Emad B. Mossad1, Rahul Baijal2, and Rajesh Krishnamurthy3 1 Arthur S. Keats Division of Pediatric Cardiovascular Anesthesiology, Vice Chief for the Heart Center, Department of Anesthesiology, Texas Children’s Hospital, Baylor College of Medicine, Houston, TX, USA 2 Staff Anesthesiologist, Department of Anesthesiology, Texas Children’s Hospital, Baylor College of Medicine, Houston, TX, USA 3 William E. Shiels Chair of Radiology, Clinical Professor of Radiology, Nationwide Children’s Hospital, Ohio State University, Columbus, OH, USA Congenital heart defects (CHD) are the most common of all congenital anomalies. Every day in the United States, 11,500 babies are born; of whom 450 have a congenital anomaly, of which 87 (20%) are congenital heart defects [1]. The incidence of CHD has remained consistent over the years and is similar across various geographic, racial, and ethnic groups. Although there is a suggested increase in the prevalence of specific lesions in certain regions, the incidence is mostly 7–10/1,000 live births [2, 3]. The demographics of patients with CHD are changing as screening methods evolve, diagnostic tools improve, and interventions change. Although CHD is frequently considered a disease of childhood, the population of adults with CHD is growing at the rate of 5% every year, and by the end of this decade, there will be more adults than children with CHD. The congenital cardiac anesthesiologist thus must be prepared to evaluate and care for adults as well as children with heart defects. However, there remains a significant population of neonates and infants with critical CHD who require care in their first days or months of life. In an epidemiologic study, 15% of all infants with CHD had significant physiological compromise (metabolic acidosis, seizures, cardiac arrest, or evidence of renal or hepatic injury) that required an invasive intervention in the first few weeks of life [4]. Many lesions are diagnosed prenatally, and fetal interventions to improve the outcome and progress of patients are increasingly applied [5]. The interventions are also changing rapidly, and an anesthesiologist must include in the preoperative evaluation an understanding of the planned procedure, the location, and the coordination needed between various teams. Many centers have adopted a hybrid approach to many defects, especially hypoplastic left heart syndrome [HLHS]) and multiple ventricular septal defects (VSDs). These procedures will require an up‐to‐date understanding and preparation for those ever‐changing interventions [6, 7]. The primary step in the preoperative anesthetic evaluation of the patient with CHD is an understanding of the terminology and nomenclature used for these defects. The communication between various chambers and vessels is described in terms of concordance and/or discordance. Sidedness and morphology of chambers, valves, and vessels also follow a standard description approach. To understand the nomenclature of a specific lesion, the anesthesiologist carefully considers: Following an interpretation of the nomenclature and terminology, the anesthesiologist has to understand the physiologic implications of various lesions. The most practical approach is to follow a physiologic classification for all CHDs (Table 18.1). Many CHDs that present in the neonatal period requiring urgent care are ductal‐dependent lesions where a patent ductus arteriosus is essential to maintain either systemic or pulmonary flow. Defects presenting later in life or requiring later care are classified according to the presence and direction of shunting, pulmonary blood flow, and obstruction. Although there are numerous CHDs, the majority will be classified into one of four categories, with the incidence of the most commonly seen defects representing one of each of those categories [8, 9]. Chapter 4 has a detailed presentation of the terminology and nomenclature of different CHDs. The preoperative period is the appropriate time to consider all the variables that can impact the preparation, planning, and conduct of the perioperative care for the child with CHD. The majority of congenital cardiac centers have a set weekly conference to discuss patients scheduled for surgical as well as catheter interventions. In that multidisciplinary conference, the history and condition of the patients are discussed, imaging studies are reviewed, and plans for any further consults or tests are investigated. The goal of the conference and the preoperative evaluation is to bring all the necessary expertise to understand the lesion, optimize the condition of the patient, and plan the best approach or intervention to the patient. The preoperative visit is an essential phase in preparing children with CHD and their families for the upcoming intervention. Parental knowledge, whether provided verbally or in written or visual form has been shown to improve the parents’ involvement and effectively reduce their anxiety [10]. The purpose of the preoperative visit and the consent process is not the delivery of information and significant potential risks, but to provide the necessary knowledge to the parents (and potentially the child) of the specifics of the anesthetic procedures, the common risks and the plans to prevent or mitigate them, and the role of the various caregivers. The consent process should bring the physicians and the family together as partners with the same purpose: the best care possible and the least risk acceptable for the care of the child. Well‐informed parents are able to participate in educating their children and preparing them for the intervention through therapeutic play interventions, establishing a rapport and connection with the anesthesiologist. Children whose parents are better educated and informed, and who are involved in the preparation process are less anxious and more prepared for the interventions [11]. Table 18.1 Classification of congenital heart defects DDL, ductal‐dependent lesion; PBF, pulmonary blood flow; L, R, left and right; TOF, tetralogy of Fallot; PS, pulmonary stenosis; SBF, systemic blood flow; HLHS, hypoplastic left heart syndrome; IAA, interrupted aortic arch; AS, aortic stenosis; VSD, ventricular septal defect; ASD, atrial septal defect; PDA, patent ductus arteriosus; P/CAVC, partial or complete atrioventricular canal defect; PA‐VSD‐MAPCA, pulmonary atresia with a ventricular septal defect and multiple aortopulmonary collaterals; MS, mitral stenosis; d‐TGA, dextro‐transposition of the great arteries; TAPVR, total anomalous pulmonary venous return; MR, mitral regurgitation; TR, tricuspid regurgitation; AI, aortic insufficiency; PI, pulmonary insufficiency; ALCAPA, Anomalous origin of the left coronary artery from the pulmonary artery; IDCM, idiopathic dilated cardiomyopathy; HCM, hypertrophic cardiomyopathy. An initial pre‐anesthetic evaluation includes reviewing accessible medical records, ordering appropriate preoperative tests, requesting appropriate consultations, and performing a thorough history and physical examination. Although most children have undergone a thorough evaluation by their pediatric cardiologist and cardiac surgeon, the history should not only address cardiopulmonary reserve but also other multisystem co‐morbidities that may affect anesthetic management. A review of the child’s medical record should assess the child’s underlying congenital heart pathology and whether that pathology is repaired or palliated; previous surgery, anesthetic experiences and complications, and diagnostic and therapeutic interventions; current medications and medication history; and drug or food allergies. A child’s functional status will reveal information about their cardiopulmonary reserve. Functional status is assessed differently at different ages. Feeding difficulties with associated tachypnea, dyspnea, and diaphoresis with subsequent growth abnormalities and frequent pulmonary infections indicate limited cardiopulmonary reserve in the neonate and infant. School‐aged children may exhibit decreased activity level or exercise intolerance with an inability to keep up with their peers, whereas older children may not only display fatigue, dyspnea, and orthopnea but may also verbalize chest pain, palpitations, and pre‐syncope or syncope. Furthermore, recent changes in baseline activity indicate a further decline in cardiopulmonary reserve. Recent upper (URI, upper respiratory infections) and lower respiratory tract infections (RTI) should be noted, as both may affect airway reactivity for 2–8 weeks [12]. RTI may additionally affect pulmonary vascular resistance (PVR), which may be poorly tolerated in those children with bidirectional cavopulmonary anastomosis (Glenn procedure), total cavopulmonary anastomosis (Fontan), underlying pathology, shunt physiology, or pulmonary vascular disease. The increased risk of breath‐holding, airway obstruction, and laryngospasm with subsequent changes in ventilation and oxygenation poses an increased risk to children with limited cardiopulmonary reserve. The recent COVID‐19 pandemic has heightened our awareness of the potential risk of proceeding with elective procedures in the face of a URI, even in asymptomatic patients, and the need to protect healthcare providers as well as other patients when planning unavoidable procedures on such patients [13]. Even in the asymptomatic SARS‐COVID‐19 polymerase‐chain‐reaction (PCR) positive patient, the incidence and degree of respiratory complications are higher than in children with other UTIs. Careful screening, identification of at risk‐patient, selective scheduling, and safe practice with protective personal equipment for the care providers are essential lessons learned from this pandemic that will be applicable and useful for any future similar viral pandemics [14]. Laboratory tests are available to potentially confirm the diagnosis of an RTI. Nasopharyngeal swabs or aspirates may detect common viral infections, but, depending on the phase of the infection, they may offer limited sensitivity. White blood cell counts are a poor indicator of infection, especially in neonates and children with immature or impaired immune systems. Chest radiograph findings typically lag behind the presentation of clinical symptoms in children with lower RTIs and are often of little utility. Children with URIs undergoing cardiac surgery have an increased incidence of postoperative complications when compared with children without a URI. The recommended period for recovery from a URI and avoiding perioperative respiratory adverse events is a 4‐ to 6‐week period for the recovery of mucosal and ciliary function in children scheduled for cardiac surgery or catheterization, especially if airway instrumentation is planned [15, 16]. Children with underlying congestive heart failure (CHF) who present with a URI pose a dilemma for the perioperative team. It may be challenging to distinguish baseline CHF symptoms as noted earlier from URI symptoms. Furthermore, although decreased respiratory reserve in children with a respiratory tract infection may place the child at risk for perioperative complications, the need for surgical expediency given the CHF may obviate the URI symptoms, and it may be prudent to proceed with surgery to prevent further progression of the CHF. The physical examination further assesses the child’s general condition. Observation of the child during the pre‐anesthetic evaluation may be the most valuable component of the physical examination. Is the child active, playful, age‐appropriate, lethargic, or in failure to thrive compared with similarly aged children? Vital signs, including weight and height, heart rate, respiratory rate, blood pressure, and oxygen saturation (SpO2) should be recorded. Failure to thrive with reduced weight and height indicates limited cardiopulmonary reserve and functional status. Blood pressure variation between extremities suggests an unexpected coarctation of the aorta. Tachypnea is one of the earliest signs of CHF. Cyanosis is usually recognized when the SpO2 is 85% or lower and is associated with unrepaired or palliated CHD from decreased pulmonary blood flow and right‐to‐left shunting, but children with left‐to‐right shunting may also have desaturation from pulmonary edema [17]. Progressive cyanosis or frequent cyanotic episodes suggest the potential for rapid cardiopulmonary decompensation intraoperatively. Pre‐ductal and post‐ductal oxygen saturations should be recorded in an infant with an unrepaired defect. If the pre‐ductal (right arm) saturation is higher than the post‐ductal saturation, deoxygenated blood from the pulmonary circulation enters the descending aorta through a patent ductus arteriosus (PDA). This differential cyanosis may occur with persistent pulmonary hypertension of the newborn and left‐heart abnormalities, such as aortic arch hypoplasia, interrupted aortic arch, critical coarctation of the aorta, and critical aortic stenosis. The remainder of the physical examination should be a detailed evaluation of the airway, chest, and heart, with any changes from baseline noted. Wheezing and rales on lung auscultation indicate not only reactive airway disease, chronic lung disease, or an acute pulmonary infection but also CHF. Auscultation of the heart should ideally be performed in a quiet setting with the child being calm and cooperative. Heart sounds, pathologic murmurs, particularly a different murmur from previously documented, gallop rhythms, and the presence of a thrill representing a palpable murmur should be noted. Hepatomegaly, jugular venous distension, and peripheral edema indicate CHF, from either left‐ or right‐sided pathology. Jugular venous distension is of limited value in neonates and infants but may provide qualitative information about central venous pressure in older children [18]. Heart sounds and murmurs are influenced by patient position during auscultation as well as the period of the respiratory cycle during which the heart sound or murmur is being evaluated. Repositioning the child from supine to sitting decreases venous return suddenly and improves the auscultation of heart sounds and murmurs. Innocent murmurs increase in intensity in the supine position. Diminished peripheral pulses and delayed capillary refill indicate reduced systemic perfusion. The peripheral pulse will be reduced in the arm in which the subclavian artery to pulmonary artery shunt (Blalock–Taussig shunt) was constructed; the blood pressure will also be reduced in that ipsilateral arm. Finally, clubbing may indicate long‐standing cyanosis. New or different physical examination findings from baseline necessitate further evaluation, which may include a chest radiograph, electrocardiogram (ECG), or echocardiogram. Current and potential vascular access sites should be assessed, as it is frequently difficult to obtain access in children with CHD. Parents often have insight and knowledge about previous vascular access that can facilitate the selection of sites for potential vascular access. Children with CHD often have multiple previous arterial and venous cannulations that may result in trauma or thrombosis to those vessels. That information is additionally valuable when considering what potential sites to access. Arterial access should be avoided on the side where a Blalock–Taussig shunt was constructed as peripheral pulses and blood pressure will be reduced in that ipsilateral arm. The airway evaluation is an essential component of the preoperative evaluation of children with CHD, particularly those with chromosomal abnormalities and syndromes. Airway evaluation should include a history of noisy breathing that may indicate airway compression from supraglottic or subglottic obstruction; for example, children with aortic arch obstruction have a 27% incidence of subglottic airway compression that becomes more symptomatic postoperatively following an end‐to‐side aortic arch reconstruction [19]. Previous airway history also includes previous airway interventions and complications and previous surgery to the face and neck. Airway physical examination includes assessing body mass index, patency of nares, degree of mouth opening, tongue size, palate shape and size, mandibular size, and neck mobility. Mandibular size with micrognathia and retrognathia is associated with difficult mask ventilation and intubation [20]. Many chromosomal abnormalities and syndromes that are associated with CHD, including trisomy 13, 18, and 21, DiGeorge syndrome and Turner syndrome, have an abnormal airway examination that may indicate possible difficult mask ventilation or intubation. Patients with conotruncal abnormalities, including pulmonary atresia, truncus arteriosus, and interrupted aortic arch are at increased risk for DiGeorge syndrome or velocardiofacial syndrome (partial deletion of chromosome 22q11.2). All neonates and infants with reduced functional residual capacity and increased oxygen consumption require a patent airway to prevent cardiorespiratory decompensation, but children with cyanotic CHD or pulmonary vascular disease are particularly at risk of inadequate ventilation and oxygenation from a compromised airway. Previous anesthetic records should always be reviewed for any potential difficulty in mask ventilation and/or intubation along with any airway adjuncts used during mask ventilation, intubation, or extubation. Children with trisomy 21 frequently present for surgical and other interventions at various stages of life. As these children’s age progresses, the risk of hypotonia, atlanto‐axial instability, and spinal cord compression increases [21]. Many centers have adopted an algorithm to approach these patients and decide on the imaging needed and the best approach to the airway [22]. The algorithm applies standard cervical spine series with flexion and extension views in older trisomy 21 patients, or patients with any signs or symptoms of atlanto‐axial instability. An atlanto‐dens interval >4.5 mm or a neural canal width <14 mm provides indirect evidence of cervical spine instability and will require confirmation by magnetic resonance imaging (MRI). If confirmed, these findings in the preoperative period are significant for a high risk of atlanto‐axial instability and the need for a neurosurgical consult, potential surgical fusion, or, at a minimum, a modification of airway management and intraoperative positioning. The finding of poor laryngoscopic view, reported as a Cormack and Lehane [23] grade III or IV, is significantly higher in children with CHD, with a reported incidence of 3.5%, twice as high as the general pediatric population. This incidence was higher in patients below 1 year of age (5.6%) and in those with concomitant chromosomal and genetic abnormalities [24]. Approximately 25–40% of children with CHD may have associated anomalies, which may include neurologic, airway, gastrointestinal, hematologic, endocrine, syndrome, or chromosomal abnormalities [25]. Approximately 5–10% of children with CHD have a known chromosomal abnormality – the most common abnormalities associated with congenital heart disease are listed in Table 18.2. (see Chapter 8 for further discussion of genetic syndromes and CHD). Similar to adults, children can have significant cardiac decompensation, resulting in poor peripheral perfusion and the inability to adequately support vital organ function. Unlike adults, where CHF is most commonly caused by myocardial ischemia and dysfunction, children may have CHF despite normal myocardial vascularity and function. This may occur in the presence of significant volume overload (in shunt lesion) or pressure overload (in obstructive lesions), and frequently in patients with single‐ventricular physiology, especially those with a systemic right ventricular morphology. CHF is characterized by reduced cardiac output and increased venous pressure in the systemic and/or pulmonary venous systems, often secondary to an abnormality in the myocardium. Cardiomyopathy is an abnormality of the myocardium. Cardiomyopathies are classified as dilated, restrictive, hypertrophic, arrhythmogenic, or unclassified [26]. Other causes of CHF in children include volume overload secondary to large left‐to‐right shunts, valvar regurgitation, and obstructive pathology. The classification of heart failure focuses primarily on the severity of illness. The New York Heart Association classification, which ranges from I (asymptomatic) to IV (heart failure with symptoms at rest), was designed for adults but may also be used for older children. The Ross Heart Classification Scale also ranges from I to IV but may be used in infants (see Table 18.3). Accurate assessment and grading of CHF in children require consideration of age, activity, and compensatory mechanisms. Thus the revised Ross Classification has five different age groups with the scaling system (0–2) evaluating different activities and findings per age group [27]. The Canadian Cardiovascular Society has established recent guidelines for the age‐appropriate diagnosis of CHF, using commonly encountered symptoms, with recommendations for further investigations (brain natriuretic peptide [BNP], endomyocardial biopsy) and a stepwise introduction for medical therapy to optimize children with CHF prior to surgery and intervention [28]. Table 18.2 Chromosomal and genetic defects, syndromes and congenital heart disease CAVC, complete atrioventricular canal defect; ASD, atrial septal defect; VSD, ventricular septal defect; PDA, patent ductus arteriosus; BAV, bicuspid aortic valve; TOF, tetralogy of Fallot; DORV, double outlet right ventricle; AS, aortic stenosis; IAA, interrupted aortic arch; PS, pulmonary stenosis; HCM, hypertrophic cardiomyopathy, AV, aortic valve; MV, mitral valve; MVP, mitral valve prolapse. The prevalence of heart failure‐related hospitalization for children <18 years has not changed with time, amounting to 11,000–14,000 admissions annually. In a retrospective analysis of the largest pediatric admissions database, the Healthcare Cost and Utilization Project Kids’ Inpatient Database, between 1997 and 2006, pediatric heart failure‐related admissions were at the rate of 14.5–17.9/100,000 children. Although the prevalence has not changed significantly, the overall mortality is 7%, significantly higher than the overall expected mortality of 0.4% for all pediatric admissions with no, or compensated, heart disease. The hospital length of stay is significantly longer, and the outcome is clearly influenced by co‐morbid conditions [29]. Table 18.3 Ross classification of congestive heart failure in children (Source: Ross [27]. Reproduced with the permission of Springer Nature.) Children with limited cardiopulmonary reserve should be identified preoperatively as those children at the highest risk of perioperative morbidity and mortality. Preoperative evaluation should include the degree and progression of symptoms and heart failure therapy. The anesthesiologist should communicate directly with the treating cardiologist to assess the child’s functional and clinical status. Additionally, strategies and decisions for escalation of care should be discussed with the child’s parents, cardiologists, anesthesiologists, and/or intensivists. This might include the potential need for extracorporeal membrane oxygenation or other circulatory support devices. Infants with CHF often have difficulty feeding, diaphoresis with feeding, inadequate growth, and frequent pulmonary infections. Older children with CHF have symptoms similar to adults, including dyspnea at rest or exertion, orthopnea, angina, and peripheral edema. The clinical findings are similar to those seen in adults, with some variations specific to children. Common findings include tachycardia, shortness of breath, difficulty feeding, and lethargy. Hepatomegaly is frequently a characteristic sign of right‐sided CHF, with edema seen in the sacral area (especially in babies) as well as the lower extremities. Left‐sided CHF is manifested with pulmonary congestion, gallop on auscultation, and poor peripheral perfusion with cold and clammy skin. Children with CHF have low baseline blood pressure from the combination of diuretic therapy, angiotensin‐converting enzyme (ACE) inhibitors, and beta‐blocker therapy. Preoperative volume status should be assessed, as these children often have intravascular hypovolemia that is exacerbated by preoperative fasting. Children with CHF who are in‐patients often have disease progression with increased perioperative risk. Those children who are treated with inotropes, vasodilators, and inodilators have the potential to deteriorate significantly with anesthetic exposure, and the ability to escalate support to maintain cardiac output is limited. B‐type natriuretic peptide (BNP) is a hormone that is synthesized and released into the circulation by the ventricular myocytes in response to volume expansion and increases in myocardial wall stress. The marker is useful for assessing the functional status and cardiac function in those children with CHF. BNP is age‐ and gender‐dependent, so higher BNP values are found in older female patients [30, 31]. Hypoxemia directly increases BNP production and is elevated in uncorrected or palliated single‐ventricle patients. Children with CHD and pulmonary hypertension (PAH) are at significant risk for perioperative cardiac complications, whether in the cardiac operating room, the catheterization laboratory or for non‐cardiac procedures or imaging studies [32]. The preoperative assessment can be done using the clinical presentation, imaging studies, and, most importantly, cardiac catheterization information that reports the pulmonary/systemic pressure ratio and the reactivity of the pulmonary vascular bed [33]. Important information preoperatively is the response of the pulmonary vascular bed to oxygen, hyperventilation and inhaled nitric oxide and intravenous (IV) dilators. It is essential to consider the medical regimen for managing the patient, the potential benefit of additional therapy, and the safest interventions [34]. The preoperative use of single or combination therapy, including prostacyclin analogs (Treprostinil/Remodulin), endothelin receptor antagonists (Bosentan), and phosphodiesterase inhibitors (Sildenafil) even for a short period can have significant impact on intraoperative risk and postoperative outcomes, especially in patients with severe PAH [35, 36]. Children on multiple therapies may be unsafe to proceed with an intervention without a period of optimization, or a procedure may preferably be done at the bedside, avoiding the triggering effects of motion, temperature change, and stimulation on pulmonary vascular resistance (PVR). Chapter 33 has a comprehensive review of the assessment, therapy, and potential outcomes for children with CHD and PAH. Neonates and premature infants continue to present the most critically ill group of patients with CHD requiring preoperative evaluation and urgent interventions. Early recognition and appropriate preparation result in a significant improvement in outcome. The American Heart Association (AHA) thus recommends routine oximetry screening on all neonates prior to discharge to prevent delayed diagnosis of critical CHD. The estimated sensitivity for detection is 70% and the risk of false‐positive screening oximetry that results in further evaluation is only 0.035% of infants screened within 24 hours of birth [37]. There is a significant negative linear relationship between perioperative mortality of preterm and near‐term infants with CHD and gestational age, decreasing significantly for the same lesion as gestational age approaches 40 weeks [38]. It is also evident that the neurodevelopmental outcome, studied as various domains of language, cognition, and visual or fine motor skills up to 4 years of age, is improved in infants and children with various CHDs when delivery (spontaneous or elective/induced) is at 39–40 weeks’ gestation [39]. The preoperative preparation and multidisciplinary discussion should examine the gestational age of the fetus with CHD and the best timing and location of delivery for a neonate with critical CHD [40]. Although small for gestational age and low birth weight are strong independent predictors of mortality in the first year of life following repair of CHD [41], delay of interventions neither compromises nor improves the outcome. For neonates <2 kg, survival following surgical or interventional repair of CHD was determined by prenatal diagnosis (improved), type of cardiac lesion (worse), with defects including pulmonary venous malformations, and associated extracardiac defects [42]. Children with CHD are often taking numerous medications, including diuretics, angiotensin‐converting enzyme (ACE) inhibitors, angiotensin receptor blockers (ARBs) beta‐blockers, anti‐arrhythmics, and digoxin. These medications should be continued preoperatively until the time of the surgical procedure. Reports in the adult literature suggest that ACE inhibitors and ARBs may cause prolonged hypotension during anesthetic induction and should be discontinued at least 24 hours prior to the surgical procedure [43]. Digoxin is discontinued 24 hours prior to the surgical procedure, as serum levels may be potentially toxic postoperatively [44, 45]. The American College of Chest Physicians recently published guidelines for antithrombotic therapy in children [46]. Children with systemic‐to‐pulmonary artery shunts, a history of shunt or conduit thrombosis, mechanical or biological prosthetic heart valves, transcatheter interventions or device placement, treatment of Kawasaki disease, and risk factors for the thromboembolic phenomenon, such as bicaval pulmonary anastomosis, are often taking antithrombotic therapy. No specific guidelines exist for discontinuation of antithrombotic medications prior to elective surgery in children. Aspirin irreversibly inhibits cyclooxygenase for the life of the platelet, approximately 10–14 days [47]. Clopidogrel also irreversibly inhibits platelet aggregation. A child taking preoperative aspirin and/or clopidogrel to maintain shunt patency should continue these medications, as the risk of shunt thrombosis is greater than that of perioperative bleeding. Immediately discontinuing aspirin preoperatively does not reduce the risk of perioperative bleeding, given aspirin’s long half‐life. There should be a discussion with the child’s cardiologist, surgeon, and anesthesiologist regarding the optimal timing for stopping these medications. Children who are taking warfarin for their antithrombotic medication should discontinue the warfarin 3–5 days prior to the surgical procedures. The international normalized ratio will usually fall below 2.0 in approximately 2–3 days and will normalize in 4–6 days [48]. Patients should be admitted 2–3 days prior to the surgical procedure to start a bridging regimen of IV heparin. Cessation of the IV heparin 3–6 hours prior to the surgical procedure does not increase the risk of perioperative bleeding [49]. Prostaglandin E1 (Alprostadil) maintains ductal patency in ductal‐dependent lesions, such as pulmonary atresia with or without VSD, tricuspid atresia, HLHS, interrupted aortic arch, or severe coarctation of the aorta. The dose, usually between 0.0125 and 0.1 μg/kg, is adjusted to maintain appropriate oxygenation without signs of over‐circulation, decreased systemic diastolic blood pressure, and appropriate acid‐base status. Common side effects of prostaglandin E1 infusion include apnea, fever, flushing, tachycardia or bradycardia, and platelet dysfunction; rarely, cardiac arrest can occur, with the prostaglandin being a contributing factor. Children who have undergone heart or lung transplantation are often taking multiple immunosuppressive medications that should be continued through the perioperative period to reduce the risk of acute rejection. Finally, children who are taking other medications, such as those for reactive airway disease, gastroesophageal reflux, and seizure disorder, should continue these medications until the surgical procedure (Box 18.1). Children with CHD frequently have rhythm disturbances that require anti‐arrhythmic therapy and are manifest on a preoperative ECG. A 12‐lead ECG with adequate length of lead II recording for diagnosis of arrhythmias and assessment of changes from baseline is an important component in the evaluation for cardiac surgery. Even if it is “normal” (i.e., normal sinus rhythm without any evidence of chamber enlargement or repolarization abnormalities, etc.), the formal 12‐lead ECG serves as a comparison to the postoperative state if ECG changes are suspected and thus should be obtained before any cardiac surgery. If the patient has a history of significant arrhythmia or suspicion for changing rhythm status, a 24‐hour Holter monitor is an extremely valuable test to assist in planning for the management of cardiac rhythm during the procedure. Chapter 22 presents an extensive discussion on ECG and arrhythmia analysis and treatment. Pacemakers and implantable cardioverter defibrillators, collectively referred to as cardiac rhythm management devices (CRMDs), are commonly used in children with CHD due to either congenital, acquired, or iatrogenic rhythm disturbances. These devices may be placed temporarily (postoperatively) or as permanent due to intrinsic heart disease (common with CCTGA and cardiomyopathies) or they may be acquired following cardiac operations due to scarring, patches, and morphologic chamber changes (as with repaired tetralogy of Fallot). The preoperative period is a critical time to prepare the child with a CRMD for a safe perioperative period. The primary step is to obtain a detailed history of the indication, timing, and type of device placed. Devices have a standard nomenclature established by the North American Society of Pacing and Electrophysiology (NAPSE). An understanding of the position/nomenclature used is essential: I, chamber (s) paced; II, chamber(s) sensed; III, response to sensing; IV, rate modulation; V, multi‐site pacing capability. It is important to obtain an ECG to identify pacer spikes and rate, and a chest radiograph to examine the location of the generator and the pacing leads/wires. The CRMD should be interrogated by a programmer, a manufacturing consultant, or an electrophysiologist and its battery life examined [50]. Children are more vulnerable than adults to faster battery generator utilization (due to heart rate) or dislodged/fractured leads (growth changes) or to receiving inappropriate shocks, as shown in a large pediatric CRMD series [51]. The CRMD should be reprogrammed for elective surgery to an asynchronous mode at a rate appropriate for the age and metabolic demands of the patient and the planned procedure, with all anti‐tachycardia function turned off. The location of the generator must be clearly marked and noted to plan the necessary approach to avoid or limit electromagnetic interference intraoperatively [52]. For urgent or emergency procedures, a magnet may be placed on a CRMD to revert the device to a fixed rate, asynchronous pacing mode where no cardiac activity is sensed. Caution must be used when applying a magnet, as the preset rate may not be sufficient for the metabolic demands and may be dependent on the battery voltage and life of the generator. A regular 90 Gauss magnet will deactivate the anti‐tachycardia function of an implantable cardioverter‐defibrillator, but not reset an asynchronous pacing mode. Thus, for all children with a CRMD, whether the device is reprogrammed or a magnet is used, perfusion must be monitored with a waveform monitoring device (pulse oximeter or arterial line wave) throughout the operative procedure until the magnet is removed or the device is interrogated and reprogrammed [53]. Chapter 22 contains a detailed discussion of the management of CRMDs.
CHAPTER 18
Preoperative Evaluation and Preparation
Introduction
The patient with congenital heart disease
Terminology and classification
Multidisciplinary approach
Consent
Category
Shunt direction
PBF
Most common examples
Ductal‐dependent lesions (neonatal period)
DDL for PBF
L‐to‐R
Decreased
Pulmonary atresia, tricuspid atresia, TOF with severe PS, Critical PS
DDL for SBF
R‐to‐L
Increased
HLHS, Preductal coarctation, IAA, Critical AS
Common CHD
Acyanotic shunt lesions
L‐to‐R
Increased
VSD (20%), ASD (16%), PDA (12%), P/CAVC (4%)
Cyanotic shunt lesions
R‐to‐L
Decreased
TOF (7%), Ebstein anomaly, PA‐VSD‐MAPCA
Obstructive lesions
No shunting
Normal
Coarctation (6.8%), AS, PS, cor triatriatum, MS
Mixing lesions
L ↔ R
Variable
d‐TGA (3.6%), Truncus Arteriosus, TAPVR
Regurgitant lesions
No shunting
Normal
MR, TR, AI, PI (most post‐surgical repairs)
Others
Variable shunting
Variable
Vascular rings, anomalous coronary lesions (ALCAPA), tumors, cardiomyopathy (IDCM, HCM)
Preoperative evaluation
History and physical examination
Congestive heart failure
Chromosome, genetic, or phenotypic abnormality
Incidence
Associated CHD
Extracardiac anomalies
Common chromosomal defects
Trisomy 21 (Down syndrome)
1 : 650
CAVC, primum ASD, VSD
Mid‐facial and mandibular hypoplasia, micrognathia, macroglossia, epicanthal folds, simian creases, pronounced hypotonia, atlanto‐axial subluxation
Trisomy 13 (Patau syndrome)
1 : 7,500
VSD, PDA, BAV
Mid‐facial hypoplasia, cleft lip, microphthalmia, microcephaly, developmental delay
Trisomy 18 (Edward syndrome)
1 : 7,000
Malalignment VSD, TOF, DORV
Prominent occiput, short neck, low set ears, small mandible, clenched fists
Monosomy X (XO‐Turner syndrome)
1 : 2,500
BAV, AS, coarctation
Triangular facies with slanting palpebral fissure, short stature, webbed neck, difficult airway, lymphedema
22q11 deletion (DiGeorge–velocardiofacial syndrome)
1 : 4,000
Conotruncal anomalies, IAA, truncus arteriosus
Thymic hypoplasia, hypoparathyroidism, immunodeficiency, hypocalcemia, renal anomalies, learning disability
7q11 deletion spectrum (Williams syndrome
1 : 20,000
Valve and supravalvular AS, coronary ostial anomalies, peripheral PS
Elfin facies, friendly “cocktail” personality, developmental delay, short stature, hypercalcemia
Common genetic defects
Noonan syndrome (12q22 single gene defect)
1 : 2,000
HCM, PS, ASD, TOF, coarctation
Short webbed neck, micrognathia, dental malocclusion, cognitive delay, vertebral anomalies, pectus excavatum
Ehler–Danlos syndrome (variable gene mutation COL5A1)
1 : 5,000
AV/MV prolapse, aortic dissection
Temporomandibular joint dislocation, hyperextensibility of skin and joints, poor wound healing
Marfan syndrome (FBN1 single fibrillin gene defect)
1 : 5,000
Aortic root dilation/aneurysm, MVP
Airway abnormalities (high arched palate), pectus excavatum, scoliosis, joint dislocations
Duchenne muscular dystrophy (absent dystrophin gene Xp21)
1 : 3,500
Cardiomyopathy, conduction abnormality
Progressive respiratory failure, severe scoliosis, muscle wasting
Syndromic Associations
VACTERL
Vertebral, Anal, Cardiac (VSD, TOF), Tracheoesophageal, Renal, and Limb defects
CHARGE
Coloboma, Heart defect (TOF, Truncus), Choanal atresia, Retardation, Genitourinary, Ear abnormalities
Stage
Presentation
A
Intact function but recognized to have a risk factor for the development of heart failure, e.g., exposure to cardiotoxic chemotherapy or a valvular abnormality that imposes a volume or pressure overload on the ventricle
B
Presymptomatic state where there is discernible myocardial dysfunction, including chamber enlargement or reduced systolic function. Never had signs or symptoms of heart failure
C
Present or past state of symptomatic heart failure. Includes patients with New York Heart Association (NYHA) class II and III disease as well as patients who have had signs or symptoms but are asymptomatic by virtue of heart failure treatment
D
Advanced heart failure requires specialized therapy such as intravenous inotropes or mechanical circulatory support
Pulmonary arterial hypertension
The neonates and premature infants
Medications
Electrocardiographic evaluation, pacemakers, and defibrillators

Full access? Get Clinical Tree
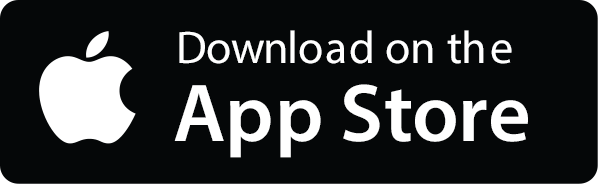
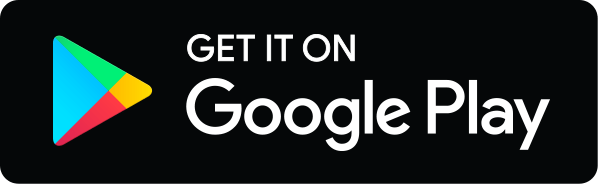