Introduction
Every year six million children, including 1.5 million infants, receive general anesthesia for a surgical procedure within the United States [1]. In particular, premature infants are often in need of multiple surgical procedures, thereby requiring multiple general anesthetics.
Despite this being an essential practice, most anesthetics routinely administered to neonates and infants have not been adequately tested for safety or efficacy. A growing body of evidence indicates that exposure to anesthetic drugs may be harmful to the developing brain of very young children [2–4]. Controversy exists regarding to what extent long-term neurodevelopmental outcome is impacted by the repeated exposure to general anesthesia. Yet it seems to be clear that the effects of anesthesia are most detrimental in the age group of neonates and infants, as well as very old patients.
Against this background it appears prudent to push for the development of new anesthetic drugs with a better neuroprotective pharmacologic profile.
Xenon
The noble gas xenon has been around in anesthesia practice for more than 60 years. The name xenon derives from the Greek term for stranger, reflecting its rarity. As a colorless, heavy, odorless gas, xenon constitutes 0.0018 percent of the earth’s atmosphere.
Xenon was discovered in 1898 by Sir William Ramsay and Morris Travers, who purified the inert gas by fractional distillation from air. Yet, it was not until 1951 when it was used for the first time as an anesthetic agent by Cullen and Cross [5]. Since then, xenon has been considered as the closest candidate for an ideal anesthetic gas. High costs in the purification process that involves liquefaction of air and subsequent fractional distillation make xenon an expensive anesthetic.
Since 1990 xenon has regained attention as more studies were published examining clinical aspects of xenon anesthesia. Xenon has favorable kinetic characteristics: it is inflammable, not explosive, and does not undergo metabolism. Furthermore, it does not contribute to atmospheric pollution. Xenon does not affect hepatic and renal function and is not teratogenic or fetotoxic [6–8].
Mechanism of Action
The N-methyl-D aspartate (NMDA) receptor antagonist xenon blocks the NMDA receptor competitively at its glycine co-agonist binding site. This inhibition is considered to be the main mechanism for xenon’s anesthetic and neuroprotective properties [9].The NMDA receptor is an ionotropic receptor that is activated by the binding of glutamate. Glutamate is the main excitatory neurotransmitter, yet constant activation of the NMDA receptor has been found to contribute to neuronal death in the setting of ischemia or stroke [5].
In addition, other ionotropic transmembrane receptors such as the α-amino-3-hydroxy-5-methyl-4-isoxazolepropionic acid receptor (AMPA) might contribute to xenon’s anesthetic and analgesic action as well, yet their precise role is not fully understood [10].
Pharmacokinetics
The blood partition coefficient of xenon is 0.115 and therefore significantly lower than any of the other to-date known inhalational anesthetics [11]. The minimum alveolar concentration (MAC) of xenon has been estimated as 63–71 percent [12].
Very short induction and emergence times have been demonstrated in various studies [13,14]. This might be advantageous in an outpatient setting as well as any other clinical scenario where fast-tracking is desirable.
One of the many reasons why xenon regained attention is its cardio protective profile: Systolic blood pressure is maintained at baseline values during xenon anesthesia [15]. Ventricular function as assessed by transesophageal echocardiography is unchanged [16]. In a guinea pig ventricular muscle model, no effect on cardiac contractility could be observed under xenon exposure. In contrast, isoflurane led to a 30 percent decrease in myocardial contractility in the same study [17].
Great hemodynamic stability was also observed in adults with markedly reduced ejection fraction who underwent cardioverter/defibrillator implantation [18]. Pilot studies examining the safety feasibility of xenon anesthesia in children undergoing cardiac catheterization are currently on the way [19].
Neuroprotection, Therapeutic Hypothermia
To date, several studies demonstrate xenon’s role acting as a neuroprotective agent by inhibiting apoptotic neuronal signaling pathways. An activation of NMDA receptors may lead to initiation and progression of apoptosis in the context of cerebral hypoxia and ischemia.
Xenon reduces NMDA receptor signaling through its antagonistic property and also limits ischemia-induced neurotransmitter release [5].
Subanesthetic doses of xenon were shown to be beneficial in a neonatal hypoxia-ischemia model in rats, where three hours of xenon exposure following hypoxia-ischemia provided short-term neuroprotection [20]. Other studies investigated xenon as a preconditioning agent: preexposure to xenon for two hours revealed a decrease in infarction size and improved neurological function in neonatal hypoxic-ischemic rats [21].
Recently a clinical feasibility study was conducted in England in which 14 infants were subjected to hypothermia and ventilated with 50 percent xenon for a time period of up to 18 hours for the treatment of neonatal encephalopathy. The neurodevelopmental outcome of these infants was assessed after 18 months and showed no adverse effects in comparison to the subgroup of infants that was treated with hypothermia alone. The authors concluded that it is feasible and safe to do a randomized type II outcome study to examine a potential therapeutic benefit of xenon in newborns suffering from moderate to severe neonatal encephalopathy [22].
Mechanisms of Analgesia
Analgesic properties of xenon were first described by Lachman et al. in 1990. This study demonstrated lower fentanyl supplementation in patients who underwent xenon anesthesia for routine surgery in comparison to a control group that received nitrous oxide, which was found to have significantly higher narcotic requirements [23].
Xenon appears to be at least three times more potent in blocking the responses to surgical incision than nitrous oxide at the same concentration.
NMDA receptors are the main target of xenon and, as such, play an important role in postoperative and inflammatory pain. Inhibition of AMPA receptors via xenon might contribute to the antinociceptive effects of this gas as seen in a rat model in which inhibition of AMPA receptors in cortical neurons led to inhibition of pain sensitization [24].
Toxicity
All volatile anesthetics are harmful to the ozone layer. Nitrous oxide is a very potent greenhouse gas, being 230 times more potent than carbon dioxide. The naturally occurring noble gas xenon lacks all these harmful environmental effects.
Besides its cardio- and neuroprotective properties, xenon has no impact on coagulation and platelet function [25]. Renal and hepatic function are not affected. Xenon is not fetotoxic or teratogenic. Taken together, xenon has the features of an idle gas, yet high costs and its scarcity are currently limiting clinical use. It has been estimated that xenon’s expenses for two hours of anesthesia are around $300, whereas conventional volatile anesthetics cost around $10 for the same time use [5]. More studies are necessary to better understand how xenon anesthesia relates to clinical outcome and potential reduction in hospital stay duration and decreased need for postoperative intensive care. The development of more refined low-flow and closed-circuit systems will help to limit the costs of xenon anesthesia.
Xenon’s advantages as a neuro- and cardioprotective agent, as well as its other clinically favorable features and non-toxic chemical properties, should ultimately lead to innovations in the medical gas field that will aim to establish xenon as a readily available gas in daily anesthesia practice.
Remimazolam
Remimazolam is a new ultra-short-acting benzodiazepine that is highly selective for the GABAA receptor. It is metabolized by tissue esterases and as such promises a more predictable offset of action. Rapid onset of dose-dependent sedation and rapid recovery were demonstrated in Phase I clinical trials. Similar to remifentanyl, the short, context-sensitive half-time of remimazolam seems to be insensitive to the infusion duration. Phase II studies are currently on the way.
Etomidate Analogs
Etomidate is a well-known short-acting induction agent that provides rapid onset of anesthesia with minimal cardiovascular depression. It is metabolized in the liver by ester hydrolysis. Side-effects of etomidate are pain on injection, postoperative nausea and vomiting, myoclonus, and, most concerning of all, adrenocortical suppression via 11β-hydroxylase inhibition. Increased mortality after a single dose of etomidate has been found in critically ill patients with sepsis [26]. New analogs of etomidate were designed to address the problem of adrenocortical suppression. Three analogs are briefly introduced here.
Methoxycarbonyl (MOC) etomidate is an etomidate analog with a half-life of a few minutes. Rapid metabolism leads to accumulation of MOC etomidate carboxylic acid (MOC-ECA), which is 350 times less potent as a hypnotic metabolite and as an inhibitor of adrenocortical steroid synthesis. Follow-up studies have showed that high infusion rates are required for this drug to maintain hypnosis, which results in an accumulation of MOC-ECA with prolonged recovery times.
A more promising etomidate analog appears to be cyclopropyl MOC etomidate (CPMM). This is a spacer-linked etomidate ester which is eight times more potent than MOC etomidate. Animal studies indicate that it doesn’t suppress adrenocortical function. Clinical usefulness has yet to be investigated in humans.
MOC-carboetomidate shows potent hypnotic activity together with reduced adrenal suppression and good hemodynamic stability. However, onset time is slow due to water insolubility.
In order to improve rapid metabolism together with no adrenal suppression, as of now etomidate analogs need further optimization: Improved rapid metabolism, meaning rapid recovery from hypnosis, minimal cardiovascular suppression, and no adrenal suppression are the main goals for novel etomidate analog drug design.
Sugammadex
Sugammadex is a modified γ-cyclodextrin that was discovered in 2007. It inhibits steroidal neuromuscular blockers (NMBAs) by encapsulating them. The ability to encapsulate NMBAs is strongest for rocuronium. Sugammadex exhibits no cholinergic effects such as seen with neostigmine. The co-administration of an antimuscarinic agent such as atropine is not necessary. Multiple clinical trials reported a rapid and safe reversal of varying degrees of neuromuscular blockade. Sugammadex was approved for use in the European Union in summer 2008. Concerns about its potential side-effects on bone and tooth modeling as well as safety in pediatric and parturient populations resulted in non-approval of this drug by the FDA.
Neosaxitoxin
Neosaxitoxin (neoSTX) belongs to the group of paralytic shellfish toxins that reversibly block voltage-gated sodium channels at the neuronal level. Less cardiotoxicity in comparison to amide local anesthetic agents has been observed. This is likely due to neosaxitoxin’s lower affinity for cardiac sodium channel receptors.
Furthermore, prolonged duration of local anesthesia has been demonstrated with neoSTX: whereas commonly available local anesthetics rarely last longer than a few hours after wound infiltration, this new compound was shown to last beyond 24 hours, with a single dose injection following laparoscopic cholecystectomy [27]. No serious adverse reactions including systemic neoSTX effects were observed in a first randomized double-blind trial that was conducted in Chile. Recently, the FDA approved neoSTX for a phase I clinical study on healthy volunteers within the United States. This current trial aims to provide more refined dose escalation and safety data in line with FDA standards.
References





Full access? Get Clinical Tree
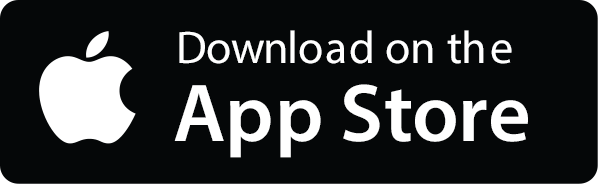
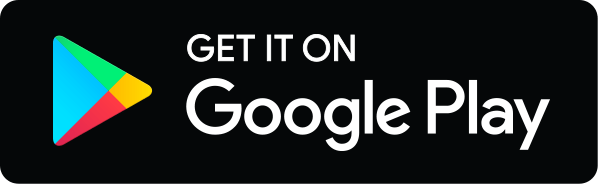
