 6000 ft has been shown to eliminate problems for patients with cardiovascular disease. Cabin pressure is not a fixed variable and may need to be adjusted to accommodate patient condition. Cabin pressure can be adjusted to as low as sea level; however, a decrease in cabin pressure may come at the expense of travel at a lower altitude. Flights at decreased altitude may result in decreased speeds and therefore longer flights with an increased need for refueling stops during the trip.
Decisions regarding altitude restrictions or limitations must be based on patient history, current clinical condition, and pilot-in-command expertise and judgment. These decisions need to be evaluated throughout the mission and may need to be adjusted as a result of continued in-flight patient surveillance. 9,25
SPECIAL CONSIDERATIONS FOR CARDIOPULMONARY RESUSCITATION IN THE TRANSPORT ENVIRONMENT
Cardiac arrest is of special concern to nurses involved in the transport of critical patients via either air or surface because CPR in the confined space of an aircraft cabin or ground transport vehicle often can be both difficult and challenging. The advanced cardiac life support (ACLS) guidelines are the standard of practice for resuscitation of the patient in cardiac arrest and should be used as such in the event of cardiac arrest in the transport environment. Transport team members are expected to have current verification in ACLS through the American Heart Association to participate in patient care within the transport environment. 24,25 Thorough pretransport assessment, planning, and intervention along with prompt correction of dysrhythmias, repletion of electrolytes, and continuous maintenance of adequate oxygenation may help to prevent the need for in-transport resuscitation.
However, the transport team must maintain a state of perpetual readiness for emergencies such as cardiac arrest. Preparation includes ensuring that resuscitation equipment and an adequate oxygen supply are easily accessible. ACLS drugs should be well labeled, not beyond the expiration date, and ready for quick administration. Generally, the number of crew members available to perform basic and advanced life support resuscitation is limited to only two medically trained personnel. For effective and rapid response in the event of an emergency, these team members must establish well-defined roles and responsibilities.
Special consideration should be given to transport vehicle configuration in anticipation of the potential need for CPR during admission. The position and height of the stretcher in relationship to the medical crew’s ability to change positions is critical to facilitate proper hand and arm positioning in chest compressions. A well-designed configuration minimizes the need for crew members to extend or release restraint devices in administration of therapeutic interventions. 25
Defibrillation during Transport
The most critical factor in determination of the success of a resuscitative effort is the time to restoration of effective spontaneous circulation. Immediate defibrillation is certainly the priority in the treatment of confirmed ventricular fibrillation and unstable ventricular tachycardia. The close quarters, metallic composition of transport vehicles, and proximity of vital electronic equipment, particularly in the rotor-wing environment, previously generated concern among transport personnel about safely defibrillation in this environment. Holleran25 addressed the potential electrical risks of airborne defibrillation and showed that defibrillation with modern equipment in a medically equipped twin-engine helicopter is safe. Despite cramped quarters and sensitive electrical equipment, defibrillation can be carried out without hesitation, whether the aircraft is on the ground or in flight, provided that standard defibrillation precautions are observed. Air medical personnel should follow the ACLS defibrillation standards for selection of energy levels and use of self-adhesive monitor/defibrillation pads for the transport environment. The crew must inform the pilot before defibrillation and maintain clearance from the patient and stretcher when discharging the current.
CORONARY ARTERY DISEASE
Definition and Pathophysiologic Factors
Coronary artery disease remains one of the most common health problems in Western civilization and is directly responsible for approximately 50% of all deaths annually in the United States. 2 Progressive atherosclerosis of the coronary arteries results in a reduction of blood flow to the myocardium. Risk factors for coronary artery disease (i.e., diabetes, hypertension, cigarette smoking, high levels of low-density lipoprotein [LDL], and low levels of high-density lipoprotein [HDL]) contribute to the formation of atherosclerotic plaques by injuring or interfering with the normal function of the vascular endothelium. The response-to-injury theory8,15 of the development of atherosclerosis suggests injury to the endothelium and the subintimal accumulation of lipids. Chronic intimal injury encourages the deposit of lipids in the wall of the coronary artery, resulting in lipid-rich plaques with a fibrous cap. 8,15,31,40 The atherosclerotic plaque increases over time and at varying rates, depending on the degree of vascular injury and risk factors present. An inflammatory process can be appreciated along the vessel wall with propagation of plaque formation and risk of shearing of the plaque. Acute coronary syndromes appear to be caused by rupture of an unstable coronary plaque that appears as a single lesion on angiography. However, increasing evidence shows that systemic effects, such as inflammation, are more widespread within the coronary circulation and lead to instability of multiple plaques. Significant obstruction to flow, as shown by coronary ischemia, occurs when the coronary arteries are narrowed by approximately 70%. At this point, myocardial oxygen blood supply may not be adequate to meet myocardial oxygen demand at times of stress, and ischemia of the myocardium develops. 10,15,31
An area of the ventricular myocardium that becomes ischemic develops abnormal contractility almost immediately at the onset of ischemia, which results in decreased left ventricular function. 19 Although ischemic, the electrical homeostasis of the heart is altered, and life-threatening ventricular arrhythmias can occur.
Pathophysiologic Factors of Coronary Ischemia
Myocardial metabolism is an aerobic process in which the heart extracts about 70% of the available oxygen supplied by the coronary arteries. Increases in myocardial oxygen demand are normally met by increases in blood flow caused by dilation of the microcirculation system of the coronary arteries.
Angina pectoris or angina is a symptom of myocardial ischemia. It is caused by an imbalance between myocardial oxygen supply and demand. The end result is a buildup of metabolites in the ischemic tissue, which activates nerve endings and causes anginal pain. 15
The myocardial oxygen supply and demand relationship is the basis for understanding both the causes of and the treatment for coronary ischemia. Myocardial oxygen supply is dependent primarily on patent coronary arteries and an adequate hemoglobin concentration to ensure adequate oxygen-carrying capacity. The major determinants of myocardial oxygen demand are heart rate, contractility, and wall stress. The imbalance between myocardial supply and demand can occur for a number of reasons:
1. Conditions that decrease supply:
Decrease in the caliber of a coronary artery.
Fixed (atherosclerosis).
Transient (spasm).
Severe anemia.
Poor perfusion of the coronaries because of profound hypotension.
2. Conditions that increase demand:
Tachycardia.
Hypertension that results in increased left ventricular wall stress.
Increased myocardial contractility caused by increased adrenergic stimuli, fever, or vasopressor agents.
Angina can be divided into several categories depending on the onset, severity, duration, and alleviation of symptoms. These categories include classic or stable angina, unstable angina, Prinzmetal’s angina, or silent angina (more commonly termed silent ischemia).
Chronic Stable Angina
In the presence of a flow-limiting coronary artery atherosclerotic lesion, myocardial oxygen supply may be inadequate to meet periods of increased myocardial oxygen demand. When a patient with a significant atherosclerotic lesion undertakes an action that increases myocardial demand (such as walking on a treadmill), the patient begins to show signs of myocardial ischemia (angina with ST segment changes) when the myocardial oxygen demand begins to outstrip supply. This is the basis of the cardiac stress test to diagnose coronary artery disease.
Stable angina is usually precipitated by physical exertion or emotional stress. Chronic stable angina is predictable, given a measured work load. These symptoms usually last 1 to 5 minutes and are often abated with stopping the exertion activity or with the use of sublingual nitroglycerine (NTG). 10,26
Unstable Angina
Unstable angina is a clinical syndrome and not a specific disease. Unstable angina is routinely classified with clinical descriptors, such as the presence of chest discomfort at rest. 13 The term unstable angina refers to an abrupt change in a patient’s anginal symptoms. Unstable syndromes include angina with minimal exertion or angina with an exertion level that is much less than usual, new-onset angina, or angina at rest. The abrupt change in symptom pattern can occur as a result of any mechanism that suddenly increases myocardial oxygen demand (tachycardia from pain, fever, hypovolemia, profound hypertension, or thyrotoxicosis, or a sudden decrease in myocardial oxygen supply. This change includes a sudden decrease in the caliber of a coronary from progression of an atherosclerotic plaque or coronary spasm or anemia from acute bleeding. These symptoms and the ischemic electrocardiographic (ECG) changes that are associated with them are managed with treatment of the underlying problem.
The most common cause of a sudden decrease in myocardial perfusion is transient thrombus formation on the surface on a ruptured atherosclerotic plaque. Unstable angina, which is caused by an abrupt decrease in the caliber of a coronary artery, falls into the category of acute coronary syndromes, which are discussed extensively in a later section of this chapter.
Prinzmetal’s angina, or variant angina, is a form of angina in which spontaneous chest discomfort with ST segment elevation occurs without relationship to exertion. Prinzmetal characterized it in 1959 as the result of temporary increased tone in a coronary artery resulting in a marked reduction in coronary blood flow. Evidence supports focal coronary spasm as the mechanism of transient ST segment elevation and concurrent symptoms. 27 Spasm is usually confined to a single epicardial vessel, but multivessel spasm can occur. 29 Spasm generally occurs in 1 centimeter of an atherosclerotic plaque in a mildly diseased vessel. Patients are generally young and often do not exhibit the classic atherosclerotic risk factors except for cigarette smoking. A circadian rhythm is often seen with anginal attacks, which are clustered in the early morning hours. During the period of time in which the coronary artery is in spasm, these patients are at risk for myocardial necrosis and the life-threatening arrhythmias that are associated with coronary ischemia. The pathophysiology of this disease appears to involve a combination of endothelial dysfunction and increased reactivity in response to vasoconstrictor substances. Treatment includes the use of vasodilator substances, such as NTG and calcium channel blockers, and risk factor modification. 10,29,43
Silent Ischemia
Evidence of ischemia in the absence of symptoms occurs frequently. Upwards of 75% of ischemia in patients with stable angina is silent, or without clinical manifestations. 15 ST segment changes are often detected on ambulatory monitoring. Several explanations exist for the absence of anginal symptoms in patients with silent ischemia, including the observation that patients with this silent angina have less sensitivity to pain in general than do patients with more traditional angina.
Continuum of Acute Coronary Syndromes
An abrupt change in the caliber of a coronary artery can occur as a result of coronary blood vessel spasm or the abrupt worsening of an atherosclerotic plaque. Atherosclerotic plaques can suddenly become more narrowed because of the formation of atheroma in the wall of the vessel or the formation of a thrombus on the surface of a damaged plaque. 8
Atherosclerotic lesions, which are rich in lipids, are susceptible to endothelial damage. Disruption of the protective endothelial lining, known as plaque rupture, leads to a cascade of processes, which are aimed at healing this damaged vessel. If these processes become overexuberant, a thrombus can form on the surface of the damaged endothelium, which results in the total occlusion of the blood vessel. 28,40
Rupture of vulnerable plaques is responsible for 75% of all acute coronary syndromes and results in acute obstruction in blood flow to the myocardium normally served by the occluded coronary artery. 40 Acute coronary syndromes begin with a disruption of the endothelium overlying an atherosclerotic lesion. The lesions more likely to be involved in acute coronary syndromes are the mild to moderate lesions with thin fibrous tissue caps; larger lipid concentrations of the underlying coronary vessel wall causes both platelet activation and the stimulation of the clotting cascade to form thrombin. Thrombin is a potent stimulant of platelet activation and is responsible for the conversion of fibrinogen in the bloodstream to fibrin. The newly formed fibrin is responsible for cross linking of platelets into a stable thrombus.
Platelets, once activated, bind to exposed areas of the vessel wall. More platelets are drawn to the area, and the activated platelets begin to bind to each other via receptors on their surface, known as glycoprotein IIb/IIIa receptors. These platelets eventually form a platelet plug. If large enough, they can completely occlude the relatively small (3-mm range) coronary arteries and occlude flow in the vessel. Platelet activation also leads to the secretion of vasoconstriction substances, which increases limited blood flow in the affected coronary artery. 18
The degree to which a coronary artery is occluded and whether or not it remains occluded by this process subsequently determines in which part of the continuum of acute coronary syndromes an event is classified. For example:
1. Unstable angina caused by an acute change in the caliber of a coronary artery as a result of plaque rupture and thrombus formation. If transient occlusion of a coronary by activated platelets at the site of a ruptured plaque occurs with subsequent recanalization of the vessel, the patient may be symptomatic at rest (while the coronary is occluded) and have symptoms resolve spontaneously with recanalization of the vessel. Transient ECG changes may occur while the patient is symptomatic, but the episode is often too short to show either ECG changes or evidence of myocardial injury.
2. An event classified as a non–Q wave infarction begins in the same manner, but either occlusion of the coronary is prolonged (with myocardial necrosis) or distal embolization of small platelet clumps occurs, leading to occlusion of smaller distal coronary branches and therefore myocardial necrosis. Spontaneous recanalization may occur, but biochemical evidence of myocardial necrosis is found (troponin, CPKDMB).
In both of these instances, a significantly narrowed coronary artery (although recanalized) remains, and therapy is aimed at keeping the activated platelets present on its surface from progressing into a stable thrombus. In the patient with symptoms that resolve with therapy, the question of further management becomes less clear. 11 The data support the use of either of the following strategies:
1. Conservative management: Use of cardiac catheterization with subsequent revascularization only for patients with recurrent symptoms of angina or positive stress test results.
2. Invasive management: Performance of a cardiac catheterization with subsequent revascularization in all patients with documented acute coronary syndromes.
Acute MI represents the syndrome of acute plaque rupture, which continues to its ultimate end point, an organized thrombus made up of activated platelets with cross-linked fibrin, which results in a stable thrombus. These patients have continued chest pain and associated ST segment elevation because of injury caused by total occlusion of coronary blood flow. At this point, therapy must be aimed at immediate reperfusion.
Chronic Stable Angina: Assessment, Diagnosis, and Treatment
Classic angina is usually described as substernal chest discomfort, pressure, or heaviness that occurs with activity and is resolved with rest. The chest discomfort usually increases in intensity and often gradually self resolves. Occasionally, patients may report dyspnea, back, shoulder, arm, or jaw pain without the presence of chest pain. The location of the chest pain is most commonly in the middle or lower sternum or the left precordium. Patients may also have such symptoms as nausea, vomiting, diaphoresis, dyspnea, and fatigue, but in general, these symptoms are predictable and patients know their limitations. The patients usually stop exerting themselves or take NTG tablets before the symptoms become severe.
Physical examination results in patients with episodes of chronic stable angina are often normal, although patients could have an S4 because the ischemic area of the left ventricle is stiff. 15,19
In stable angina, the ECG has limited diagnostic value. Transient ST depression may occur during exertion; it represents ischemia in the subendocardium, the inner surface of the myocardium. This area of the ventricular myocardium, just adjacent to the left ventricular cavity and farthest away from the oxygen rich blood supplied from the coronary arteries, is the most susceptible to ischemia. Cardiac arrhythmias, some potentially lethal, may occur with any episode of ischemia caused by alterations in the conductance of electrical activity through the myocardium at times of limited coronary blood flow.
The goal of treatment of chronic stable angina, as with all forms of angina, is identification of the imbalance in the myocardial oxygen supply and demand relationship and subsequent fix of the causative problem. Treatment involves strategies to either increase coronary blood supply or decrease myocardial oxygen demand, or both. This treatment may be accomplished through pharmacology, percutaneous angioplasty, or surgical interventions. 17
Medical therapy for chronic stable angina is directed towards decreasing myocardial oxygen demand by controlling heart rate and blood pressure. Therapy includes beta-blockers, angiotensin-converting enzyme (ACE) inhibitors, transdermal or oral nitrates, and calcium channel blockers. Medical therapy is also aimed at risk factor modification, including smoking cessation and aggressive lipid lowering.
Nitroglycerin can be used in both short-acting and long-acting forms. Sublingual NTG is often used for the initial management of anginal pain and ischemia. It works by relaxing vascular smooth muscle, which leads to both arterial and venous vasodilation. Venodilation leads to a decrease in preload and therefore a decrease in ventricular wall tension. Arterial vasodilation leads to a decrease in systemic blood pressure. These actions both work to decrease myocardial oxygen consumption or demand. Coronary vasodilation, both epicardial and microvasculature, can help to increase myocardial oxygen supply. Patients who use long-acting nitrates should be warned of the tolerance effect with this medication and should have at least an 8-hour drug-free window to maintain drug efficacy. Patients often use sublingual NTG as prophylaxis before an activity that is known to precipitate an anginal attack.
Beta-blockers work by decreasing myocardial oxygen demand. These agents interrupt sympathetic impulses by competing with the neurotransmitter norepinephrine at the beta-sympathetic nerve endings. Beta-receptor inhibition results in decreased heart rate, myocardial contractility, and slowed impulse transmission through the cardiac conduction system. These effects lead to decreased myocardial oxygen consumption. In addition, beta-blockers decrease MI size and improve survival rates because of a decreased incidence of myocardial rupture and ventricular fibrillation. 17
The calcium channel blockers verapamil and diltiazem slow heart rate, decrease blood pressure, and decrease myocardial contractility, with each of these contributing to a decrease in myocardial oxygen consumption. Although previously widely used for the management of angina, calcium channel antagonist use has decreased since controversial data were presented in 1995.
Beta-blockers should be used as first-line agents unless contraindicated, and calcium channel blocking agents can be added as an alternative or additional therapy if the maximal doses of beta-blockers have been achieved. 17,34
All patients with coronary artery disease should take 81 mg of aspirin each day34 and follow a lifestyle that promotes cardiovascular health. In patients with elevated cholesterol levels, a statin-type lipid-lowering agent should be used to achieve an LDL of less than 100 mg/dL. 34
Percutaneous transluminal coronary angioplasty, with or without intracoronary stenting, can be performed in patients with symptomatic angina, which indicates ischemia in the territory of a significantly narrowed coronary artery. In patients with coronary artery disease that cannot be rescued with angioplasty, coronary artery bypass surgery may be needed to revascularize the entire myocardium. Again, aggressive risk factor modification in addition to interventional therapy is indicated. 41
Patients with chronic stable angina often come into contact with transport personnel when they are faced with a sudden stressful situation not necessarily directly related to a cardiac event. Patients with significant coronary artery disease may be seen in the setting of a trauma, gastrointestinal bleed, or sepsis. The key to the evaluation and treatment of these patients is to ask the question “What is the underlying problem?” A patient with chest pain and ST segment changes in the setting of a broken pelvis and a hematocrit of 22% has symptoms caused by an increased myocardial oxygen demand, which therefore may provoke cardiac symptoms. This is again is from the increased demand on the myocardium and not necessarily a flow or supply issue. However, the true mechanism of what physiologically took place first is never truly known in this situation, and transport personnel should maintain a high suspicion. In the example used previously, no change has been seen in the caliber of the coronaries, therefore treatment should not be aimed at antiplatelet and anticoagulation therapy. The patient has stress caused by hypovolemia, anemia, and pain, which all lead to increased heart rate and therefore increased myocardial oxygen demand. The goal is correction of the underlying problem and accurate evaluation of the causes of the patient’s clinical presentation, and therefore correction of the causative problem with transfusion, volume replacement, and pain control if indicated.
Acute Coronary Syndromes: Diagnosis, Assessment, and Treatment
The term acute coronary syndrome is applied to an abrupt change in anginal symptoms caused by the sequelae of a ruptured atherosclerotic plaque. The continuum of unstable angina, non–Q wave infarction, and AMIs is, in truth, a varying degree of the same underlying problem: a mild-to-moderate lipid-laden atherosclerotic plaque that suddenly ruptures exposing the underlying cholesterol gruel to the circulating blood and setting in place multiple mechanisms that are responsible for trying to repair the damaged vessel wall. Platelets cover the surface of the injured area and then attach to each other by receptors on their surfaces called GP IIb/IIIa receptors. The activation of platelets during this process releases regulatory substances, which cause further aggregation of platelets and vasoconstriction. At the same time, the clotting cascade becomes activated, which results in the formation of thrombin, a potent stimulator of platelets. Thrombin is also responsible for the formation of fibrin from fibrinogen in the blood. As platelets accumulate in the area, a platelet plug forms, which itself can intermittently occlude flow in the coronary. As this mass of platelets becomes organized, fibrin interconnects the platelets into a stable blood clot, or thrombus. 28
Once platelets have aggregated on the surface of ruptured plaque, the competition between antithrombotic and thrombotic processes in the body becomes intense. The vessel may reocclude at any time, or this area may heal without further symptoms, and the ruptured plaque/platelet plug may be incorporated into the atherosclerotic plaque, contributing to the progressive growth of atherosclerotic coronary artery disease. The goal of treatment in acute coronary syndromes is to augment the anticoagulant properties that the body possesses and interfere with the clot forming processes.
Acute coronary syndromes begin primarily as a process mediated by activated platelets; therefore, therapeutic strategies are primarily directed toward the inhibition of platelets and interference with platelet-to-platelet interactions.
Efforts aimed at decreasing myocardial oxygen demand, such as beta-blockers, pain control, and NTG, also help but do not address the primary problem of activated platelets on the surface of a ruptured plaque.
Aspirin is a potent inhibitor of thromboxane, a stimulated platelet aggregation. Many other pathways stimulate platelet aggregation and interactions. Aspirin has clearly been shown to decrease MI and death in patients who present to the hospital with unstable angina. All patients with symptoms consistent with unstable angina and without contraindications should receive at least 160 mg of aspirin. 1
Ticlopidine and clopidogrel bisulfate inhibit platelet activation by inhibiting ADP-dependent platelet activation. This effect does not occur immediately; there is a risk of neutropenia with their use, and they should be used with caution. These drugs are primarily used in patients with true aspirin allergies and as an adjunct to anticoagulation therapy after intracoronary stent placement.
Heparin is a potent anticoagulant that augments the body’s ability to reduce thrombin generation and fibrin formation. Heparin does not dissolve a clot that has already formed; however, it does halt the propagation of the clot or any new clots. Clinical evidence supports the use of heparin in acute coronary syndromes. The transport team should follow recommended protocols.
The glycoprotein IIb/IIIa receptors on the surface of platelets are responsible for the attachment of platelets to one another. Drugs that inhibit this process block the common final pathway of platelet aggregation and are therefore inhibitors of the formation of thrombus. The agents currently in use, such as abciximab, eptifibatide, and tirofiban, all have different mechanisms of actions, dosing strategies, and half-lives. A review of these drugs is not included here because it is beyond the intended scope of this chapter. These medications, which have become an important part of the treatment of patients with acute coronary syndromes, should be familiar to transport personnel. Current American Heart Association (AHA) guidelines recommend the use of GP IIb/IIIa inhibitors in addition to aspirin and heparin for patients with a non–ST segment elevation MI or refractory ischemia. In most patients, anticoagulation and antiplatelet therapies cause improvement of symptoms and signs of ischemia. For those patients with ongoing signs and symptoms of ischemia not relieved with medical therapy, cardiac catheterization with subsequent revascularization should be performed without delay. Depending on the patient’s coronary anatomy, revascularization may include angioplasty with or without intracoronary stent placement or coronary artery bypass graft surgery. 1,34
Acute Myocardial Infarction: Assessment, Diagnosis, and Treatment
An acute myocardial infarction occurs as a result of a coronary artery occluding from a thrombus forming on the surface of a ruptured atherosclerotic plaque. When flow no longer exists in the coronary artery, the entire distribution of that coronary artery is at risk for injury or myocardial cell death. Initially, that wall of the heart becomes stiff and then stops moving, which results in a loss of left ventricular ejection fraction and potentially leads to significant valvular dysfunction and ventricular arrhythmias. The longer the interruption to coronary flow, the more extensive the injury resulting in decrease of myocardial function.
As with the other acute coronary syndromes, an AMI begins as a platelet problem but evolves into a process that ends in the formation of a thrombus through the formation and infiltration of the platelet plug by fibrin cross-links. The initial treatment goals are therefore similar to those for other acute coronary syndromes. The use of aspirin, heparin, and GP IIb/IIIa inhibitors in addition to a definitive reperfusion strategy, such as emergent angioplasty, are the basic concepts of AMI therapy. Fibrinolytic therapy destroys fibrin in the intracoronary thrombus. In doing so, activated platelets are released from the thrombus. These activated platelets can reassemble and reocclude the vessel if adjunctive anticoagulation and antiplatelet strategies are not used. Agents such as beta-blockers, NTG, and morphine sulfate for pain control are important to decrease myocardial oxygen demand; reperfusion with definitive restoration of myocardial oxygen supply should be the primary goal. 12
Diagnosis of Acute Myocardial Infarction
Patients who present with an AMI describe chest heaviness, discomfort, or pressure, often associated with shortness of breath, diaphoresis, or nausea. The discomfort may radiate to the neck, jaw, or arms. Ongoing angina associated with electrocardiographic results that show ST segment elevation in contiguous leads (leads that represent an area of the heart that is supplied by a single coronary), known as an acute injury pattern, together make the diagnosis of an AMI. ST segment elevation on the electrocardiogram represents ischemia, injury and subsequent myocardial cell necrosis in the area of the occluded coronary artery. 1,34
The cardiac blood supply is made up of three principal coronary arteries. These vessels, the first branches off the aorta, originate from the coronary ostia at the level of the aortic valve cusps. The left main coronary artery divides into the left anterior descending (LAD) and the left circumflex arteries. The LAD supplies the anterior surface of the heart, the anterior two thirds of the septum, and part of the lateral wall. The LAD distribution is represented on the surface electrocardiogram in the V or chest leads. The circumflex coronary artery supplies branches to the lateral and posterior surfaces of the heart. The circumflex is not well represented on the standard electrocardiogram. Changes caused by ischemia or infarction in the circumflex coronary artery may be seen in the lateral (V5, V6, aVL, and I) leads or in the posterior wall by inference from changes in the V1 and V2 leads, which appear as ST segment depression that is actually elevation on the posterior surface of the heart.
In 85% of patients, the right coronary artery (RCA) is responsible for coronary blood flow to the inferior surface of the heart and to the posterior third of the interventricular septum by way of one of its branches, the posterior descending coronary artery. These areas of the heart are represented on the electrocardiogram as the inferior leads or leads II, III, and aVF. On the way to the inferior surface of the heart, the RCA is also responsible for supplying blood flow to the right ventricle.
Once the diagnosis of an AMI is clear, it should immediately prompt the decision of reperfusion therapy with rescue angioplasty and associated pharmacologic therapy. This decision must be made in a rapid fashion because for each minute the myocardium is devoid of blood flow, more injury occurs. The sooner an occluded coronary artery is reperfused, the less likely the patient is to die of an MI. 1
Examination of the patient suspected of having an AMI should be focused and include evaluation for contraindications to therapy and a search for complications of the MI. Excessive sympathetic stimulation may result in an elevated blood pressure and an increased heart rate.
The patient should be evaluated for the presence of an S3, rales, or distended neck veins, which indicate the presence of congestive heart failure caused by either severe left ventricular failure from the AMI or flash pulmonary edema resulting from the acute onset of severe mitral regurgitation. 15
Mitral regurgitation caused by the abrupt disruption of the mitral valve is often not audible on examination. It occurs as a consequence of occlusion of the blood supply to the lateral surface of the heart. Mitral regurgitation may also be related to a mechanical defect of the valve caused by untreated hypertension in some individuals. This may predispose the patient to acute onset of mitral regurgitation consequential to chordate rupture. Tachycardia and hypotension in association with the signs of congestive heart failure or pulmonary edema represent cardiogenic shock. These patients are best treated with emergent angioplasty, ideally after temporary placement of an intraaortic balloon pump. The intraaortic balloon pump is a counterpulsation therapy and assists in increasing both coronary perfusion and afterload reduction. 48
These patients in cardiogenic shock must not receive beta-blockers; the tachycardia is a functional compensatory mechanism because of the severely limited stroke volume from an extensive MI.
Critical care transport personnel are involved in the transport of patients with cardiogenic shock to facilities that have the capabilities to perform emergency angioplasty. In patients transported before the placement of an intraaortic balloon pump, perfusion to the brain and vital organs must be maintained. The use of low-dose phenylephrine or norepinephrine to maintain blood pressure, albeit at a cost of increased myocardial oxygen demand, should be used. Dopamine is common; however, caution should be used because it not only increases myocardial depend but also the potential of tachyarrhythmias. These medications should be used at the lowest dose possible to maintain adequate perfusion of the vital organs, which can normally be achieved by a mean arterial pressure (MAP) of 65 to 70 mm Hg. Evidence of adequate perfusion includes not only adequate systolic blood pressure (SBP) but a patient who is conscious and making urine. However, ensuring an adequate preload status before initiating or increasing presser support is important. 19
Profound bradycardia with complete heart block and hypotension can occur because of occlusion of the blood supply to the inferior surface of the heart. This generally responds to crystalloid infusion and administration of atropine. A patient with symptoms attributed to an inferior wall MI is extremely dependent on preload, and vigorous volume resuscitation is advised. A patient may need transcutaneous or transvenous pacing, and the transport personnel should be well versed in this use.
Hypotension with distended neck veins and clear lungs occurs in patients with right ventricular infarction as a result of occlusion of the proximal right coronary artery, which supplies blood to the right ventricle. Patients with right ventricular infarctions are extremely volume dependent because of inadequate preload to the left ventricle. Because the right and left ventricles work in circuit, a poorly functional right ventricle is unable to maintain adequate volume back to the left ventricle. The patient shows signs of poor cardiac output despite adequate left ventricular function. The basis of therapy for a right ventricular infarction is large volumes of intravenous fluid. Also, medication that reduces preload, such as nitrate and morphine, should be used with extreme caution if at all.
Therapy for Acute Myocardial Infarction
As with the previously described acute coronary syndromes, an AMI begins as a platelet problem; therefore, the same treatment, anticoagulation with antiplatelet therapies, is initiated.
Rescue angioplasty is angioplasty performed for the purpose of definitive reperfusion therapy and is considered the standard of care. The use of intracoronary stenting may result in decreased levels of restenosis in the future.
Critical care transport personnel are essential in the rapid transport of these patients to tertiary institutions with angioplasty facilities. Acknowledgment of the presence of an AMI at a referral institution should prompt the receiving institution to activate its cardiac catheterization laboratory staff. This process can be facilitated by transport personnel. Direct physician-to-physician contact and the timely fax of electrocardiographic results can avoid unnecessary delays in reperfusion therapy.
In patients with adequate blood pressure (SBP > 110 mm Hg) and the absence of posterior or inferior involvement, intravenous (IV) morphine sulfate (2 to 4 mg) and IV NTG therapy (goal is to decrease SBP by approximately 10% to 20%) can be used for pain control. Beta-blockers (metoprolol 5 mg IV q 5 min for three doses) can help decrease myocardial oxygen demand in the patient with no contraindication (i.e., severe congestive heart failure, bradycardia, or diffuse wheezing).
Emergency coronary artery bypass surgery (CABG) is performed in the setting of an AMI for patients with severe left main disease, failed angioplasty, and multivessel coronary artery disease not amenable to percutaneous revascularization. The time needed to activate a pump team and to place patients on bypass limits CABG as an initial strategy for reperfusion of an infarcting segment of myocardium.
Critical care transfer of these patients via air or ground is essential and should not be performed by basic or advance life support (ALS) units.
DYSRHYTHMIAS
Serious electrical abnormalities of the heart rate and rhythm are classified as dysrhythmias. They are described on ECGs as a deviation from the normal sinus rhythm in rate, too fast as in tachycardia or too slow as in bradycardia; in regularity, one or more beats occurring earlier or later than expected; or in a different pattern of activation of the cardiac muscle. Dysrhythmias can originate in any area of the heart. They are usually divided into arrhythmias that start in the atrium, that start in the atrioventricular (AV) node, and that start in the ventricle. Identification of the origin of the dysrhythmias is based on the following:
1. The relation between the P wave and the QRS complex.
2. The width and configuration of the P wave, which indicate how the atria are activated during the arrhythmia.
3. The width and configuration of the QRS complex, which indicate how the ventricles are activated during the arrhythmia.
Dysrhythmias are caused by a variation in the state of discharge of the sinoatrial (SA) node, ectopic impulses that compete with the SA node, or abnormal conduction of impulses from the SA node through the heart. The origin of a normal heartbeat is in the sinus node. The sinus node is located laterally near the junction of the superior vena cava and the right atrium. The sinus node is actually a region made up of groups of pacemaker cells, which automatically depolarize. The blood flow to this region is variable.
Dysrhythmias can be categorized as tachyarrhythmias, which include atrial tachycardia, supraventricular arrhythmias, and ventricular arrhythmias, and bradyarrhythmias, which include symptomatic bradycardia and conduction disturbances known as atrioventricular (AV) blocks.
Pathophysiologic Factors
The cells that conduct the electrical current through the heart are known as the pacemaker or automatic cells. The pacemaker of the heart, the SA node, is located at the junction of the superior vena cava and the right atrium. An electric impulse is initiated at this node, and it travels through the internodal pathways to the AV node.
The AV node is located in the right atrium, directly above the tricuspid valve and anterior to the coronary sinus. The electrical impulse travels through the AV node and then moves through a common bundle of His, which divides almost immediately into the right and left bundles. The left bundle divides further to form two direct pathways to the anterior and posterior papillary muscle. The electrical impulse then permeates the many small fibers of the Purkinje network, beginning at the endocardium and ending in the ventricular myocardium. 19
Dysrhythmias are the result of an irritable focus or foci in the electrical conduction system. Several mechanisms contribute to the development of dysrhythmias. The ischemic process and postnecrotic entities and underlying cardiac disease may enhance myocardial electrical instability. In addition, the development and treatment of myocardial failure result in mechanical dysfunction, metabolic changes, and electrolyte shifts and contribute to rhythm disturbances. Invasive cardiac instrumentation or pharmacologic therapies also have the potential to provoke serious dysrhythmias.
Assessment, Diagnosis, and Treatment
Tachyarrhythmias/Tachycardias
Atrial Tachycardia
Atrial tachycardias possess a regular rhythm; the impulse formation is in the atrium but outside the sinus node. P waves precede the QRS but are usually obscured because of the rapid heart rate, although AV conduction may be present. The heart rates vary from 140 to 240 bpm. The incidence rate of atrial tachycardia seems to increase with age. Current classifications of atrial tachycardia are based on the three mechanisms responsible for the tachycardia. 19
a. Intraatrial reentry. The characteristics of the reentrant circuit in atrial tachycardia are still not well understood. Many of these patients have other arrhythmias, specifically atrial fibrillation, or flutter. 19 Common symptoms are dyspnea, fatigue, and palpitation. Although the condition is symptomatic, usually no associated homodynamic compromise is seen. Catheter ablation is the primary therapy for reentrant atrial tachycardia with a suggested success rate of greater than 75%.
b. Ectopic automaticity. This is generated by a single or multiple atrial focus that clusters around the crista terminalis in the right atrium and around the base of the pulmonary veins in the left atrium. This condition has also been referred to as multiple atrial tachycardia (MAT). This is a descriptive entity that is characterized by an atrial rate of greater than 100 bpm with P wave morphology with varying AV intervals. It is easily confused with atrial fibrillation, and its mechanism is unknown. However, it is seen in patients with acute pulmonary disorders and accompanying hypoxia. Treatment is focused on the underlying cause. Patients with this mechanism are generally younger in age. This tachycardia does not respond to vagal maneuvers and is not terminated with atrial pacing. Treatment of precipitating causes is advisable. Also, amiodarone has been found to be useful. 19
c. Atrial flutter. Atrial flutter presents as a series of rapid regular flutter waves, usually described as saw tooth or resembling a picket fence, with a rate from 220 to 350 bpm. Diagnosis is usually made in leads II, III, and AVF. Conduction through the AV node delays impulses and thus prevents rapid ventricular rates. The QRS complex during atrial flutter is usually the same as during sinus rhythm. Treatment methods are addressed in conjunction with fibrillation.
Atrial fibrillation. Atrial fibrillation represents chaotic atrial activity, with the atrial rate ranging from 300 to 700 bpm. Impulses are randomly conducted through the AV node to the ventricles, resulting in a typically irregular ventricular response that varies continuously in shape. Atrial fibrillation results in loss of effective atrial contraction, which reduces cardiac output and promotes mural thrombus development.
Treatment for patients with either atrial flutter or fibrillation is based on several clinical factors.
1. Is the patient’s condition clinically stable or unstable?
2. Is impaired cardiac function associated with the flutter or fibrillation?
3. Is Wolff-Parkinson-White syndrome present?
4. Has the flutter or fibrillation been present for more or less than 48 hours?40
If the patient’s condition is unstable, the patient should be appropriately treated immediately. The objectives of treatment are to control the rate, convert the rhythm, and use anticoagulation therapy when appropriate. Increased emphasis is placed on assessment of causes of the atrial fibrillation. Some underlying causes include hypoxemia, anemia, hypertension CHF, hypokalemia, and mitral regurgitation. The length of time of fibrillation or flutter is important because if such a rhythm continues for more than 48 hours, there is a risk of systemic embolization on conversion to a sinus rhythm if the patient does not undergo anticoagulation appropriately. If the condition in atrial fibrillation becomes unstable, cardioversion is recommended. If the patient is in atrial flutter, lower joules may be effective to convert the condition.
Supraventricular Arrhythmias
Supraventricular arrhythmias originate above the ventricle and reflect atrial irritability. They can originate from the SA node, the AV node, the penetrating part of the bundle of His, or an accessory pathway. 28 These arrhythmias include premature atrial contractions, atrial tachycardia, atrial flutter, and atrial fibrillation.
Premature Atrial Contractions
Premature atrial contractions are an early atrial depolarization. They are predictors of impending supraventricular arrhythmias and do not necessitate intervention.
Sinus Tachycardia
Sinus tachycardia originates in the sinus node and is characterized by a heart rate greater than 100 bpm. It is a physiologic response to a demand for a higher cardiac output, and treatment is directed toward correcting the physiologic demand, as opposed to correcting the rapid heart rate. Causes can include infection, anemia, hypotension, and hyperthyroidism. IV or oral beta-blockers may be used when the tachycardia produces symptoms, as long as the underlying cause is corrected first.
Ventricular Arrhythmias
Ventricular ectopic activity is a common phenomenon in AMI, and ventricular arrhythmias are the major cause of sudden cardiac death in the United States. The most common cause of ventricular arrhythmia is ischemic coronary artery disease. Ventricular arrhythmias arise in the ventricles beyond the bifurcation of the bundle of His.
Death from a ventricular arrhythmia occurs through its interference with the cardiac pumping function. Several conditions that occur during the ventricular arrhythmia contribute to the decrease in cardiac output, which in turn can cause syncope and lead to arrest. The loss of the normal atrioventricular sequence is associated with a significant decrease in cardiac output. The rate of the ventricular arrhythmia also determines hemodynamic instability. A rate below 150 bpm does not usually cause hemodynamic compromise if the duration is short. If the ventricular tachycardia exceeds 200 bpm, significant symptoms are usually present and can include dyspnea, light-headedness, loss of vision, syncope, and cardiac arrest. Patients with ventricular tachyarrhythmias should be closely monitored because they have an increased risk of progression to ventricular fibrillation, especially with a history of compromised ejection fraction. In the absence of an independent licensed practitioner such as a physician or nurse practitioner or orders from online medical control, adhere to the American Heart Association’s ACLS guidelines for specific algorithms for treatment of all arrhythmias or as indicated by prewritten orders. 11
< div class='tao-gold-member'>
Only gold members can continue reading. Log In or Register a > to continue

Full access? Get Clinical Tree
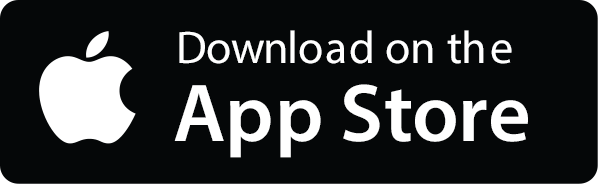
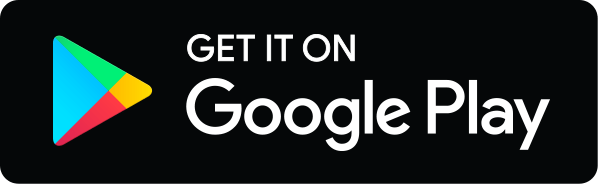
