Influence of Plasma Volume
The problem with the hematocrit and Hb concentration as measures of O2 carrying capacity is the influence of plasma volume on these variables. This is demonstrated in Figure 18.1, which shows the postural changes in hematocrit and plasma volume in a group of healthy adults (5). When changing from the standing to supine positions, there is a decrease in the hydrostatic pressure in the veins and capillaries in the legs (due to loss of the gravitational effect), and interstitial fluid moves into the bloodstream and increases the plasma volume (by 420 mL in this study). The hematocrit then decreases by dilution, but there is no change in the O2 carrying capacity of blood. The postural change in hematocrit (4.1%) is equivalent to one unit of erythrocytes (packed red blood cells), so a dilutional drop in Hct similar to the one in Figure 18.1 could be misinterpreted as indicating a decrease in O2 carrying capacity equivalent to one unit of blood.
Increased plasma volume is common in critically ill patients (see Figure 11.3 in Chapter 11), which means the hematocrit and Hb concentration will overestimate the occurrence and severity of anemia. Clinical studies have confirmed that the Hct and Hb concentration are unreliable measures of anemia in critically ill patients (6,7). Unfortunately, these are the measures used in all the clinical studies evaluating anemia and erythrocyte transfusions in critically ill patients.
FIGURE 18.1 Postural changes in hematocrit (Hct) and plasma volume (PV) in a group of healthy adults. The numbers above the columns are mean values for each measurement. From Reference 5.
ICU-Related Anemia
Two conditions have been implicated in the anemia that appears during the ICU stay (8): systemic inflammation, and repeated phlebotomy for laboratory studies.
Anemia of Inflammation
Inflammation is responsible for the anemia of chronic disease, which is now called the anemia of inflammation (8). The hematologic effects of inflammation include inhibition of erythropoietin release from the kidneys, re-duced marrow responsiveness to erythropoietin, iron sequestration in macrophages, and increased destruction of RBCs (12,13). The changes in plasma include a decrease in plasma iron, total iron binding capacity, and transferrin levels, and an increase in plasma ferritin levels.
Phlebotomy
An average of 40–70 mL of blood is withdrawn daily from each ICU patient to perform laboratory tests (9,10), which is at least 4 times more than the daily phlebotomy volume in non-ICU patients (9). The cumulative phlebotomy volume can reach 500 mL (one unit of whole blood) after one week, and this volume loss can result in an iron-deficiency anemia if allowed to continue.
The daily phlebotomy volume can be reduced by ordering fewer laboratory tests, and by reducing the volume of blood discarded for each laboratory blood draw. When blood is withdrawn through vascular catheters for laboratory testing, the initial aspirate is discarded to eliminate interference from intravenous fluid in the lumen of the catheter. The volume of discarded blood is typically about 5 mL for each laboratory blood draw, and returning this blood to the patient can reduce the daily phlebotomy volume by 50% (11).
Physiological Effects of Anemia
Anemia elicits two responses that help to preserve tissue oxygenation: (a) an increase in cardiac output, and (b) an increase in O2 extraction from capillary blood.
Cardiac Output
The graph in Figure 9.9 (page 166) shows the increase in cardiac output that occurs in response to a progressive decrease in hematocrit. This response is explained by the influence of anemia on blood viscosity. The hematocrit is the principal determinant of blood viscosity, and decreases in hematocrit are accompanied by similar decreases in blood viscosity. The relationship between hematocrit and blood viscosity is shown in Table 9.2 (page 165). The final section of Chapter 9 contains a detailed description of blood viscosity and its influence on circulatory blood flow.
Systemic Oxygenation
The influence of progressive anemia on measures of systemic oxygenation are shown in Figure 18.2 (12). The principal findings are explained using the following relationships between O2 uptake (VO2), O2 delivery (DO2), and O2 extraction:
(18.1)
1. The progressive decrease in hematocrit is associated with a steady decrease in O2 delivery (DO2). However, there is also an equivalent increase in O2 extraction, and the reciprocal changes in DO2 and O2 extraction results in an unchanged O2 uptake (VO2).
2. When the hematocrit falls below 10%, the increase in O2 extraction is no longer able to match the decrease in DO2, and the VO2 begins to fall. The decrease in VO2 represents a decrease in O2 availability in tissues, and is accompanied by lactate accumulation in the blood.
3. The maximum O2 extraction is about 50%, and it marks the threshold for impaired tissue oxygenation. Therefore, an O2 extraction of 50% could be used as a trigger point for transfusion of erythrocytes. This point will be revisited later in the chapter.
Lowest Tolerable Hematocrit
Animal studies have shown that, when the intravascular volume is maintained, hematocrits as low as 5 to 10% (Hb=1.5 to 3 g/dL) do not adversely affect tissue oxygenation (12–14), even in awake animals breathing room air (14). The lowest tolerable hematocrit or hemoglobin has not been determined in humans, but in one study of progressive hemodilution in healthy adults, Hb levels of 5 g/dL showed no apparent harm (15). The important message from studies of severe anemia is not the lowest tolerable hematocrit, but the realization that severe anemia is tolerated when the intravascular volume is maintained.
FIGURE 18.2 The influence of progressive isovolemic anemia on measures of systemic oxygenation. DO2 = systemic O2 delivery, VO2 = systemic O2 uptake. Data from Reference 12.
Paradoxical Effect
The graph in Figure 18.3 reveals an effect of anemia that few would predict; i.e., enhanced tissue oxygenation! The data in this graph is from a study that used direct measurements of the PO2 in subcutaneous tissue to evaluate normovolemic hemodilution in isolated skin flaps (16). As indicated in the graph, progressive decreases in hematocrit were accompanied by increases in the subcutaneous PO2 in both normal and ischemic skin regions, and this relationship continued until the hematocrit fell to 15%. Similar results in other studies has led to the use of normovolemic anemia to promote the viability of skin flaps.
FIGURE 18.3 The effect of progressive isovolemic anemia on the subcutaneous PO2 in normal and ischemic regions of the skin. From Reference 16.
So how can anemia improve tissue oxygenation? Only if the flow augmentation in response to anemia is greater than the decrease in hematocrit. Exaggerated flow responses to anemia have been reported in the coronary and cerebral circulations (17), which may be a protective mechanism for the heart and brain, but the effect on tissue oxygenation is not known. Regional variations in the flow response to anemia add further to the problem of selecting an appropriate transfusion trigger.
TRANSFUSION TRIGGERS
Surveys indicate that 90% of the erythrocyte transfusions in ICU patients are given to alleviate anemia (18), and are guided by the hemoglobin concentration in blood. This is a flawed practice, as described in this section.
Hemoglobin
In 1942, a hemoglobin (Hb) level <10 g/dL was recommended as an indication for erythrocyte transfusions (19), and this was the standard transfusion trigger for the next 60 years, until clinical studies showed that adopting a lower transfusion trigger (i.e., Hb <7 g/dL) had no adverse consequences (20,21). This lower transfusion trigger is now included in the clinical practice guidelines for RBC transfusions.
Guidelines
The most recent guidelines on erythrocyte transfusions in critically ill patients (3) contain the following statement:
1. The use of only the Hb level as a “trigger” for transfusion should be avoided (italics mine)
Despite this recommendation, the guidelines also contain the following inconsistent statements:
2. Consider transfusion if Hb <7 g/dL in critically ill patients who require mechanical ventilation.
3. Consider transfusion if Hb <7 g/dL in resuscitated critically ill trauma patients.
4. Consider transfusion if Hb <7 g/dL in critically ill patients with stable cardiac disease.
5. RBC transfusion may be beneficial in patients with acute coronary syndromes (ACS) who are anemic (Hb <8 g/dL).
What’s Wrong
There are two fundamental problems with the Hb as a transfusion trigger.
1. The Hb concentration in blood provides absolutely no information about the adequacy of tissue oxygenation, so transfusions based on the Hb level have no relationship to tissue oxygenation.
2. Decreases in the Hb concentration can be a dilutional effect, and may not reflect decreases in the O2 carrying capacity of blood.
Clinical practice guidelines published over the past 25 years have recommended abandoning the Hb level as a transfusion trigger and adopting more physiologic measures of tissue oxygenation, like the ones described next (3,22). However, instead of abandoning the Hb level, physicians have abandoned the recommendation!
Oxygen Extraction
As described earlier (and shown in Figure 18.2) anemia elicits a compensatory increase in O2 extraction from capillary blood, which serves to maintain a constant rate of O2 uptake into tissues. However, the O2 extraction cannot increase much further than 50%, and, when the O2 extraction is maximum at 50%, further decreases in Hb are accompanied by proportional decreases in O2 uptake into tissues (indicating tissue dysoxia). Therefore, an O2 extraction of 50% can be used as a transfusion trigger because it identifies the threshold for impaired tissue oxygenation. The O2 extraction is roughly equivalent to the (SaO2–ScvO2), and can be monitored continuously using pulse oximetry (for the SaO2) and a central venous oximetry catheter (PreSep Catheter, Edwards Life sciences) to monitor the central venous O2 saturation (ScvO2). The (SaO2–ScvO2) is very appealing as a transfusion trigger (23) because it provides information about the adequacy of tissue oxygenation.
Central Venous O2 Saturation
When the SaO2 is close to 100%, the (SaO2–ScvO2) is equivalent to (1–ScvO2), and the ScvO2 can be used as a transfusion trigger. An ScvO2 <70% has been proposed as a transfusion trigger (24), although it seems a lower ScvO2 (i.e., closer to 50%) would be more appropriate for identifying the threshold for impaired tissue oxygenation.
ERYTHROCYTE TRANSFUSIONS
Whole blood is stored only on request, and is otherwise separated into its component parts; i.e., red blood cells, platelets, plasma, and cryoprecipitate. This practice allows each unit of donated blood to serve multiple transfusion needs. The erythrocyte preparations that are available for transfusion are shown in Table 18.2.
Table 18.2 Erythrocyte Transfusion Preparations
Packed Red Blood Cells
The erythrocyte fraction of donated blood is placed in a preservative fluid and stored at 1 to 6°C. Newer preservative solutions contain adenine, which helps to maintain ATP levels in stored erythrocytes, and allows storage of donor erythrocytes for up to 42 days (25). Each unit of donor erythrocytes, known as packed red blood cells (packed RBCs), has a hematocrit of about 60% and a volume of about 350 mL. Packed RBCs also contain 30–50 mL of residual plasma, and a considerable numbers of leukocytes (1–3 billion leukocytes per unit of packed RBCs) (25).
Leukocyte Reduction
The leukocytes in packed RBCs can trigger an antibody response in the recipient after repeated transfusions, and this is responsible for febrile nonhemolytic transfusion reactions (see later). To reduce the occurrence of this reaction, donor RBCs are passed through specialized filters to remove most of the leukocytes. This is performed routinely in many blood banks, but universal leukocyte reduction has yet to be adopted in the United States. Leukocyte-reduced RBCs are recommended for pa-tients with prior febrile nonhemolytic transfusion reactions (25).
Washed Red Blood Cells
Donor RBCs can be washed with isotonic saline to remove residual plasma. This reduces the risk of hypersensitivity reactions caused by prior sensitization to plasma proteins in donor blood. Washed RBC preparations are recommended for patients with a history of hypersensitivity reactions to blood transfusions, and for patients with immunoglobulin A deficiency, who have an increased risk of transfusion-related anaphylaxis (25). Saline washing does not effectively remove leukocytes.
Infusing Packed RBCs
The infusion of packed RBCs is described in Chapter 11 (see page 205). Rapid infusion rates are not necessary when packed RBCs are used to alleviate anemia, and this eliminates the need for infusion pumps or dilution with saline to increase infusion rates. The gravity-driven flow rate of packed RBCs through an 18-gauge peripheral catheter is 5 mL/min (see Figure 11.5 on page 206), which corresponds to 70 minutes for the transfusion of one unit (350 mL) of packed RBCs. This is well within the recommended transfusion time of 2 hrs per unit of packed RBCs for hemodynamically stable patients (26).
Blood Filters
Standard blood filters (pore size 170 to 260 microns) are required for the transfusion of all blood products (26). These filters trap blood clots and other debris, but they do not trap leukocytes, and are not effective for leukocyte reduction (25). These filters can become an impediment to flow as they collect trapped debris, and sluggish infusion rates should prompt replacement of the blood filter.
Systemic Oxygenation
In an average sized adult, one unit of packed RBCs is expected to raise the hemoglobin concentration and hematocrit by 1 g/dL and 3%, respectively (25). The effects of RBC transfusions on measures of systemic oxygenation are shown in Figure 18.4. The data in this figure is from a group of postoperative patients with severe normovolemic anemia (Hb <7 g/dL) who were transfused with 1–2 units of packed RBCs to raise the Hb above 7 g/dL. The RBC transfusions increased the mean Hb concentration from 6.4 to 8 g/dL (25% increase), and there was a similar increase in O2 delivery (DO2). However, the systemic O2 uptake (VO2) was unchanged. The constant VO2 in the face of an increased DO2 indicates that O2 extraction was reduced by the RBC transfusions, as predicted by equation 18.1. These changes in DO2 and O2 extraction are the reverse of the changes produced by anemia, as shown in Figure 18.2.
Tissue Oxygenation
The lack of an effect on VO2 indicates that RBC transfusions do not en-hance tissue oxygenation. This has been confirmed in several clinical studies (27–30), and prolonged storage of RBCs can actually impair tissue oxygenation after transfusion (31). These studies have prompted the following statement in the most recent clinical practice guidelines for red blood cell transfusions (3): “RBC transfusion should not be considered an absolute method to improve tissue oxygenation in critically ill patients.” If RBC transfusions do not provide a benefit for tissue oxygenation, then why do we give RBC transfusions? Unfortunately, there is no satisfying answer to this question.
FIGURE 18.4 Effects of erythrocyte transfusions (1–2 units packed RBCs) on hemoglobin concentration (Hb), systemic oxygen delivery (DO2), and systemic oxygen uptake (VO2) in 11 postoperative patients with severe anemia (Hb <7 g/dL). Data points represent mean values for each parameter. Numbers in parentheses are the mean hemoglobin concentrations before and after transfusion. Data from personal observations.
The inability of RBC transfusions to enhance tissue oxygenation raises serious questions about the practice of transfusing RBCs simply to raise the blood Hb level. Adding to the concern are studies indicating that RBC transfusions are a source of morbidity and even mortality in critically ill patients (32). The risks associated with RBC transfusions are described next.
TRANSFUSION RISKS
The spectrum of adverse events associated with blood transfusions are shown in Table 18.3, along with the incidence of each event expressed in relation to the number of units transfused (33–37). (Only acute events are included.) Note that transfusion errors are much more frequent than the feared transmission of HIV or the hepatitis B virus. The following is a brief description of the principal transfusion reactions (37–41).
Table 18.3 Adverse Events Associated with RBC Transfusions (per units transfused)
Acute Hemolytic Reactions
Acute hemolytic reactions are prompted by the transfusion of RBCs that are ABO-incompatible with the recipient. When this occurs, antibodies in recipient blood bind to ABO antigens on the donor RBCs , and the ensuing lysis of donor RBCs triggers a systemic inflammatory response that can be accompanied by hypotension and multiorgan failure. These reactions are usually the result of human error.
Clinical Features
The hallmark of acute hemolytic reactions is the abrupt onset of fever, dyspnea, chest pain, low back pain, and hypotension within minutes after starting the transfusion. Severe reactions are accompanied by a consumptive coagulopathy and progressive multiorgan dysfunction.
Management
1. If a hemolytic reaction is suspected, STOP the transfusion immediately and verify that the correct blood was given to the correct patient. It is imperative to stop the transfusion as soon as possible because the severity of hemolytic reactions is a function of the volume of blood transfused (33).
2. If the donor blood is correctly matched to the patient, an acute hemolytic reaction is unlikely. However, the blood bank must be notified, and they will ask for blood samples to perform a plasmafree hemoglobin determination (for evidence of intravascular hemolysis) and a direct Coomb’s test (for evidence of the anti-ABO antibody).
3. If an acute hemolytic reaction is confirmed, support blood pressure and ventilation as needed. The management of severe hemolytic reactions is similar to septic shock (i.e., volume resuscitation and a vasopressor, if necessary) because inflammation is the culprit in both conditions. Most patients with hemolytic reactions should survive the condition.
Febrile Nonhemolytic Reactions
A febrile nonhemolytic transfusion reaction is defined as a temperature elevation >1°C (1.8°F) that occurs during transfusion or up to 6 hours after transfusion, and is not attributed to another cause (e.g., acute hemolytic reaction) (35). The culprit is the presence of antileukocyte antibodies in recipient blood that react with antigens on donor leukocytes. This triggers the release of endogenous pyrogens from phagocytes, which is the source of the fever. This reaction is reported in 0.5% of RBC transfusions (once per 200 transfusions), and occurs in patients who have received prior transfusions, and in multiparous women. Transfusion of leukocyte-reduced RBCs reduces, but does not eliminate, the risk of this reaction (35).
Clinical Features
The fever typically does not appear in the first hour after the start of transfusion (unlike the fever associated with acute hemolytic reactions), and it can be accompanied by rigors and chills.
Management
1. The initial approach to transfusion-related fever is the same as described for hemolytic transfusion reactions, even though the fever may not appear until after the transfusion is completed. The diagnosis is confirmed by excluding the presence of hemolysis with the tests described previously.
2. The blood bank will perform a Gram stain on the donor blood, and may request blood cultures on the recipient. This is usually unrewarding because microbial contamination in stored blood is rare (1 per 5,000,000 units). The organism most frequently isolated in stored RBCs is Yersinia enterocolitica (34).
3. More than 75% of patients with a nonhemolytic fever will not experience a similar reaction to subsequent transfusions (34). Therefore, no special precautions are needed for future transfusions. If a second febrile reaction occurs, leukocyte-reduced RBCs are advised for all subsequent transfusions.
Hypersensitivity Reactions
Hypersensitivity reactions are the result of sensitization to plasma proteins in donor blood from prior transfusions. Patients with IgA deficiency are prone to hypersensitivity transfusion reactions, and prior exposure to plasma products is not required. The most common hypersensitivity reaction is urticaria, which is reported in one of every 100 units transfused (36). More severe anaphylactic reactions (e.g., bronchospasm) are much less common, and anaphylactic shock is rare.
Clinical Features
The usual manifestation is mild urticaria that appears during the transfusion and is not accompanied by fever. The abrupt onset of dyspnea during a transfusion could represent laryngeal edema or bronchospasm, and hypotension from anaphylactic shock can be mistaken for an acute hemolytic reaction.
Management
1. Mild urticaria without fever does not require interruption of the transfusion. However, the popular practice is to stop the transfusion temporarily and administer an antihistamine for symptom relief (e.g., diphenhydramine, 25–50 mg PO, IM, or IV).
2. Severe anaphylactic reactions should be managed as described in Chapter 14. The transfusion should be stopped immediately if severe anaphylaxis is suspected.
3. Washed RBCs should be used for all future transfusions in patients with hypersensitivity reactions. However, in patients with severe anaphylactic reactions, future transfusions are risky, even with washed RBCs, and should be avoided unless absolutely necessary.
4. Patients who develop hypersensitivity reactions should be tested for an underlying IgA deficiency.
Acute Lung Injury
Transfusion-related acute lung injury (TRALI) is an inflammatory lung injury associated with RBC and platelet transfusion (38), and resembles the acute respiratory distress syndrome (ARDS), which is described in Chapter 23. Recent surveys show an incidence of 1 per 12,000 transfusions (38), and a mortality rate of 6% (37). TRALI is considered the leading cause of transfusion-related deaths (37).
Etiology
The prevailing theory is that TRALI is the result of antileukocyte antibodies in donor blood that bind to antigens on circulating neutrophils in the recipient. This triggers neutrophil activation, and the activated neutrophils become sequestered in pulmonary capillaries and migrate into the lungs to produce the inflammatory injury. The risk of TRALI is higher when donor blood contains high levels of antileukocyte antibodies, and in donor blood from females (37). The link between female blood and TRALI is not well understood.
Clinical Features
Signs of respiratory compromise (dyspnea, tachypnea, hypoxemia, etc.) can appear for up to 6 hours after the start of a transfusion, but they usually appear within the first hour after the transfusion begins (37). Fever is common, and the chest x-ray eventually looks like the one in Figure 18.5, with diffuse, homogeneous infiltrates in both lungs that are indistinguishable from ARDS. The diagnosis of TRALI is based on the clinical setting (i.e., ARDS that appears within 6 hours after the start of a blood transfusion). The chest x-ray can be mistaken for acute hydrostatic pulmonary edema, but this can be excluded based on the clinical setting. TRALI can be severe at the outset, and often requires mechanical ventilation, but the condition typically resolves within a week (37).
FIGURE 18.5 Portable chest film from a patient with transfusion-related acute lung injury. Note the homogeneous pattern of infiltration in the lungs, and the fine “ground-glass” appearance of the infiltrates, which are characteristics of inflammatory lung injury.
Management
1. If the transfusion is not completed, it should be stopped at the first signs of respiratory difficulty. The blood bank should be notified for all cases of TRALI. (Assays for antileukocyte antibodies are available, but are not currently used in the diagnostic evaluation of TRALI.)
2. The management of TRALI is supportive, and is very similar to the management of ARDS described in Chapter 23.
3. There are no firm recommendations regarding future transfusions in patients who develop TRALI. Some recommend using washed RBCs to remove antibodies from donor blood, but the effectiveness of this measure is not known.
Nosocomial Infections
The immunosuppressive effects of blood transfusions became evident with the discovery (in the early 1970s) that pre-transplant blood transfusions improved the survival rate of renal allografts (39). Since then, a multitude of clinical studies have shown that patients who receive blood transfusions have a higher incidence of nosocomial infections (32,39–41). The risk of infection increases with the volume of blood transfused (see Figure 18.6), and with the storage time of donor blood (42). There has been some concern that the association between blood transfusions and infection is not a causal relationship, but instead is a reflection of severity of illness (i.e., sicker patients develop more infections and they also require more blood transfusions). However, at least 22 studies have shown that blood transfusion is an independent risk factor for nosocomial infections (32).
Transfusion-related immunosuppression is poorly understood, but the emerging opinion is that nosocomial infections are a major source of transfusion-related morbidity and mortality in critically ill patients (32).
Clinical Outcomes
A review of 45 clinical studies evaluating RBC transfusions in critically ill patients, which included 272,596 patients, revealed the following findings (32):
1. In 42 of the 45 studies, the adverse effects of RBC transfusions outweighed any benefits.
2. Only 1 of 45 studies showed that the benefits of RBC transfusions outweighed the adverse effects.
3. Eighteen studies evaluated the relationship between RBC transfusions and survival, and 17 of the 18 studies showed that RBC transfusions were an independent risk factor for death. The likelihood of a fatal outcome was, on average, 70% higher in patients who re-ceived an RBC transfusion.
Not a very good report card, is it? These observations, combined with those showing that RBC transfusions do not improve tissue oxygenation, suggest that the current practice of transfusing RBCs to increase the Hb concentration in blood may simply be bad medicine.
FIGURE 18.6 Results of a large multicenter study showing the relationship between the incidence of nosocomial infections and the volume of blood transfused in critically ill patients. N = number of patients in the study. From Reference 40.
A FINAL WORD
Blood Volume vs. RBCs
The practice of transfusing RBCs to raise the Hb level in blood is rooted in the belief that anemia is a threat to tissue oxygenation. However, as described earlier in the chapter, the severest anemias do not threaten tissue oxygenation as long as the intravascular volume (and hence cardiac output) is maintained. The supremacy of blood volume over RBCs in supporting tissue oxygenation is evident when you consider that hypovolemia is a recognized cause of impaired tissue oxygenation (i.e., hypovolemic shock), but anemia is not (i.e., “anemic shock” is not a clinical entity). The importance of blood volume is often overlooked, even by the American Red Cross, whose popular slogan, blood saves lives, deserves a more accurate update, as shown in Figure 18.7. Awareness of the attributes of blood volume would help to curb the undeserved emphasis on the transfusion of RBCs to support tissue oxygenation.
FIGURE 18.7 A popular slogan of the American Red Cross on the left, and an amended version on the right that recognizes the contribution of intravascular volume in the survival benefit of blood transfusions.
REFERENCES
King KE (ed). Blood Transfusion Therapy: A Physician’s Handbook. 9th ed. Bethesda, MD: American Association of Blood Banks, 2008.
Introduction
1. Hebert PC, Tinmouth A, Corwin HL. Controversies in RBC transfusions in the critically ill. Chest 2007; 131:1583–1590.
2. Bennet-Guerrero E, Zhao Y, O’Brien SM, et al. Variation in the use of blood transfusion in coronary artery bypass graft surgery. JAMA 2010; 304:1568–1575.
Clinical Practice Guidelines
3. Napolitano LM, Kurek S, Luchette FA, et al. Clinical practice guideline: Red blood cell transfusion in adult trauma and critical care. Crit Care Med 2009; 37:3124–3157.
4. Ferraris VA, Ferraris SP, Saha SP, et al. Perioperative blood transfusion and blood conservation in cardiac surgery; the Society of Thoracic Surgeons and the Society of Cardiovascular Anesthesiologists Clinical Practice Guideline. Ann Thorac Surg 2007; 83(suppl):S27–S86.
Anemia in the ICU
5. Jacob G, Raj SR, Ketch T, et al. Postural pseudoanemia: posture-dependent change in hematocrit. Mayo Clin Proc 2005; 80:611-614.
6. Jones JG, Holland BM, Wardrop CAJ. Total circulating red cells versus hematocrit as a primary descriptor of oxygen transport by the blood. Br J Hematol 1990; 76:228–232.
7. Cordts PR, LaMorte WW, Fisher JB, et al. Poor predictive value of hematocrit and hemodynamic parameters for erythrocyte deficits after extensive elective vascular operations. Surg Gynecol Obstet 1992; 175:243–248.
8. Prakash D. Anemia in the ICU. Crit Care Clin 2012; 28:333–343.
9. Smoller BR, Kruskall MS. Phlebotomy for diagnostic laboratory tests in adults: Pattern of use and effect on transfusion requirements. N Engl J Med 1986; 314:1233–1235.
10. Corwin HL, Parsonnet KC, Gettinger A, et al. RBC transfusion in the ICU: Is there a reason? Chest 1995; 108:767–771.
11. Silver MJ, Li Y-H, Gragg LA, et al. Reduction of blood loss from diagnostic sampling in critically ill patients using a blood-conserving arterial line system. Chest 1993; 104:1711-1715.
12. Wilkerson DK, Rosen AL, Gould SA, et al. Oxygen extraction ratio: a valid indicator of myocardial metabolism in anemia. J Surg Res 1987; 42:629–634.
13. Levine E, Rosen A, Sehgal L, et al. Physiologic effects of acute anemia: implications for a reduced transfusion trigger. Transfusion 1990; 30:11–14.
14. Nielsen VG, Baird MS, Brix A, Matalon S. Extreme, progressive isovolemic hemodilution with 5% albumin, PentaLyte, or hextend does not cause hepatic ischemia or histologic injury in rabbits. Anesthesiology 1999; 90:1428–1435.
15. Weiskopf RB, Viele M, Feiner J, et al. Human cardiovascular and metabolic response to acute, severe, isovolemic anemia. JAMA 1998; 279:217–221.
16. Hansen ES, Gellett S, Kirkegard L, et al. Tissue oxygen tension in random pattern skin flaps during normovolemic hemodilution. J Surg Res 1989; 47:24–29.
17. Hebert PC, McDonald BJ, Tinmouth A. Clinical consequences of anemia and red cell transfusion in the critically ill. Crit Care Clin 2004; 20:225–235.
Transfusion Triggers
18. Corwin HL, Gettinger A, Pearl R, et al. The CRIT study: anemia and blood transfusion in the critically ill – Current clinical practice in the United States. Crit Care Med 2004; 32:39–52.
19. Adam RC, Lundy JS. Anesthesia in cases of poor risk: Some suggestions for decreasing the risk. Surg Gynecol Obstet 1942; 74:1011–1101.
20. Hebert PC, Wells G, Blajchman MA, et al. A multicenter, randomized, controlled clinical trial of transfusion requirements in critical care. N Engl J Med 1999; 340:409–417.
21. Hebert PC, Yetisir E, Martin C, et al. Is a low transfusion threshold safe in critically ill patients with cardiovascular disease. Crit Care Med 2001; 29:227–234.
22. Consensus Conference on Perioperative Red Blood Cell Transfusion. JAMA 1988; 260:2700–2702.
23. Levy PS, Chavez RP, Crystal GJ, et al. Oxygen extraction ratio: a valid indicator of transfusion need in limited coronary vascular reserve? J Trauma 1992; 32:769–774.
24. Vallet B, Robin E, Lebuffe G. Venous oxygen saturation as a physiologic transfusion trigger. Crit Care 2010; 14:213–217.
Erythrocyte Transfusions
25. King KE (ed). Blood Transfusion Therapy: A Physician’s Handbook. 9th ed. Bethesda, MD: American Association of Blood Banks, 2008:1–18.
26. Ibid, pp. 91–95.
27. Conrad SA, Dietrich KA, Hebert CA, Romero MD. Effects of red cell transfusion on oxygen consumption following fluid resuscitation in septic shock. Circ Shock 1990; 31:419–429.
28. Dietrich KA, Conrad SA, Hebert CA, et al. Cardiovascular and metabolic response to red blood cell transfusion in critically ill volume-resuscitated nonsurgical patients. Crit Care Med 1990; 18:940–944.
29. Marik PE, Sibbald W. Effect of stored-blood transfusion on oxygen delivery in patients with sepsis. JAMA 1993; 269:3024–3029.
30. Fuller BM, Gajera M, Schorr C, et al. Transfusion of packed red blood cells is not associated with improved central venous oxygen saturation or organ function in patients with septic shock. J Emerg Med 2012; 43:593–598.
31. Kiraly LN, Underwood S, Differding JA, Schreiber MA. Transfusion of aged packed red blood cells results in decreased tissue oxygenation in critically ill trauma patients. J Trauma 2009; 67:29–32.
32. Marik PE, Corwin HL. Efficacy of red blood cell transfusion in the critically ill: A systematic review of the literature. Crit Care Med 2008; 36:2667–2674.
Transfusion Risks
33. Kuriyan M, Carson JL. Blood transfusion risks in the intensive care unit. Crit Care Clin 2004; 237–253.
34. Goodnough LT. Risks of blood transfusion. Crit Care Med 2003; 31:S678–S686.
35. King KE (ed). Acute transfusion reactions. In: Blood Transfusion Therapy: A Physician’s Handbook 9th ed. Bethesda, MD: American Association of Blood Banks, 2008:148–173.
36. Greenberger PA. Plasma anaphylaxis and immediate-type reactions. In: Rossi EC, Simon TL, Moss GS (eds). Principles of transfusion medicine. Philadelphia: Williams & Wilkins, 1991:635–639.
37. Transfusion reactions: newer concepts on the pathophysiology, incidence, treatment, and prevention of transfusion-related acute lung injury. Crit Care Clin 2012; 28:363–372.
38. Toy P, Gajic O, Bachetti P, et al. Transfusion-related acute lung injury: incidence and risk factors. Blood 2012; 119:1757–1767.
39. Vamvakas EC, Blajchman MA. Transfusion-related immunomodulation (TRIM): an update. Blood Rev 2007; 21:327–348.
40. Agarwal N, Murphy JG, Cayten CG, Stahl WM. Blood transfusion increases the risk of infection after trauma. Arch Surg 1993; 128:171–177.
41. Taylor RW, O’Brien J, Trottier SJ, et al. Red blood cell transfusions and nosocomial infections in critically ill patients. Crit Care Med 2006; 34:2302–2308.
42. Juffermans NP, Prins DJ, Viaar AP, et al. Transfusion-related risk of secondary bacterial infections in sepsis patients: a retrospective cohort study. Shock 2011; 35:355–359.

Full access? Get Clinical Tree
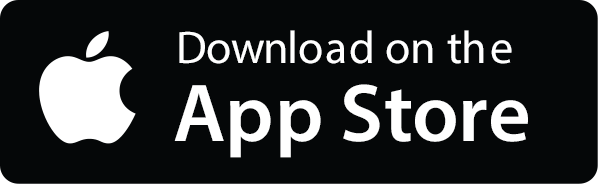
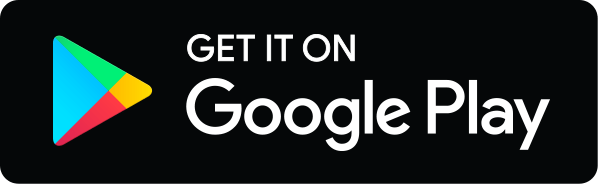