A solitary pulmonary nodule (SPN) is a round or oval opacity smaller than 3 cm in diameter that is completely surrounded by pulmonary parenchyma. Owing to the high etiological diversity and the potential for malignancy, pulmonary nodules represent a clinical challenge, which becomes increasingly frequent as the number of CT examinations rises. Due to its low sensitivity, a bronchoscopic approach to small pulmonary nodules <2 cm in diameter was previously not recommended by The American College of Chest Physicians. With the recent advent of innovative guidance technics for bronchoscopic sampling, this statement was revised, taking the higher diagnostic yield of navigation systems such as endobronchial ultrasound, guide sheath, virtual bronchoscopy, and electromagnetic navigation into account. By means of these methods, the identification of malignant nodules measuring ≤≤2 cm could be raised from a prior reported overall sensitivity of 33 percent for fluoroscopy-guided bronchoscopy with transbronchial biopsy (TBB) to a pooled diagnostic yield of 61 percent.
The following chapter will give an overview of the different earlier mentioned navigation technics.
Endobronchial Ultrasound for Peripheral Lesions
In the diagnosis of peripheral lung lesions, radial probe endobronchial ultrasound (EBUS) provides real-time confirmation of the target lesion localization. This method currently depicts the most practically used bronchoscopic device in the diagnosis of peripheral lung lesions. Consisting of a rotating piezoelectric crystal embedded in a flexible catheter, the miniaturized transducer, also referred to as miniprobe, produces a 360° ultrasound image. The most commonly used frequency of the EBUS probe is 20 MHz which allowes for a high resolution of <1 mm and a penetration depth of around 4 cm. Aside from the excellent depiction of the airway wall with its different layers, it enables the investigation of peribronchial structures not only within the mediastinum, but also in the lung periphery. For the purpose of diagnosing peripheral lung lesions and pulmonary nodules, the miniprobe is advanced into different bronchi like a forceps. Unlike the forceps, the EBUS probe must be handled carefully due to its fragility. Based on the pre-procedure CT scan, the miniprobe is inserted into the segmental bronchi where the lesion is suspected. In case the operator perceives a high resistance while introducing the probe deeper into the desired bronchus, one can dilate the airway through prior insertion of a sampling forceps. By this means damage to the transducer or the connecting drive wire by shear forces and flexion can be prevented.
While advancing the miniprobe through the airway, a characteristic “snowstormlike” whitish ultrasound image (Figure 15.1), produced by air-filled alveolar tissue, can be seen. Due to the total reflection of ultrasound waves by air, structures that are close to the probe but separated by air cannot be inspected. Particularly in the proximal airways, vessels, appearing totally black, are often noticed. Owing to the lack of color doppler imaging in radial EBUS imaging, it is sometimes difficult to clearly differentiate between vessels and necrotic areas. By tracking the structure in its entirety and looking for potential arterial pulsation it is usually possible to make a clear differentiation. Once a solid tumor is reached, the ultrasound image will change to a homogeneous grey ultrasound image with plain white borders indicating the borders of the lesion (Figure 15.2). Continuous hyper echoic margins and the absence of an air bronchogram further raise the suspicion for cancer. Dark inhomogeneous zones however, do not exclude malignancy since necrotic areas and vessels within the tumor might be visible. Atelectasis or inflammatory tissue is characterized by an inhomogeneous image resulting from different structures like fluid filled dark structures and sharp white echoes from small bronchi without plain borders (Figure 15.3).
Figure 15.1 Typical EBUS image of normal peripheral lung.
Figure 15.2 EBUS image of a solitary pulmonary nodule.
Figure 15.3 EBUS image of an or inflammatory process (inhomogeneous image, resulting from different structures like fluid filled dark structures and sharp white echoes)
Once having localized the target lesion, a methodological limitation of the EBUS-TBB approach constitutes the removal of the ultrasonic probe from the bronchoscope prior to the introduction of biopsy instruments. The procedure is not “real-time”; hence, the detection of the identic subsegmental bronchus after removal of the miniprobe can be problematic. This is often aggravated by the different rigidity of the bronchoscope after insertion of the biopsy forceps into the working channel. Furthermore, due to its higher rigidity, the biopsy forceps sometimes takes another path in the distal bronchial distribution as the miniprobe.
To overcome this constraint, the operator has mainly two options. First introduced by Kurimoto, the endobronchial ultrasound can be used in combination with a guide sheath. For the 1.4-mm measuring miniprobes, catheters with a diameter of 2.0 mm can be used, whereas the larger 1.7-mm probes should be employed with catheters of a 2.7-mm diameter. The probe is advanced through the guide sheath which serves as an extended working channel. Once the lesion is reached and confirmed via EBUS, the miniprobe is extracted while the guide sheath is kept in place. Now different biopsy instruments can be advanced through the guide sheath which enables sampling at the exact same position as the miniprobe before. If the target bronchus cannot be intubated due to the required angle, a double-hinged curette can be used in order to lead the guide sheath in successfully reaching the lesion. Before usage, the miniprobe has to be removed from the guide sheath in order to allow the double-hinged curette to be inserted. Once the desired bronchus is reached under fluoroscopic guidance, the miniprobe can be reinstalled.
Another EBUS guiding option is the fluoroscopic registration of the miniprobe position once the target lesion is reached. By means of intermittent fluoroscopic guidance, the biopsy tool can be advanced to the identical location as the prior miniprobe. A potential benefit of this approach is the shorter duration and retrenchment at expense of radiation burden. Since fluoroscopic and EBUS guidance are frequently combined in order to reach the target lesion, the fluoroscopic registration of the miniprobe seldom depicts an extra effort.
Particularly in case of small pulmonary nodules, which can seldom be visualized by fluoroscopy, EBUS guidance offers an advantage. A prospective study investigating the diagnostic yield of EBUS in fluoroscopically invisible lung lesions could establish a diagnosis by biopsy in 70 percent of the cases. In pulmonary nodules <2 cm, a diagnostic yield of 69 percent was reported if the lesion could be visualized by EBUS. Another study could show the usefulness of EBUS guidance in combination with guide sheath as a stand-alone guidance technic. A systematic review of published literature evaluating radial probe EBUS accuracy showed a sensitivity (73 percent) and specificity (100 percent) markedly higher than for routine bronchoscopy. However a significant inter-study variation in EBUS method was noted.
Virtual Bronchoscopic Navigation / Ultrathin Bronchoscopy
Virtual Bronchoscopy (VB) is a relative novel technique for the noninvasive evaluation of the tracheobronchial tree. Through reconstruction of helical CT images it creates a three-dimensional rendering resembling the bronchoscopical investigation of the lung. Except radiation exposure, VB has no adverse effects but requires thin-section CT scans with a slice thickness of 1.25 mm or less, depending on the system used. The level of anatomic detail visualized by VB depends on the obtained volume data. In general, good volume data are obtained by minimizing collimation and overlapping the image reconstruction by at least 50 percent.
Until recently VB has been used mainly for the investigation of trachea and bronchi in children, evaluation of airway stenosis, endobronchial malignancy, postoperative bronchial complications, and the study of anatomic malformation and variants.
Prior to a bronchoscopical investigation of a pulmonary nodule, the bronchoscopist aims to determine the bronchial path leading to the desired target based on CT images. However, it can be difficult to obtain a three-dimensional understanding of the complicated tracheobronchial tree solely relying on axial images. By providing a three-dimensional depiction of the bronchial path to the lesion, VB resolves this problem. Particularly for the usage of ultrathin bronchoscopes with external diameters of 3 cm, this might be a key advantage. Whereas standard bronchoscopes are limited to the first three to four divisions of the bronchial tree, ultrathin bronchoscopes can be advanced to a median of the sixth generation bronchi. Here VBN comes into play since guidance in the deeper bronchial tree gets more difficult with every branching. In case of successful guidance, subsequent sampling by forceps is easier due to the closer advance of the ultrathin bronchoscope to the pulmonary nodule. In addition, the ultrathin bronchoscope can be advanced to lesions in places such as the mediastinal side of the lung apex that are difficult to reach with a conventional bronchoscope.
Current VBN guidance systems include, but are not limited to the LungPoint System by Broncus Technologies and the VBN system Bf-NAVI by Olympus Medical Systems. Those Systems process recorded CT data in order to automatically compile three-dimensional VB images. Once the operator chooses the target, up to three pathways are calculated to reach this point closest as possible. A virtual bronchoscopic animation enables the estimation of the calculated pathways prior to the intervention. During the intervention, VB images are synchronized with real images for bronchoscopic navigation (Figure 15.4). Since the tip of the bronchoscope can only be steered in one axis, variable rotation of the scope is necessary at every bronchus branching. This causes a shift of the virtual and real image to a greater or lesser extent. To overcome this issue, an assistant adjusts the virtual images produced by the Bf-NAVI system by using the forward, backward, and rotation function. As the bronchial branching pattern includes many bifurcations into two bronchi of similar sizes, mistakes tend to be made identifying the bronchus into which the bronchoscope should be advanced. Therefore, careful alignment of the virtual and real image is necessary prior to further bronchoscope advancement. After reconciliation, VB images of the next branching indicate the proper path for the subsequent bronchoscope advancement. This procedure is repeated until the target bronchus is reached. As a result of unexpected patient movements such as coughing, a substantial image shift might emerge. In this case, VB images can be redisplayed and thumbnails of each branching provide a reference.
Figure 15.4 Virtual track of the LungPoint system in the CT navigation image (left) and in the real time image (right)
The LungPoint system offers a slightly different solution for the earlier-discussed issues of image shifting and unexpected patient movement. The system displays VB image and the bronchoscopic video next to each other and overlays the correct path to the target airway on both images in blue. An image-based synchronization technique aligns virtual image and bronchus anatomy seen through the bronchoscope. This process can be accelerated or corrected by an assistant by advancement and adjustment of the virtual bronchoscopic rendering. The system not only displays airways measuring 3 mm and greater, but also extracts major vessels. Those and the target can be overlaid on the VB image to further facilitate navigation and increase procedure safety.
One study evaluating the LungPoint system showed a mean planning time of five minutes and a mean examination time of 15 minutes. In general, VBN seems to reduce the bronchoscopic examination time by facilitating its navigation. This holds true especially for procedures applying an ultrathin bronchoscope.
VBN studies available to date show a high diagnostic yield of 73 percent by sampling 428 lesions with a slightly lower yield of 67 percent for lesions <2 cm. However, concerning the usefulness of combining ultrathin bronchoscopes and VBN, conflicting evidence exists. This might be owed to the possibility that VBN is more useful for non-expert bronchoscopists, since highly trained operators might be able to assume the route to the desired target to some extent from CT images alone.
One limitation of ultrathin bronchoscopes depicts the restriction to miniaturized sampling tools and therefore the extraction of merely small tissue samples. In future, this might be overcome by small cryoprobes designed for tissue sampling through ultrathin bronchoscopes.
Electromagnetic Navigation
In contrast to VBN, electromagnetic navigation bronchoscopy (ENB) depicts a real-time procedure with the ability of mapping instruments within an electromagnetic field. It resembles a GPS car system whereby the operator knows the position of the instruments at any point of the procedure. Examples for ENB are the Superdimension and the Veran guidance system. ENB not only provides guiding to peripheral lung lesions but also to mediastinal and hilar lymph nodes.
Similar to VBN, ENB requires high-quality CT scans in order to provide adequate resolution for the creation of a 3D map of the airways. In case of the Superdimension system, cuts of 1.5 mm or less and a 1.0 mm overlap are necessary. The Superdimension system is composed of four main components: a disposable working channel and guide catheter, a software which provides the physician with planning and navigation views of the lungs via merged CT images and hardware including computer, monitors, and an electromagnetic location board. The location board emits low-frequency electromagnetic waves during the procedure and is placed under the cranial end of the mattress on the bronchoscopy table.
After CT image registration by the ENB software, the operator can determine several targets to which navigation is desired. The software then suggests a pathway to the chosen target and provides a 3D film of the appropriate route. It can be adjusted as deemed necessary.
In the following registration phase the patient lies down on the location board and three sensors are placed on the body, serving as markers for the system.
Subsequently, electromagnetic guidance is achieved through a sensor incorporated in the distal tip of a steerable navigation catheter. It can be tracked by means of the electromagnetic field created by the locator board and matched to the information contained in the CT scan analyzed in the planning phase. Together with the extended working channel the navigation catheter is inserted into the bronchoscopes working channel. Once the bronchoscope cannot proceed any more, the steerable guide catheter is advanced by turning and pulling the control lever on the catheter’s handle. The first version of the catheter was steered by four separate wires controlling the movement of the probe from the proximal end of the device thus allowing its distal section to be steered in a complete circle (360°). The location and steering directions are performed according to the sensors’ position on the CT roadmap leading to the target that was previously registered to the patient’s lung. When reaching an airway bifurcation, the catheter is steered by manipulating the handle. A triangle depicted on the handles dial indicates the direction of the catheters tip. By pulling the handle, the tip is brought into the desired position. After reaching the target, the extended working channel is kept into place whereas the navigation catheter is removed. The extended working channel now serves as an extension of the bronchoscopes channel similar to a guide sheath. Tissue sampling can now be performed. A recently introduced, updated version of the steerable guide catheter has a curved tip. Through this, it can be steered more easily by simply rotating the catheters handle (Figure 15.5).
Figure 15.5 Image of an electromagnetic guided bronchoscopy with the sensor in the lesion in different planes.
One study reported on the influence the adjustment of CT scan and patient has on diagnostic yield. In case of a discrepancy <4.4 mm, the diagnostic yield was 71 percent, whereas it dropped to 40 percent where discrepancies were >4.4 mm. While the size of lesions had no significant impact on diagnostic yield, sampling in the lower lobes showed a lower yield. This might be due to a higher navigation error occurring in more peripheral lesions. This also applies to lesions near the diaphragm caused by permanent movement within the respiratory cycle. These drawbacks might be overcome to some degree by the recently implemented automatic registration of the system.
A recent meta-analysis characterized ENB to be effective and safe. It showed an overall sensitivity of 71 percent in the detection of cancer by sampling 1033 nodules.
The major challenge to ENB depicts the high costs of the procedure. In case of the SuperDimension system, the single use locatable guide costs between US $700 and $1000, depending on the market.

Full access? Get Clinical Tree
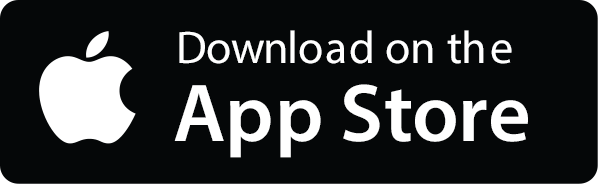
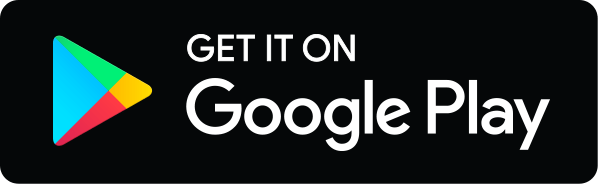