Annette Vegas1 and Wanda C. Miller‐Hance2,3 1 Department of Anesthesia and Pain Management, Toronto General Hospital, Toronto, Ontario, Canada 2 Department of Anesthesiology, Perioperative and Pain Medicine, Arthur S. Keats Division of Pediatric Cardiovascular Anesthesiology, Baylor College of Medicine and Texas Children’s Hospital, Houston, TX, USA 3 Department of Pediatrics, Section of Cardiology, Baylor College of Medicine and Texas Children’s Hospital, Houston, TX, USA Currently, most institutions use a combination of imaging modalities, including echocardiography, cardiac catheterization and angiography, computed tomography (CT), and cardiac magnetic resonance (CMR), for the comprehensive diagnostic evaluation of patients with congenital heart disease (CHD). Transesophageal echocardiography (TEE) has evolved over time to become a critically important cardiovascular imaging modality that has major benefits, particularly in the perioperative setting. Since the introduction of TEE in the late 1980s, it has proven useful as a diagnostic and monitoring tool in adult patients during cardiac and noncardiac surgery. Until the early 1990s, intraoperative TEE in infants and small children was not feasible, as TEE probes of a suitable size were unavailable. Advances in ultrasonography technology, specifically the miniaturization of imaging probes, have since permitted the safe use of TEE in these patients. Most cardiac centers currently consider TEE to be the standard of care for assessing the adequacy of surgical interventions in children and adults with CHD. This imaging approach can immediately detect suboptimal surgical results, thus improving clinical outcomes by avoiding subsequent reoperations and reducing overall morbidity, mortality, and costs. The use of TEE in environments such as the cardiac catheterization laboratory, electrophysiology suite, and intensive care unit (ICU) also significantly facilitates the management of patients with CHD. Although TEE is not commonly used for purely diagnostic indications in children, many adults with CHD and challenging images benefit significantly from this use of TEE. Epicardial echocardiography (E‐echo), which directly places the probe on the heart surface, provides an option to image the heart when TEE is unavailable or contraindicated, or when this modality can provide information that complements the TEE findings in the operating room or ICU. This chapter focuses on the use of TEE and E‐echo for monitoring and assessment in CHD patients, highlighting these modalities’ many benefits and roles in clinical practice. The emphasis is on intraoperative use, as this is the most common application of the technology in CHD patients. Echocardiography is the preferred imaging modality for the initial diagnostic assessment and serial follow‐up of patients with CHD. Transthoracic echocardiography (TTE) provides excellent definition of cardiovascular anatomy in most patients. In fact, the exceptionally high resolution of TTE imaging in infants and young children with CHD is often sufficient to plan medical and surgical management. Published standards exist for the echocardiographic assessment of pediatric and adult patients with CHD, including TTE, TEE, quantification, and 3D imaging [1–5]. Despite the merits of TTE, its technical limitations result in suboptimal imaging in patients with poor windows caused by obesity, mechanical ventilation, and prior or recent cardiac surgery. TEE overcomes many of these limitations and can be extremely helpful when other imaging approaches, including TTE, provide insufficient information or there is a need to answer important diagnostic questions. The portability of TEE makes it easy to perform in various settings. TEE in the patient with CHD is often an adjunct to other diagnostic studies and, as such, should complement, but not substitute for, a complete TTE examination. Several professional societies in North America and other countries have proposed indications for the use of TEE [3, 6–9]. Guidelines published by the American Society of Echocardiography (ASE) in 2005 and 2019 address specific indications for TEE in pediatric patients and in all patients with CHD [5, 10]. These documents describe three major categories of indications for TEE in CHD patients: diagnostic assessment, perioperative evaluation, and related to percutaneous interventions (Table 15.1). Outpatient TEE is a routine diagnostic tool used in many centers that care for adult patients with cardiovascular disease, including CHD, because transthoracic imaging becomes more challenging and less revealing as patients reach adulthood [11]. The TEE modality plays an important role in this setting, as congenital anomalies are highly variable in presentation. The benefits of TEE include the detailed evaluation of primary structural malformations, secondary compensatory cardiovascular changes, associated complications, and functional and hemodynamic consequences. The proximity of the imaging probe to the back of the heart provides optimal visualization of cardiovascular structures. Overall, TEE is superior to TTE for diagnosing specific pathologies involving the aorta (Ao) and left atrial appendage (LAA) and for evaluating prosthetic heart valves, native valve masses, and complications resulting from endocarditis, including vegetations, abscesses, and fistulae [3]. It is the imaging approach of choice to exclude thrombus before performing elective cardioversion in most patients with atrial rhythm disturbances. The TEE imaging approach is also useful in evaluating potential cardiac sources of embolism, such as a patent foramen ovale (PFO) [12]. The modality can also enhance the assessment of intracardiac or extracardiac baffles in patients who have undergone Fontan, Senning, or Mustard procedures [13, 14]. Intraoperative evaluation represents a frequent application of TEE and has the following goals [15]: Table 15.1 Indications for transesophageal echocardiography (TEE) in the patient with congenital heart disease (CHD) Source: Puchalski et al. [5]. Reproduced with permission of Elsevier. A summary of the indications in both adults and children with CHD includes [5]: TEE may provide significant benefits in patients with CHD undergoing cardiac catheterization procedures, such as device closure of intracardiac communications (atrial septal defects [ASDs] or ventricular septal defects [VSDs]), balloon valvuloplasty, perforation of the atretic pulmonary valve, and atrial septostomy [16–19]. Likewise, TEE can be extremely useful during electrophysiologic procedures by guiding catheter placement and assisting with ablation therapies [20, 21]. In these various applications, TEE can offer valuable information that facilitates planning, refinement, and, upon completion of the intervention, real‐time assessment of the results. Despite its considerable merits, TEE has inherent limitations, including the following: Before using transesophageal imaging, it is prudent to carefully consider the indications, exclude contraindications, obtain informed consent, and completely review relevant clinical information, including all available echocardiographic studies and other diagnostic data. Given the potential risks associated with this semi‐invasive procedure, the need for TEE requires judicious decision‐making for every patient. In any clinical situation in which safety concerns exist, one should consider alternate imaging modalities such as E‐echo, discussed later in this chapter. General contraindications to TEE include esophageal pathology, severe respiratory decompensation, and inadequate control of the airway (Table 15.2) [3, 5]. The risk‐to‐benefit ratio for TEE warrants cautious assessment in patients with cervical spine injury or deformity and those with severe coagulopathy. The presence of a gastrostomy feeding tube often necessitates deferring the transgastric (TG) and deep transgastric (DTG) examinations. Although there is no established timing for safe esophageal instrumentation after esophageal surgery, anecdotally, many children have undergone TEE imaging several months or years later without notable ill effects. Table 15.2 Contraindications to transesophageal echocardiography Source: Puchalski et al. [5]. Reproduced with permission of Elsevier. Using echocardiography requires knowing the science of ultrasound and the many important principles involved. The application of TEE assumes a thorough understanding of the instrumentation needed to perform the examination. This chapter cannot address these aspects of echocardiography and TEE practice, even in an abbreviated form; the interested reader is referred to detailed resources on the subject [22–28]. Ultrasonography machines suitable for echocardiography incorporate multiple modalities for image and signal processing during interrogation of the heart and vasculature. Modern systems integrate components such as a high‐resolution display, a console with hard keys, connection ports for transducers, ports for physiologic monitors (e.g., electrocardiogram), and a computer with software for processing data and network ports. Controls and their layout on the machine vary by manufacturer; nonetheless, some common features among systems merit a basic understanding of their function. By manipulating various knobs, one can adjust image quality, activate different modes, record images, and perform other functions. Understanding how the machine operates is essential for acquiring images of diagnostic quality. Discussions of these important aspects of echocardiography are available in print [25, 26] and in a number of web‐based resources. Several TEE probes are commercially available for clinical use (Table 15.3). In general, probes are not interchangeable among different echocardiographic systems and may not even be compatible between machines across the same platform. Using a TEE probe in a particular system requires not only appropriate hardware compatibility but also specific software to interface the imaging device with the echocardiography machine. The evolution of transducer technology has produced TEE probes that have higher resolution than the first probes, as well as enhanced image plane options and additional modes to better assess the cardiovascular system. These advances represent major improvements in our ability to evaluate complex disease comprehensively. Early monoplane (single‐plane) TEE probes provided imaging only in the transverse orientation or horizontal plane (0°) [29]. Biplane probes incorporated a second scanning crystal that enabled, in addition, imaging in the longitudinal orientation or vertical plane (90°), thereby providing information in planes perpendicular to each other (i.e., orthogonal planes) [30–34]. The introduction of multiplane (omniplane) probes permitted interrogation of cardiovascular anatomy in any plane between 0° and 180° [35–38]. These flexible devices all contain phased‐array transducers that produce “pie‐shaped” or sector images. Sophisticated multiplane probes with many imaging planes to visualize most cardiac structures have largely replaced monoplane and biplane devices (Figure 15.1). Three‐dimensional (3D) volume TEE and TTE scanning involves using a specialized matrix array probe to display a selected volume of the heart as a real‐time 3D data set [39]. Manipulating the data in real time can display anatomy from any perspective, including the surgical orientation [40, 41]. This latest advance replaces previously available 3D technology, which relied on off‐line reconstruction of sequentially acquired two‐dimensional (2D) image planes, thus limiting its use in the operating room. Unlike the almost “one‐size‐fits‐all” approach to selecting a TEE probe in an adult, choosing among available TEE devices adds another layer of complexity and sometimes even inconvenience to imaging pediatric patients (Figure 15.2). Two parameters guide the selection of an appropriate probe in the pediatric age group: patient weight and probe size (Table 15.4). At this time, it is rare to use monoplane and biplane TEE probes, as these may no longer be serviced by their respective manufacturers. However, several centers worldwide, mainly in countries with limited resources, still perform biplane and even monoplane TEE imaging in infants and children. The pediatric multiplane probe (mini‐multiplane) has allowed safe TEE imaging for many years in infants and small children weighing more than 3 kg. The availability of an even smaller device, a neonatal micro‐multiplane probe, allows the use of this imaging modality in tiny neonates weighing as little as 2.5 kg [42]. These TEE probes incorporate full 2D and Doppler capabilities [43]. In clinical practice, the use of both pediatric and neonatal TEE probes occurs in even smaller patients than recommended by the equipment manufacturers [44, 45]. In older children, the preference is to use multiplane imaging with an adult‐sized TEE probe, if feasible. Current 3D TEE probes have slightly larger dimensions than available adult 2D TEE probes, rendering them unsuitable for use in most young children. Although manufacturers suggest a patient weighing greater than 25 kg to use an adult 2D TEE probe or greater than 30 kg for a 3D TEE probe, in clinical practice, the usage of these devices often occurs in children weighing less than this. In general, these probes have more piezoelectric crystals than equivalent pediatric devices, and their larger size may improve contact with the esophagus; both features enhance the overall image resolution. Table 15.3 Currently available multiplane transesophageal echocardiography (TEE) probes for clinical use Abbreviations: H height; L length; W width Probe specification information provided by respective companies. Figure 15.1 Evolution of TEE imaging hardware. The graphic displays the evolution of TEE imaging technology from monoplane to biplane, to multiplane, to 3D devices. (Source: Texas Children’s Hospital. Reproduced with permission.) Figure 15.2 TEE multiplane probes. The photograph shows four currently available TEE multiplane imaging devices. (A) Neonatal probe; (B) pediatric probe; (C) “adult” 2D probe; (D) “adult” 3D probe. Table 15.4 Manufacturer recommendations for multiplane transesophageal echocardiography (TEE) probe selection according to patient weight 2D two‐dimensional; 3D three‐dimensional Intracardiac echocardiography (ICE) catheters (8–10 F) inserted via the transesophageal approach can provide 2D imaging in a single, longitudinal plane in neonates and small infants [46–48]. Although these catheters are not formally marketed for this specific application, reports indicate that they deliver clinically useful information in some cases, albeit with several limitations. In the operating room, TEE probe insertion occurs after induction of general anesthesia, endotracheal intubation, and arterial catheter placement. Although aspirating gastric contents is standard anesthetic practice in many centers, it does not reliably enhance TEE image quality [49]. In pediatric patients, some providers prefer nasal rather than oral endotracheal intubation to better stabilize the endotracheal tube (ETT), which reduces the risk of inadvertent extubation or movement during TEE probe manipulation. This practice, however, is variable. Regardless of the intubation route, securely taping the ETT should minimize the risk of displacement. Blind positioning of the lubricated probe tip in the oropharynx with gentle advancement into the esophagus should occur with the patient’s head in the midline position. The probe control for transducer tip flexion should remain unlocked at this time to allow free movement. Slight anterior flexion of the probe tip, forward thrusting of the mandible, and device guidance with a gloved finger can aid advancement of the probe along the contour of the posterior pharynx, thereby minimizing trauma. Alternatively, laryngoscopy can be used initially with direct visualization during probe insertion or after a difficult blind probe insertion [50]. In children, positioning the head to the side may facilitate the probe’s passage, as this position reportedly results in closure of the ipsilateral pyriform sinus, where the probe may encounter obstruction [51]. After placing the transducer behind the heart, repositioning the patient’s head avoids interference with the surgical field during probe manipulation. Independent factors that render probe insertion difficult in infants weighing 4 kg or less include lower weight, abnormal craniofacial anatomy, prematurity, a diagnosis of 22q11 deletion [52], and, possibly, Down syndrome. Probe placement is an essential skill in the practice of TEE. Distinguishing expected from excessive resistance requires thorough expertise and ongoing experience with the technique [53]. Although esophageal intubation requires some degree of effort, it is important never to force the probe against resistance. Manufacturers recommend insertion of the TEE probe through a bite block (also referred to as bite guard), which is positioned between the patient’s teeth to provide safe passage through the patient’s mouth, prevent damage to the TEE probe, and maintain the probe warranty. In clinical practice, this is more commonly used when imaging is done under sedation than with general anesthesia and more likely to be used in adults versus children. Probe insertion and manipulation can be significantly stimulating and requires an adequate level of patient sedation or anesthesia and even neuromuscular blockade in most children before placement. These maneuvers can also elicit clinically significant hemodynamic and/or respiratory changes, particularly in patients with specific anomalies with known significant potential risks of TEE (e.g., anomalous pulmonary venous drainage, vascular rings or slings); thus, vigilance is imperative [54]. In the event of acute hemodynamic or airway compromise, the probe should be repositioned or removed immediately. Outpatient TEE is performed often in adults but rarely in children, because the excellent image quality of TTE in the pediatric population provides adequate information as previously noted. In most cases, TEE is used to address a specific question, such as assessing for a thrombus in the LAA. A combination of oropharyngeal topical anesthesia and intravenous sedation may be sufficient for TEE imaging in the adolescent or adult. In younger patients, some adolescents, and a few adults, deep sedation or general anesthesia with endotracheal intubation may be necessary to overcome lack of cooperation, avoid respiratory compromise, and provide adequate time for a complete study. The facility where TEE occurs should be equipped with oxygen, suction capabilities, drugs, and equipment for cardiopulmonary resuscitation and other emergency therapies. Standard cardiorespiratory monitoring during the procedure includes intermittent blood pressure measurements, electrocardiography, pulse oximetry, and capnography. Patients with CHD may have significant hemodynamic alterations with marginal compensation, requiring the judicious, titrated administration of pharmacologic agents [55]. Patients with cyanotic heart disease are at additional risk for paradoxical air embolism during the administration of intravenous fluids or drugs. Decreases in systemic vascular resistance associated with a sedative or anesthetic agent can worsen right‐to‐left shunting and exacerbate underlying systemic arterial desaturation, necessitating careful observation of drug responses. Manipulating the TEE probe in several directions aids in imaging cardiovascular structures relative to the sagittal plane (Figure 15.3). The probe shaft can be pushed in (advanced) or pulled back (withdrawn) and can be turned to the right (clockwise) or to the left (counterclockwise). Wheel controls on the probe handle manipulate the probe tip, which can be flexed anteriorly (anteflexed) or posteriorly (retroflexed) and to the right or left (Figure 15.4). Pediatric TEE probes may lack the right‐to‐left flexion control, seldom a limitation, because only minimal adjustments in the probe’s position are needed to navigate from view to view. The imaging plane of a stationary, multiplane transducer can be rotated between angles of 0° and 180°, without the maneuvers required by monoplane and biplane devices to obtain equivalent views [56]. The control to change the multiplane angle in adult devices is electronic, whereas it can be either mechanical or electronic in pediatric devices (Figure 15.5). Figure 15.3 TEE probe manipulation. The graphic displays the terminology corresponding to the various TEE probe manipulation options. (A) Advance/withdraw; (B) turn to the right/left; (C) anteflex/retroflex; and (D) flex to the right/left. Note that the first two maneuvers (shown in panels A and B) require movement of the entire probe shaft, while performing the second two (depicted in panels C and D) is by special controls that move only the probe tip. (Source: Texas Children’s Hospital. Reproduced with permission.) Figure 15.4 Adult multiplane TEE probe controls. This photograph shows three different types of commercially available multiplane TEE adult probes with their respective flexion (anterior/posterior, right/left) and multiplane angle controls. The echocardiographic evaluation of the patient with CHD presents many challenges because of factors such as the wide spectrum of lesions; the myriad of anatomic variants, each with specific physiologic alterations; the frequent presence of coexistent pathologies; and the complexity of many defects [1]. Compounding these considerations is the need to be able to reconstruct the 3D anatomy of the congenitally malformed heart while relying primarily, and in most instances exclusively, on 2D imaging. Despite these challenges, TEE permits excellent visualization of cardiovascular structures and provides significant benefits. A comprehensive TEE examination in the patient with CHD can employ different approaches. Each approach has specific advantages and some potential disadvantages, as described briefly in the sections that follow. Figure 15.5 Pediatric multiplane TEE probes. The photograph displays two commercially available pediatric multiplane TEE probes to highlight differences in the multiplane angle control (mechanical in top panel vs. electronic in lower panel), the wheel control for flexion (anteflexion/retroflexion), and the probe tip locking mechanism. The “view‐based” TEE examination relies on obtaining several specific predefined cross sections by moving the probe to various locations within the esophagus or stomach. Initial guidelines by the ASE and Society of Cardiovascular Anesthesiologists (SCA) suggested a set of 20 TEE views for a comprehensive examination. This widely adopted approach became standard practice for the TEE examination of the adult patient with an anatomically normal heart [57]. Subsequently, updated recommendations incorporated 8 additional cross sections, for a total of 28 TEE views (Figure 15.6) [3]. Recent ASE TEE guidelines specifically addressing children and all patients with CHD recommend 28 views for a comprehensive examination [5]. Most of these views are the same described for imaging of the adult heart, but a few were added in recognition of the need for examination of specific structures or pathologies commonly seen in the pediatric age group or the congenital population (Figure 15.7). Selected adult TEE views were correspondingly excluded to maintain the same overall number of cross sections. A more detailed description of views useful in imaging CHD appears in the subsequent sections. During a complete TEE examination, obtaining most or all these views, regardless of sequence, provides comprehensive anatomic, functional, and hemodynamic information. This approach helps the practitioner learn the basic principles of TEE and represents an excellent foundation on the subject. The reader is referred to the outstanding web‐based educational resources of the Toronto General Hospital (www.pie.med.utoronto.ca/TEE/) for interactive modules on the standard adult TEE examination. Figure 15.6 TEE views in the comprehensive adult examination. This graphic displays the suggested 28 views in the adult guidelines for a comprehensive TEE examination [3]. The TEE probe positioned at the upper esophageal (UE), midesophageal (ME), transgastric (TG), and deep transgastric (DTG) levels can attain these cross sections. The grouping of the views in this graphic is by the structures being interrogated or the specific TEE window. The reader is referred to this publication for a detailed description of each of these views. A anterior; L left coronary cusp; LV left ventricle; MV mitral valve; N con coronary cusp; P posterior; R right coronary cusp. (Source: Ms. Willa Bradshaw. Reproduced with permission.) The view‐based TEE examination has substantial benefits, not only as a standardized method of performing the evaluation but also in terms of facilitating teaching, archiving, and reporting of the study, as well as quality assurance. Familiarity with these views, including the nomenclature, where and how to obtain each, the structures displayed by each, and the information derived, enhances one’s ability to perform a detailed examination and to communicate the findings to others. However, this approach has several limitations. The most recent pediatric and congenital TEE guidelines highlight two of them. First, the suggested TEE views were aimed primarily at the intraoperative interrogation and excluded views that may be necessary in nonoperative settings. Second, the outlined TEE examination was not diagnosis based, potentially excluding important views in the evaluation of specific cardiac conditions. These limitations are particularly relevant to the assessment of CHD, because a strict view‐based approach is inadequate for the detailed evaluation of structural abnormalities in many patients. Standard TEE views may not allow imaging of the complex anatomic relationships that characterize congenital pathology. Of critical relevance is that comprehensively evaluating many structural anomalies requires not only single views but also sweeps between multiple planes, some modified or nonstandard, aimed at building a 3D construct of the anatomic abnormalities. The “structure‐based” examination focuses on detailed TEE imaging of cardiovascular structures of interest by using complementary views to permit their in‐depth examination. Specific structural imaging in the adult has been described, for example, in the detailed evaluation of cardiac valves, the Ao, and the LAA, among other regions [3, 58–64]. A similar type of approach has been extremely helpful in characterizing specific anatomic and functional abnormalities in the patient with CHD, such as defects that involve the atrial or ventricular septum, pulmonary veins, and other structures [65–67]. This type of image‐acquisition paradigm may be preferable when, for various reasons, a focused TEE examination needs to address only a specific pathology of concern. One potential limitation of this approach in CHD is that by targeting only particular cardiac structures or pathologies, one may overlook relevant coexisting abnormalities or anatomic variants. However, by examining all relevant structures, this is the favored examination approach in CHD [5]. Figure 15.7 TEE views in the comprehensive pediatric and congenital TEE examination. The graphic displays the 28 recommended TEE views as a starting point in the guidelines addressing the comprehensive examination in children and all patients with CHD [5]. The grouping of the views in this figure is by the specific TEE window that the views can be obtained. The reader is referred to this publication for a detailed description of each of these views. (Sources: Texas Children’s Hospital. Reproduced with permission; panels marked by asterisk from Hahn et al. [3]. Reproduced with permission of Elsevier.) The “sequential segmental” analysis has been proposed as the foundation of complete anatomic assessment in CHD [68–71]. This approach involves a well‐organized, methodical interrogation of the three major cardiac segments (i.e., atria, ventricles, and great arteries) and their relationships to each other (connections or alignments between the segments), to define a patient’s anatomy and any abnormalities (Figure 15.8; also refer to Chapter 7). The incorporation of this type of analysis into many diagnostic imaging modalities in CHD is key in echocardiographic assessment (TTE and fetal imaging) [72–75]. The fundamental principle of this methodology is the identification of specific cardiac chambers by their characteristic morphologic properties, rather than by their positions within the body. The suggested practice examines the arrangement of the thoracoabdominal organs or situs, atrial structures, ventricles, and great arteries, as well as the connections between the segments, namely, the atrioventricular (AV) and ventriculoarterial (VA) connections [76, 77]. This approach has significant merit as congenital heart lesions can have normal or abnormal relationships among chambers, valves, and vessels (refer to Chapter 7). Figure 15.8 Sequential segmental analysis. The graphic illustrates the steps involved in the diagnostic analysis of CHD. The approach examines the following: cardiac situs; position of heart in the thorax and base to apex orientation; all segments and connections; and the cardiac defects. AV atrioventricular; IVC inferior vena cava; IVS interventricular septum; L left; LA left atrium; LAA left atrial appendage; LPA left pulmonary artery; LV left ventricle; MV mitral valve; PA pulmonary artery; R right; RA right atrium; RAA right atrial appendage; RPA right pulmonary artery; RV right ventricle; SVC superior vena cava; TV tricuspid valve. (Source: Figure courtesy of Ms. Willa Bradshaw.) The sequential segmental analysis overcomes the limitations of a purely structure‐based approach, as careful interrogation and identification of all cardiovascular structures ensures a thorough TEE assessment. Despite these advantages, for those unfamiliar with segmental anatomy, the methodology involved may be less intuitive and more time‐consuming. However, this approach is very useful, particularly in a preoperative study performed to characterize or confirm the anatomic abnormalities. Guidelines and recommendations exist for the application of TTE and TEE imaging in CHD [1, 3, 5–8]. Previous publications have addressed applications of TEE in CHD [10, 78], with the most recent guidelines outlining in detail the comprehensive and systematic examination in these patients [5]. Providing details of the TEE examination in CHD is beyond the scope of this chapter, so we review only selected aspects, and the reader should look to other publications for an exhaustive discussion on the subject [5, 28, 79, 80]. Although there is no predetermined sequence for the order of acquisition of information during a TEE examination in CHD, and one should not be inferred from the following outline, it is valuable for individuals to develop their own organized approach to performing a complete and expeditious interrogation. Unique patient conditions or specific circumstances, such as hemodynamic instability or respiratory impairment by TEE probe manipulation, may shorten the examination. The sections that follow on imaging windows and planes provide a brief overview of selected TEE views frequently obtained during CHD evaluation. The examination assumes levocardia (heart in the left thoracic cavity with apex pointing to the left) and normal segmental anatomy: in other words, visceroatrial situs solitus (stomach on the left, liver on the right, and normal atrial arrangement), concordant AV and VA connections, normal ventricular topology, and great artery spatial orientation. It may not be possible to obtain all views in all patients, nor will the planes and angles of interrogation necessarily conform to each patient’s unique anatomy. The variety of structural cardiovascular malformations dictates a modified scheme in many cases. The comprehensive TEE examination in CHD includes detailed 2D imaging and meticulous spectral and color‐flow Doppler interrogation. Contrast echocardiography using an injection of agitated saline or 5% albumin into a peripheral or central vein can detect small intracardiac shunts, further delineate anatomy (e.g., persistent left superior vena cava [SVC]–to–coronary sinus connection), and identify pathology such as atrial baffle leaks [81, 82]. Figure 15.9 TEE imaging windows. The standard TEE imaging windows and representative imaging planes are shown. DTG deep transgastric; ME midesophageal; TG transgastric; UE upper esophageal positions. (Source: Texas Children’s Hospital. Reproduced with permission.) During a TEE examination in CHD, imaging of the heart and related vascular structures occurs at multiple levels: upper esophageal (UE), midesophageal (ME), TG, and DTG (Figure 15.9). Different transducer angles are used to obtain specific cross sections. These diverse TEE windows complement each other and provide information that is not otherwise obtainable in some views. The TG and DTG views, for example, provide favorable alignment of the Doppler angle of interrogation to the outflow tracts, optimizing spectral Doppler signals and the assessment of gradients. The guidelines for performing a comprehensive TEE examination in children and all patients with CHD describe in detail the recommended 28 tomographic views, acquisition protocol, and structures imaged in each of these cross sections (Figure 15.7). The reader is referred to this document for further information [5]. Table 15.5 highlights how these multiplane TEE views can be obtained (note that this is a succinct guide, as there are several approaches), structures displayed, and relevant features of each. As emphasized in the guidelines document, these views are only a starting point, as additional, modified, nonstandard cross sections may be necessary to define the anatomy of a given patient. This is particularly important in patients with complex anatomy, such as seen in heterotaxy, and in patients with cardiac malpositions, for whom the multiplane transducer angles for the standard views may need to be significantly altered. Table 15.5 Transesophageal echocardiography (TEE) views for a comprehensive examination in children and all patients with congenital heart disease Abbreviations: Ao aorta; AV atrioventricular; Asc ascending; CS coronary sinus; Desc descending; IAS interatrial septum; IVC inferior vena cava; IVS interventricular septum; LA left atrium; LAA left atrial appendage; LPA left pulmonary artery; LV left ventricle; LVOT left ventricular outflow tract; MPA main pulmonary artery; MV mitral valve; PA pulmonary artery; PV pulmonary valve; Prox proximal; RA right atrium; RAA right atrial appendage; RPA right pulmonary artery; RV right ventricle; RVOT right ventricular outflow tract; SVC superior vena cava; TR tricuspid regurgitation; TTE transthoracic echocardiography; TV tricuspid valve; VSD ventricular septal defect In general, the views can be considered as follows: A significant amount of TEE imaging takes place in the ME region; in fact, this window allows acquisition of more than half of the suggested views (15/28) for a comprehensive pediatric or adult CHD examination. The ME four‐chamber view (ME 4‐Ch) is considered the starting view or “home base,” as it acts as a reference point for obtaining other views by manipulating the probe (tip or shaft adjustments) or adjusting the transducer angle on a multiplane probe. The TG views include five cross sections of the heart. Transverse plane imaging shows the ventricles in short axis at various levels from base to apex. Longitudinal plane imaging shows the left ventricle (LV) and right ventricle (RV) and the inferior cavoatrial region. Advancing the probe into the fundus of the stomach obtains the three DTG views. Up‐down inversion displays the TEE images in an anatomic orientation (base of the heart at the top of the sector and apex at the bottom), which assists tremendously in the understanding of complex disease and correlates to TTE imaging and other diagnostic modalities. This convention is not applied to standard adult TEE DTG imaging, or to equivalent apical or subcostal views obtained by surface imaging in the adult patient. The DTG views of the semilunar valves or ventricular outflow tracts provide excellent angles of spectral Doppler interrogation, thereby allowing optimal alignment of the cursor parallel to the direction of blood flow. These views also significantly contribute to the evaluation of VA connections in CHD. These three views allow imaging of vascular structures (e.g., pulmonary artery [PA], aortic arch, variants or anomalies). The views of the thoracic descending Ao can be obtained at various levels by withdrawing and advancing the imaging probe. The level of the Ao in these views can be difficult to interpret because of the lack of landmarks. Ease of acquisition with rapid online display of detailed dynamic images has increased the use of real‐time 3D TEE in the perioperative period to assess cardiac anatomy and function [4, 40, 41, 83–85]. Special matrix array ultrasonography probes acquire raw data by volume scanning, and integrated ultrasonography machine software processes the 3D data sets. These TEE probes perform all standard 2D functions, include motion mode (M‐mode) and all Doppler modalities (e.g., spectral, color, tissue), and have the additional feature of multiplane mode (X‐plane) to simultaneously display independent 2D scanning planes during the same heartbeat. Single‐button activation of specific 3D imaging modes for both TEE and TTE matrix array probes include live, zoom, full volume, and color Doppler full volume, allowing for real‐time imaging of cardiac structures (Table 15.6). Using these modes, one can define a specific region of interest to obtain a 3D volume of varying sizes. Newer software can display all 3D data sets in real time, such that moving the probe will immediately affect the 3D volume on the display. Currently, all real‐time 3D imaging modes appear as a volume‐rendered 3D object with full details of the surface and inner structure. The free rotation of any rendered 3D object on the screen allows its display from any orientation, including the surgeon’s perspective. Cropping permits a “virtual dissection” of the 3D object that examines all its details. The 3D technology has proven superior to 2D echocardiography for assessing the LV (volume, ejection fraction [EF], mass, and dyssynchrony) and the mitral valve (MV) [86]. En face views of the MV, for example, when displayed in the surgeon’s orientation viewed from the left atrium (LA), show the anatomical details of the leaflets and define leaflet pathology of prolapse, clefts, and perforations [87, 88]. Thus, the use of this modality has become well accepted for adult patients undergoing cardiothoracic surgical interventions and catheter‐based procedures. The excellent detail of 3D imaging provides more information about cardiac anatomy and spatial relationships than 2D echocardiography, clearly a merit of the technology in evaluating CHD. Real‐time 3D TEE can better show the intricate relations of cardiac structures in patients with complex CHD. While multiple 2D views were previously necessary to assess pathology, 3D imaging can show the entire heart in a single screen display. In assessing CHD, 3D TEE has a major role in evaluating defects in both the atrial and ventricular septum and valvular pathologies of the MV and aortic valve (AoV) [4, 89]. Current recommendations for 3D TEE imaging in CHD appear in Table 15.7 [5]. Although experience with real‐time 3D TEE in children with CHD is limited by the lack of suitable imaging devices, applications of the technology have shown extremely favorable results [4, 21, 65, 66, 89–95], mirroring the reported 3D TTE experience in CHD [96, 97]. It is reasonable to consider this modality in cases for which a 3D perspective may offer additional benefits over routine 2D TEE in the patient suitable for 3D TEE imaging with currently available devices. Similar to 2D TEE, structures closest to the probe are easily imaged in 3D, enabling the evaluation of clinically relevant issues in real time. The thin interatrial septal (IAS) and distant structures (AoV and pulmonary valve [PV]) remain difficult to image by 3D TEE in some patients. Despite recommendations for 3D echocardiographic image acquisition and display, a consensus statement on 3D imaging in CHD, and the reported clinical use of 3D TEE in the pediatric and CHD TEE guidelines, there remains no standardized protocol for the use of intraoperative real‐time 3D TEE in everyday practice [4, 5, 83]. Although 3D image acquisition is rapid, processing the 3D data sets with analytical software can be time‐consuming and challenging in the hectic intraoperative environment. Table 15.6 Real‐time three‐dimensional transesophageal echocardiography imaging The standard 2D transesophageal echocardiography (TEE) view is used with different 3D imaging modes to scan a region of interest and obtain a volume of tissue. This volume or block of tissue can be cropped, rotated, and displayed in any orientation to show normal and pathologic findings. Abbreviations: 2D two‐dimensional; 3D three‐dimensional; AoV aortic valve; ASD atrial septal defect; Bic bicaval; CD color Doppler; Comm commissural; FV full volume; LAA left atrial appendage; LAX long‐axis; ME midesophageal; MV mitral valve; SAX short‐axis; TAVI transcatheter AoV implantation; TG transgastric; UE Ao Arch upper esophageal aortic arch; 2‐Ch two‐chamber; 4‐Ch four‐chamber Table 15.7 Clinical use of three‐dimensional (3D) transesophageal echocardiography (TEE) in congenital heart disease Source: Reproduced from Puchalski et al. [5], with permission Elsevier. Abbreviations: ASD atrial septal defect; VSD ventricular septal defect Assessment of ventricular function is an important component of a comprehensive TEE examination. While it is common to evaluate systolic function, evaluating diastolic function is an area of growing interest because there is increasing recognition of the role that diastolic dysfunction plays in clinical outcomes [98]. The ventricles differ in geometry, systolic contraction pattern, and load conditions, requiring different methods to assess each chamber. Quantitative assessment of LV systolic function by echocardiography relies on geometric models that may not be applicable to the unusual ventricular geometries typical of CHD [2, 99]. In patients with CHD, the extents of RV enlargement and deterioration of function often determine the need for clinical intervention [100]. Currently, CMR is the gold standard for assessing RV systolic function [101], whereas real‐time 3D echocardiography is comparable to CMR in assessing LV function [83]. Newer echocardiographic techniques can quantify subtle changes in regional and global ventricular function, independent of ventricular morphology, and are less affected by loading conditions [102]. Tissue Doppler imaging (TDI), also referred to as Doppler tissue imaging, provides a reproducible, clinically valid, and easily obtainable nongeometric quantitative method to assess both systolic and diastolic ventricular function [103]. Strain and strain rate imaging can provide global and regional assessment of ventricular function that is independent of geometry and less affected by loading conditions [104–106]. Improved ultrasonography technology has simplified techniques such as TDI, 3D EF, and tissue tracking for strain and strain rate to better quantify systolic function in the operative setting [107, 108]. For many of these techniques, there are reference values for normal adults and children, most often established by TTE [2, 99, 109, 110]. These values vary greatly according to age and lack validation by TEE. The discussion that follows highlights general concepts regarding selected approaches for assessing ventricular function by echocardiography. Normal LV function involves complex 3D movements during the cardiac cycle. Systolic function depends on heart rate, myocardial contractility, and loading conditions. Typically, quantitative echocardiographic assessment of LV function measures changes in LV volume by calculating stroke volume (SV), LV ejection fraction (LVEF), and cardiac output (CO). However, these quantitative measures are time‐consuming, limited by loading conditions, and affected by alterations in geometric shape. Technical concerns when using TEE, such as chamber foreshortening and poor endocardial definition, further complicate the acquisition of quantitative information. Thus, intraoperative ventricular systolic performance is often assessed qualitatively, most commonly by “eyeballing” and at times by using methods such as simple linear (shortening fraction [SF]) and area (fractional area change [FAC]) measurements. “Eyeballing” of wall motion and thickening in the ME 4‐Ch, TG Mid Pap SAX, and TG LAX views (refer to Figure 15.7 and Table 15.5) can be used to qualitatively assess ventricular systolic function. Although used ubiquitously, this subjective technique lacks standardization, depends on experience, and has significant inter‐ and intra‐observer variability. The TG Mid Pap SAX view is often used but examines only a portion of the LV (six segments) and may not reflect global systolic function in hearts with an abnormal geometric shape or regional wall motion abnormalities. SF estimates LV systolic function from linear measures of end‐diastolic diameter (LVEDD) and end‐systolic diameter (LVESD) by using M‐mode in a TG Mid Pap SAX view of the LV (Figure 15.10A). The superior temporal resolution of M‐mode echocardiography can capture the movement of structures over time. The SF as a percentage (normal: 26–45%) is determined by the following formula:
CHAPTER 15
Transesophageal and Epicardial Echocardiography in Congenital Heart Disease
Introduction
Indications for TEE in CHD
Diagnostic indications
Perioperative indications
TEE‐guided interventions
Contraindications to TEE
Absolute
Relative
TEE hardware
Echocardiographic system
TEE imaging probes
Device selection
TEE device
Tip dimensions (W × H × L, in mm)
Shaft dimensions (W × L, mm × cm)
Number of elements
Imaging frequencies (MHz)
Philips
Neonatal or micro‐multiplane (S8‐3t)
7.5 × 5.5 × 18.5
5.2 × 88
32
3.0–8.0
Pediatric or mini‐multiplane (S7‐3t)
10.7 × 8.0 × 27
7.4 × 70
48
3.0–7.0
Adult 2D multiplane (S7‐2t)
15.0 × 12.5 × 35
10.0 × 90
64
2.0–7.0
Adult 3D (X8‐2t)
17.0 × 13.4 × 38
10.0 × 100
2,500 matrix
2.0–8.0
Siemens
Pediatric multiplane (V7M)
10.7 × 8 × 36*
7.0 × 70
48
4.0–8.0
Adult multiplane (V5M)
14.5 × 11.5 × 45
10.5 × 110
64
3.5–7.0
Adult 3D/4D (Z6Ms)
15.0 × 12.0 × 40
10.2 × 100
2,304 matrix
General Electric
Neonatal or micro‐multiplane (10T‐D)
7.6 × 5.6 × 16
5.6 × 90
32
3.3–10.0
Pediatric micro‐multiplane (9T, 9Tc, 9T‐RS)
10.9 × 8.4 × 35.2
7.0 × 70
48
3.0–10
Adult multiplane (6T, 6Tc, 6Tc‐RS))
14.0 × 12.5 × 45
10.5 × 110
64
3.0–8.0
Adult 3D/4D (6VT‐D)
14.3 × 12.7 × 44.8
10.5 × 110
2,500 matrix
3.0–8.0
TEE multiplane probe
Minimum patient weight (kg)
Neonatal
2.5
Pediatric
3.5
Adult (2D)
25
Adult (3D)
30
Probe insertion
Instrument manipulation and probe controls
TEE examination in CHD
Structural examination
View‐based approach
Structure‐based approach
Sequential segmental approach
The TEE examination
Imaging windows
Multiplane imaging views
View (abbreviation)
Acquisition details
Structures imaged and assessment
Midesophageal views
ME Four‐chamber view
(ME 4‐Ch)
Neutral probe position, transducer angle of ~0°–10°
Equivalent to TTE apical 4‐Ch view
All four cardiac chambers, AV valves, atrial and ventricular septa, CS
ME Five‐chamber view
(ME 5‐Ch)
From ME 4‐Ch view probe tip anteflexion or probe withdrawal
In addition to structures in the ME 4‐Ch view, LVOT, proximal Ao
ME Mitral valve view
(ME Mitral)
From ME 4‐Ch view increase transducer angle to ~50°–70°
LA, LV, MV (segments, commissures), tensor apparatus, papillary muscles, CS
ME Two‐chamber view
(ME 2‐Ch)
From ME 4‐Ch view increase transducer angle ~80°–100°
Orthogonal to the ME 4‐Ch view
LA, LAA, MV, LV, CS
ME Long‐axis
(ME LAX)
From ME 4‐Ch view increase transducer angle to ~120°–140°
LA, LV, RVOT, LVOT, MV, AoV, prox Asc Ao aorta
ME Aortic valve long‐axis
(ME AoV LAX)
From ME LAX view reduce image depth
Focused view of LVOT, AoV, prox Asc Ao
ME Aortic valve short‐axis
(ME AoV SAX)
From ME 5‐Ch view keep Ao root in focus, withdraw probe, adjust transducer plane to 25°–45°
Orthogonal to ME AoV LAX view
AoV morphology (number of cusps, commissures), function, localization of pathology (stenosis, regurgitation)
ME Ascending aorta long‐axis
(ME Asc Ao LAX)
From ME LAX view withdraw probe, adjust transducer angle to ~90°–110°
Asc Ao in long axis, RPA
Allows for assessment of proximal to mid ascending aortic pathology
ME Ascending aorta short‐axis
(ME Asc Ao SAX)
From ME Asc Ao LAX view decrease transducer angle to ~0°–30°
Orthogonal to ME Asc Ao LAX view
Prox Asc Ao (short axis), MPA (long axis), RPA, SVC
Allows for Doppler assessment of MPA
ME Right ventricular inflow‐outflow
(ME RV In‐Out)
From ME 4‐Ch, ME 5‐Ch, or ME AoV SAX anteflex probe tip, rotate transducer angle to ~50°–70°
Mimics inverted TTE parasternal SAX view at base of heart
RA, LA, IAS, TV, PV, AoV, MPA, inflow and outflow of RV
Useful to assess VSDs, TR jets, RV size, function, and obstruction
ME Bicaval view
(ME Bicaval)
Transducer angle of 90°–110°
Orthogonal to ME 4‐Ch view
SVC and IVC entry to RA, RAA, IAS; useful to assess IAS, central catheter tip position
ME Modified Bicaval tricuspid valve
(ME Mod Bicaval TV)
Transducer angle of 50°–70° or from ME Bicaval view turn probe to the right
TV (anatomy, regurgitation severity, regurgitant Doppler peak velocity), LA, RA
ME Left atrial appendage view
(ME LAA)
Transducer angle of ~90°–110°
LAA also seen in ME 4‐Ch view with LA centered and probe shaft turned to left
LAA, variable anatomy of LAA requires multiple views for a detailed examination
ME Right pulmonary veins
(ME Rt Pulm veins)
From ME 4‐Ch view, turn probe shaft to right at transducer angle 0°, adjusting to 30°–50° may display all right‐sided pulmonary veins
Alternate approach is to turn the probe to the right from the ME Bicaval view
Right pulmonary veins, SVC, Mid Asc Ao
ME Left pulmonary veins
(ME Lf Pulm veins)
After centering LA in screen rotate transducer angle to 90°–110° and turn probe shaft to the left
Alternate approach is from the ME 4‐Ch view to turn probe to the left to image the LAA, and slowly advance–withdraw the probe
Left pulmonary veins, LPA
Transgastric views
TG Basal short‐axis
(TG Basal SAX)
TG Mid‐papillary short‐axis
(TG Mid Pap SAX)
TG Apical short‐axis
(TG Apical SAX)
Gently advance TEE probe into the lower esophagus/stomach, with slight anteflexion at transducer angle of ~0°–20°
Multiple cross‐sectional short‐axis views of LV at basal, mid‐papillary, and apical levels
TG Mid Pap SAX view extremely helpful in evaluating LV function (global and segmental), LV preload
TG Long‐axis
(TG LAX)
From TG Mid Pap view increase transducer angle to ~120°–140°
LV (long axis), LVOT, AoV
Useful for Doppler evaluation of Ao outflow
TG Inferior vena cava/Hepatic veins
(TG IVC/Hep veins)
From ME Bicaval view advance probe into the distal esophagus or upper gastric region at transducer angle of ~80°–100°
IVC, hepatic veins, RA
Useful to assess situs and evaluate flow in venoatrial connection (IVC–RA), hepatic veins, anomalous pulmonary veins
Deep transgastric views
DTG Five‐chamber view
(DTG 5‐Ch)
Advance probe further into stomach with transducer angle of 0°–20° and variable degrees of tip anteflexion
LV is displayed from base at top to apex at bottom of image (in contrast to standard adult TEE view)
Nearly equivalent to ME 5‐Ch view
Ventricles, LVOT, Ao root, IVS, MV
DTG Right ventricular outflow tract
(TG RVOT)
Not a standard view in the adult TEE guidelines
From DTG 5‐Ch view rotate transducer angle to ~50°–90°
At times, rightward probe shaft rotation can be helpful
Ventricles, RVOT, MPA, IVS, MV
DTG Atrial septal
(TG Atr Sept)
Not a standard view in the adult TEE guidelines
From the DTG RVOT view turn probe shaft right with a transducer angle to ~80°–90°
Simulates TTE subcostal bicaval view
Excellent delineation of IAS and systemic venous connections
Visualizing the IVC may require a transducer angle of ~100°–120°)
Upper esophageal views
UE Pulmonary artery view
(UE PA)
Not a standard view in the adult TEE guidelines
From ME 4‐Ch view withdraw TEE probe, anteflex tip, turn shaft left at a transducer angle of ~0°–20°
Alternatively, from ME RV In‐Out view withdraw probe and adjust transducer angle
MPA and branches
Useful to assess main PA, bifurcation, proximal branches, and to perform Doppler exam
Interposition of left mainstem bronchus, makes imaging LPA difficult
UE Aortic arch long‐axis
(UE Ao Arch LAX)
Position probe in upper esophagus with transducer angle of ~0°–10°
Aortic arch (long axis), innominate vein
UE Aortic arch short‐axis
(UE Ao Arch SAX)
From UE Ao Arch LAX increase transducer angle to ~70°–90°
Orthogonal to UE Ao Arch LAX
Aortic arch (short axis), innominate vein, PV, MPA and branch PAs (with probe shaft rotation)
Aorta views
Descending aorta in short‐axis
(Desc Ao SAX)
From ME 4‐Ch view rotate probe shaft left to display the left‐sided pulmonary veins and LAA, turning probe further posteriorly shows the Desc Ao SAX at a transducer angle ~0°–10°
Desc Ao (short axis)
Descending aorta in long‐axis
(Desc Ao LAX)
From desc Ao SAX view increase transducer angle to ~90°–100° (orthogonal to Desc Ao SAX view)
Desc Ao (long axis)
ME views
TG views
DTG views
UE views
Aortic views
Three‐dimensional TEE
Structure
2D view for 3D acquisition
3D mode
Clinical utility
Mitral valve
ME 4‐Ch (0°), ME MV Comm (60°), ME 2‐Ch (90°), ME LAX (120°); ± CD for all views
Zoom, FV
Morphology, function, quantification (effective regurgitant orifice area, MV area)
Aortic valve
ME AoV SAX (30°–60°), ME AoV LAX (120°); ± CD for all views
Zoom
AoV area, TAVI
Tricuspid valve
ME 4‐Ch (0°–30°), TG (40°); ± CD for all views
Zoom
Unstudied
Pulmonary valve
UE Ao Arch SAX (90°) ± CD
Zoom
TEE has limited views
Interatrial septum
ME 4‐Ch (0°), ME Bic (90°); ± CD for all views
Zoom, FV
ASD
Left ventricle
ME 4‐Ch (0°), ME 2‐Ch (90°), ME LAX (120°)
FV
Volume, mass, ejection fraction, dyssynchrony
Right ventricle
ME 4‐Ch (0°), ME 2‐Ch (90°), ME LAX (120°)
FV
Volume, ejection fraction
Left atrial appendage
ME 4‐Ch (0°), ME MV Comm (60°), ME 2‐Ch (90°), ME LAA (110°)
Live, Zoom
Thrombus
3D TEE has been recommended for
3D TEE has been used effectively during
Functional assessment
LV systolic function

Full access? Get Clinical Tree
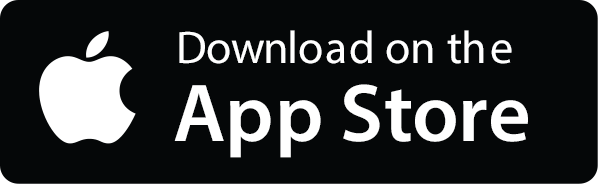
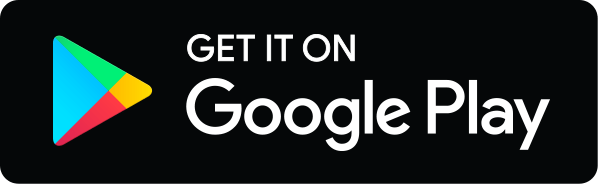