1 General Principles of Ultrasound-Guided Peripheral Nerve Blocks
1.1 Technical Requirements
1.1.1 Equipment
Portable, high-resolution ultrasound machines with interchangeable transducers now constitute devices that are well suited for use in anesthesiology and intensive medicine.
Types of Visualization
B-mode (brightness mode).
Every signal received from the transducer is displayed in a certain gray tone depending on its amplitude (intensity). The B-mode is used only in conjunction with the two-dimensional (2D) real-time mode. A two-dimensional image is made from the numerous ultrasound waves transmitted and received (Fig. 1.1). Depending on the penetration depth and the type of probe used, just a few images or up to 200 images per second can be visualized.

M-mode (or TM-mode, time–motion).
An impulse is transmitted with a high pulse repetition rate (1,000–5,000/s). The amplitudes of the signals received are displayed on the vertical axis (one dimensional) in various gray tones; the horizontal axis is the time axis on which the signals received over time are displayed at short intervals according to frequency.
Note
The M-mode has almost no role in ultrasound-guided regional anesthesia.
The M-mode can be helpful for diagnosing pneumothorax (see below). When the visceral pleura glide normally along the parietal pleura, a homogeneous granular pattern can be visualized below the pleura in M-mode—in contrast to the horizontal lines that can be seen above the pleura in immobile tissue (Fig. 1.2). If pneumothorax is present, the granular pattern below the pleura is replaced by horizontal lines (Fig. 1.3).



Transducers
Linear array and curved array transducers are used (Fig. 1.4).

Linear array transducer.
The standard transducer is the high-frequency linear array transducer. A multi-frequency transducer (e.g., 4.0–16 MHz), with the option of varying the frequency depending on the penetration depth required, is generally used. A special form of high-frequency linear probe known as a hockey stick linear array transducer is shaped so that it can be used in small anatomical situations. Modern multi-frequency linear broadband transducers use an electronic phased array technique, allowing the beam to be directed at various angles (sector scanning by electronic pan both in the transmission and the receptor field).
Curved array transducer.
The curved array transducer (2.0–6.0 MHz) is used for blocks of deeper nerves.
Practical Note
The higher the frequency, the better the resolution—but the lower the penetration depth.
1.1.2 Optimizing the Ultrasound Image
Newer generations of ultrasound machines have numerous options for optimizing the image, some of which are mentioned here; the designations sometimes vary depending on the manufacturer.
Penetration Depth
The penetration depth should be adjusted depending on the target structures. Increasing penetration depth leads to attenuation of amplitude and thus to poorer image quality. High frequencies are subject to strong attenuation and therefore do not penetrate tissue as well; low frequencies are attenuated to a lesser degree when penetrating tissue and are therefore better suited for visualizing deeper structures.
↑ Frequency = ↑ Signal attenuation
↑ Signal attenuation = ↓ Penetration
Practical Note
The higher the frequency, the higher the resolution! The highest possible frequency that still results in a usable image at the required penetration depth should always be selected. The target object should be approximately in mid-field when performing peripheral regional nerve blocks.
Frequency
The penetration depth is also relevant for the frequency.
Note
High frequency = high resolution, limited penetration depth. Low frequency = greater penetration depth, poorer resolution.
High frequency range (e.g., 8–12 MHz).
Optimal for penetration depths of 3 to 4 cm—for example, interscalene, supraclavicular, axillary, femoral, peripheral nerve blocks of the upper limb.
Medium frequency range (e.g., 6–10 MHz).
Optimal for penetration depths of 4 to 8 cm—for example, axillary, infraclavicular, femoral nerves in obese patients, distal sciatic nerve block.
Low frequency range (e.g., 2 – 5 MHz).
Optimal for penetration depths of > 8 cm—for example, proximal sciatic nerve blocks, psoas block.
Gain (Amplification)
The gain function amplifies the signals returning to the device. The amplification determines how bright (hyperechoic) or dark (hypoechoic) the image appears on the monitor. All signals received can be amplified equally over the entire field (overall gain). Too high overall gain leads to overexposure of the image and should be avoided.
Note
As a guide, the fluid in the vessels should appear black.
Using the “slide controls” available in most ultrasound machines (time gain compensation, TGC), the signals returning from various depths can be amplified to different degrees. This allows increased amplification of the attenuated signals received from deeper levels so a homogeneous image can be produced.
Modern ultrasound machines generally have a feature for automatic image optimization that produces the best possible balance between the overall gain and depth compensation settings. In particular, this feature makes it unnecessary to set the slide controls separately (they are all set at the same level when automatic image optimization is activated).
Focus.
The focus area should be set for the target structures, as the best image quality is achieved in the focus area. The number of foci can be varied.
Note
The more foci, the poorer the image quality.
Compound Imaging
Depending on the manufacturer, this technique is also known as “Cross Beam,” “M(ulti)View,” “SonoMB,” and “SonoCT.” Several two-dimensional images taken simultaneously from different angles (due to different beam angles, linear phased array, see above) are electronically compounded to one image for higher contrast resolution with sharper contours. Scatter echoes, speckle noise, and mirror artifacts are reduced.
Caution
In compound imaging, acoustic shadows are (more or less) suppressed as well as artifacts. The result may be that needles, catheters, or other structures that can be identified by the acoustic shadow they create become less visible.
Tissue Harmonic Imaging (THI)
Also called tissue enhancement imaging (TEI), this method is based on the fact that only those signals that return to the transducer with a frequency twice as great as the signals transmitted by the transducer (harmonic waves) are used for imaging. These high-frequency signals are generated by nonlinear propagation in tissue. This method allows improved contrast and spatial resolution (“lower noise” images). In particular, the artifacts that are caused by superficial fatty tissue are eliminated, as the harmonic waves are generated only with increasing penetration of the tissue (not usually used in peripheral regional nerve blocks).
Dynamic Range (Compression)
The dynamic range regulates the conversion of echo intensities to gray tones, for which the adjustable contrast range is enlarged. The dynamic range is useful for optimizing the texture of various anatomical tissues. The contours with the highest amplitudes should appear white, while the lowest amplitudes, for example blood, are still just visible.
Both in moving images, as film and video, and in still images, the dynamic range designates the ratios of the largest and smallest discernible brightness levels of noise and grain.
Many other functions are available from various manufacturers today. When the numerous functions are used optimally, an optimal image for peripheral regional anesthesia can usually be visualized.
Most machines have the option of saving the settings for various nerve blocks such as frequency, penetration depth, anticipated focus area, etc. (preset), which speeds up the procedure.
1.1.3 Structural Features in Ultrasound
Skin and Subcutaneous Fat Tissue
The skin (epidermis and dermis) is approximately 1 to 4 mm thick and can be readily differentiated as a narrow strip. Subcutaneous fat is hypoechoic with septa that are mainly parallel to the surface (Fig. 1.5).

A subcutaneous edema has a “riverbed-like” structure (Fig. 1.6).

Lymph nodes.
Lymph nodes have a hyperechoic center with a hypoechoic border. They can often be visualized in the inguinal region (Fig. 1.7).

Peripheral Nerves
Peripheral nerves consist of nerve fibers (axons) that are covered by a myelin sheath and are embedded in delicate layer of connective tissue, the endoneurium. Several nerve fibers are bundled together to form a fascicle surrounded by the perineurium. Several fascicles and connective tissue together form a peripheral nerve and are surrounded by the epineurium (Fig. 1.8).

Centrally located parts of the nerve fiber on the upper limb (nerve roots, trunks, and fascicle proximal to the clavicles) have a lower percentage of (hyperechoic) connective tissue than the infraclavicular nerve segments and therefore appear “monofascicular” or “oligofascicular” in the ultrasound image, that is, with a uniformly hypoechoic cross-section (dark round structures; Fig. 1.9, see also Chapter 3.2.3). Distal to the clavicles, the percentage of connective tissue increases in relation to the neurogenic part, leading to the characteristic “honeycomb” pattern in the cross-section of nerves located further peripheral in the upper limbs (Moayeri et al 2008). The fascicles (or rather several bundles of fascicles) appear as hypoechoic (dark), round structures within the hyperechoic connective tissue (Fig. 1.10).


The nerves in the lower limbs that can be visualized by ultrasound appear hyperechoic due to the relatively high percentage of connective tissue (Fig. 1.11; Moayeri et al 2010).

For novices, the median nerve in the forearm is the ideal object for visualizing this nerve structure in an ultrasound image (Fig. 1.12).

Tendons
It is not always easy to differentiate between tendons and nerves. Tendons do not have the typical “honeycomb” structure. Nerves can be best identified by following the course. Tendons have stronger anisotropy (see below) than nerves (Fig. 1.13).

Vessels
Vessels can be readily visualized in an ultrasound image and are often used as orientation. They are hypoechoic (i.e., dark) in the ultrasound image (Fig. 1.14).

Veins can be compressed by applying pressure.
Arteries pulsate (this is especially visible when the artery is compressed.
Nerves move in response to probe compression and do not pulsate (Fig. 1.14).
Doppler Methods
If there is any uncertainty in identifying vessels, the Doppler function can be used. One-dimensional methods (pulsed wave Doppler, continuous wave Doppler) are distinguished from two-dimensional, color-coded methods (color Doppler). The Doppler method is based on the fact that sound waves sent from a stationary transmitter change frequency when they meet a moving reflector. The velocity of the moving object (usually red blood cells) can be calculated from the change in frequency of the reflected wave compared with the transmitted wave, which is also detected by the transducer acting as receiver in order to determine blood flow velocity.
Pulsed Wave Doppler.
In the pulsed wave Doppler method (PW Doppler, “pulsed” Doppler), short intermittent ultrasonic pulses are transmitted and then received. A transducer functions as both transmitter and receiver. This allows the velocity to be measured at a selected location in a conventional ultrasound (B-mode), that is, a point in the conventional ultrasound can be determined where the velocity is to be measured. This is usually visualized as a curve in a velocity–time diagram (Fig. 1.16).
Continuous Wave Doppler.
In the continuous wave Doppler method (CW Doppler), a transmitter and a receiver in the transducer operate simultaneously and continuously, that is, signals are constantly transmitted and received simultaneously. The disadvantage of this method is that the depth of the Doppler echo cannot be determined. However, unlike PW Doppler, relatively high velocities can be registered. As in PW Doppler, visualization is in the form of a time–velocity diagram. This method is not generally used for regional anesthesia.
Color Doppler.
In the color Doppler method, the areas where movement is registered are visualized in a two-dimensional area (a window to be specified in a B-mode image) via Doppler analyses pulsed to thousands of individual points. Conventionally, movements along the beam axis toward the transducer are displayed as red and movements in the opposite direction (away from the transducer) are displayed as blue (BART: “blue away, red toward,” Fig. 1.15).


Practical Note
The visualization of flow movements is worst when the transducer is held perpendicular (90°) to the flow movement (e.g., to the cross-section of the vessel). The sound waves should thus always be directed at an oblique angle to the flow movement. To optimize the image, color gain must be at an optimal setting. Over-regulation results in the color appearing not only in the vessel, but in the entire color window! The pulse repetition frequency (PRF) must be adjusted to the anticipated flow velocity in the vessel. Arteries can be distinguished from veins based on color behavior and rhythmic behavior (artery) or more even movement (vein) of the signals received.

Full access? Get Clinical Tree
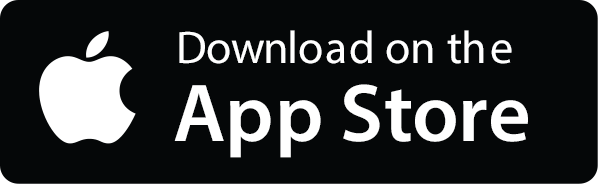
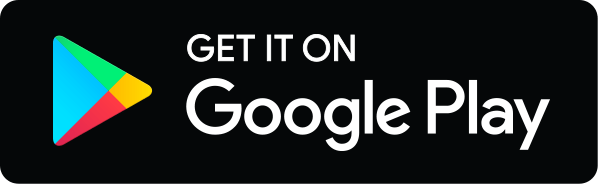
